Fig. 2.1
Tomographic ultrasound image (TUI) of multiple follicles in a stimulated ovary
3.
The static volume contrast imaging (VCI) mode: This mode allows for the receipt of information from adjacent slices in a volume. This imaging mode was especially developed to enhance the contrast between tissues or organs that would appear similar on conventional 2D US [18]. VCI is currently applied for detecting thoracic abnormalities [18] or imaging fetal pelvic anatomy [19]; it was found to be superior to 2D US in these studies.
4.
Inversion mode: In inversion mode, volumes are displayed in their entirety as an echogenic area, while the grayscale portions of the image are rendered as transparent. Some IVF and obstetrical applications could benefit from this method [20].
5.
Transparency mode: The transparency mode (also known as maximum mode) images regions with high echo density in a glass-like mode. This mode is mostly used for imaging of cartilaginous structures. With this mode, high-echogenic voxels are higher valued.
6.
7.
Glass-body rendering: Glass-body rendering (GBR) imaging is a combination of the transparency and color or power Doppler mode. It is very suitable for gynecologic applications; however, it is most useful for vessel imaging. In this mode the rendering algorithm is based on the simultaneous representation of gray and color Doppler scale [23].
8.
Four-dimensional image techniques: The four-dimensional image techniques (such as the spatiotemporal image correlation (STIC)) allow for procedures such as echocardiography of the fetal heart [24, 25]. The received data is acquired by a single, automatic volume sweep; subsequently, the software analyzes the data according to their spatial and temporal domain and processes a 4D cine sequence. Prior to the launch of these 4D techniques, the examination of the fetal heart with conventional US was often difficult.
9.
OmniView: OmniView (GE Medical Systems, Kretztechnik, Zipf, Austria) is a recently developed display technology for 3D and 4D US that allows integration of volume datasets and the simultaneous display of up to three independent (non-orthogonal) planes by manually drawing straight or curved lines from any direction or angle [26]. Although many 3D US display techniques have been employed (more than the ones described in this chapter), the two most commonly used are multi-planar reformatting and volume contrast rendering. Image quality has improved since the 1990s; nevertheless, the final quality still depends on the accuracy of the scanned volumes (also see next paragraph). Nevertheless, as previously mentioned, various software-based tools are currently available. Post-processing is done via the electronic scalpel (Kretztechnik, Zipf, Austria), contrast and brightness regulation, or speckle reduction imaging (SRI). SRI was established on the Voluson platform in 2004 by GE Healthcare. The electronic scalpel allows for the removal of pre-located obscuring structures in three steps: (1) rotation of the rendered image into a position where the obscuring structures can be cut; (2) the selection of the cutting mode; and (3) creation of the outline for the cut and activation of the cutting mode [27]. Speckles are recurrent problems in sonography due to interference of ultrasound echoes; they produce difficulties in differentiating anatomical structure. Many approaches have been attempted with the goal of reducing or even of eliminating speckles. Most significantly, the increased computer processing power and speed of calculation within recent years have allowed more complex image-processing techniques to reduce these artifacts without impairing image quality [28]. In addition, filtering is widely used for undesired echoes that are a recurrent phenomenon in sonography. Filtering allows the suppression of unwanted background noise or the enhancement of the desired information (suppressing and enhancing filters) [29].
Initially, data storage was a problem with 3D technology. This problem has been resolved by the improved storage capacity of the hard disk. The acquired data can be readily stored without quality loss either at the US systems itself or on external media (CD, DVD, USB, or external hard drive). The data can still be post-processed with medical software and can be transferred, for example, via (virtual private network) VPN tunneling to another physician. Most importantly, the data can be reloaded and subjected to an additional expert opinion or used for education [30]. This is an invaluable feature. Currently, this capability facilitates optimal patient care. Moreover, it might reduce medical malpractice and might prove to be an essential step towards standardization in this field.
Advantages and Shortcomings of 3D US Techniques
Three-dimensional US allows visualization of all three image planes (sagittal, transverse, and coronal) of the analyzed object, including planes not accessible by 2D US. The viewed planes can be chosen or changed by the user to view the desired region under investigation. Using the conventional 2D technique, the referring physician often had to integrate all the received 2D images in a three-dimensional form to obtain an impression about the anatomy and possible pathologies.
The use of 2D ultrasound for measurement of organs, such as the uterus or follicle volume, is variable and at times inaccurate. Some diagnostic and treatment IVF procedures require accurate volume measurements (such as follicle monitoring). The accurate acquisition of volumes is only possible with 3D US, especially when the analyzed structures are not spherical but complex (i.e., follicles within a multi-follicular growth complex; this problem will be discussed in a subsequent chapter). 3D US is a relatively new technique for gynecology and IVF. However, studies have already been published reporting high accuracy.
Use of the 3D technique together with the appropriate software allows for the accessed 3D volumes to be digitally stored. This is a tremendous advantage for later post-processing and evaluation. Thus, the stored data can be reformatted and analyzed in various ways. Beyond all the technical improvements, 3D US is still based on conventionally received 2D US data. Thus, poor US scanning techniques cannot be improved by this innovative technique. Inadequate resolution of 2D scans results in poor image quality of the three-dimensional structures. Therefore the 2D scans must be optimized in regard to (1) brightness, (2) the depth of field, and (3) the correct positioning of focus. The correct setting to achieve the optimal 2D scan is therefore a prerequisite for the best possible 3D calculation.
In addition, most artifacts, which are common in 3D or 4D US, are also similar to those of 2D US because both techniques are based on the same principle. Improper calibration and operation is one major source of the production of inferior data as well as for poor interobserver reliability. This holds true for both, 2D and 3D US. One exception might be the appearance of artifacts due to the movement of the examined objects with 4D US. However, the application of 4D US does not play a dominant role in ART because most of the analyzed structures are immotile. However, it is not unequivocally clear whether the 4D technique might be of future value for ART. For example, endometrial blood flow analyzed by 4D US might become an additional parameter for the prediction of embryo implantation in the future.
The 3D visualization technique offers no additional advantages in regard of reducing the aforementioned artifacts. The effective improvements of US imaging is due to accompanying post-processing software. However, this technical processing cannot be regarded separately as these are integrated components of high-quality 3D instruments.
The rapidly increasing processing power was also one basic requirement for the introduction of portable US systems. This innovation, together with standardized higher image quality, standardized image storage, and compression techniques such as the Digital Imaging and Communications in Medicine (DICOM) standard, enables a medical network and improved patient care.
Applications of 3D Ultrasound in ART
Several clinical trials have demonstrated the superiority of 3D systems compared to conventional 2D US. The images of the structure’s surfaces become fully apparent (as a result of their three-dimensional nature). The detailed advantages of the 3D sonography, especially for IVF, are discussed within the Chap. 20. However, studies concerning 3D US in the context of IVF therapies are still limited. Conversely, 3D US in monitoring is commonly used during pregnancy and a crucial tool in prenatal diagnosis because the volumes of fetal organs can be accessed more accurately by the new 3D techniques rather than by conventional 2D US. Thus, fetal anomalies can be recognized more readily [31, 32].
The applications of 3D US in IVF are also summarized in the Chap. 20. However, an overview of the applications of 3D sonography will be presented here. The investigation of the uterus, ovaries, and fallopian tubes are a prerequisite of an IVF cycle. Uterine pathology such as myomas, malformations (see Figs. 2.2 and 2.3) and carcinomas can significantly impair the outcome of IVF therapy [33], and sometimes patient health. In addition to MRI and CT, 2D US was and is currently used for the detection of congenital malformations of the female reproductive system. However, it lacks specificity and image quality [34–37]. Three-dimensional sonography allows a more accurate calculation of the uterine volume. It also allows a more precise estimation of endometrial morphology and volume. Endometrial volume and morphology are the most crucial factors for IVF success. Nonoptimal endometrial lining or a small endometrial volume impacts embryo implantation. In these cases, it might be prudent to consider embryo cryopreservation and opt for a cryocycle [38]. In addition, 3D techniques such as 3D Doppler enable the visualization of endometrial blood flow and neovascularization of cervical carcinomas, which are completely beyond the scope of conventional 2D US.
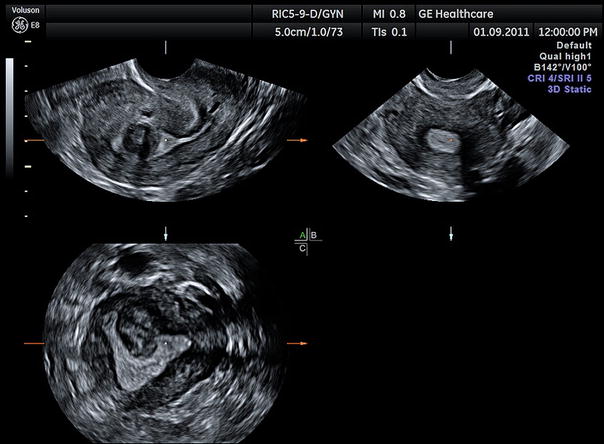
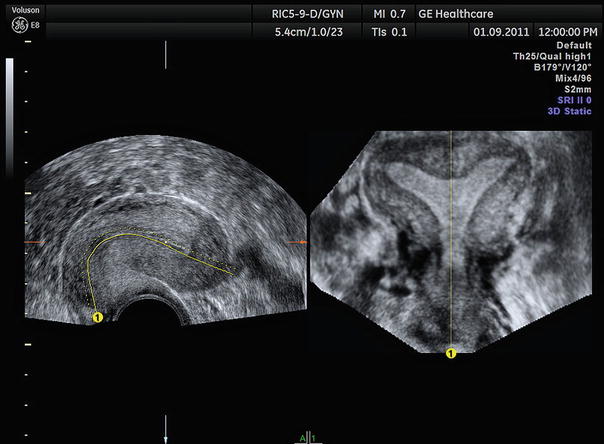
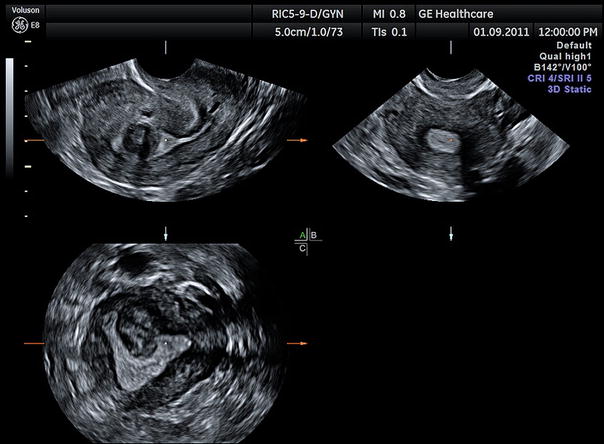
Fig. 2.2
3D image of a uterine myoma (Voluson E8)
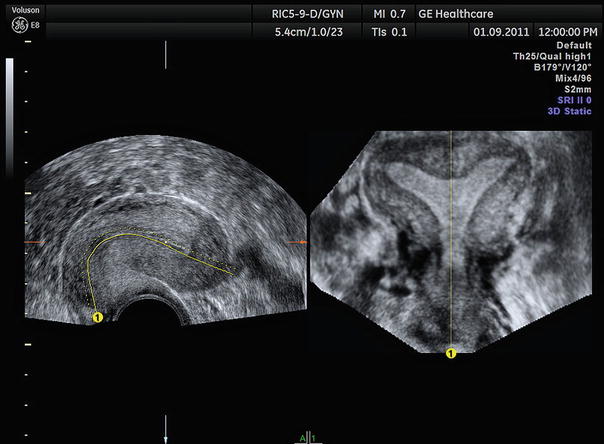
Fig. 2.3
3D image of uterus arcuatus (Voluson E8)
Imaging modes such as the glass-body mode are the most optimal for the recognition of tumor-feeding vessels. The glass-body mode is a very good method for hysterosalpingo-contrast-sonography (HyCoSy) to detect abnormal varicosity in the adnexal region. The use of 3D US dramatically facilitates HyCoSy and provides a better assessment of tubal patency [39]. Furthermore, 3D US techniques enable an estimation of the ovarian reserve and can be applied during follicle monitoring [40]. Thus, particular automated systems such as the SonoAVC (GE Medical Systems, Kretztechnik, Zipf, Austria) are user-friendly systems, which can accurately determine follicle number and size.
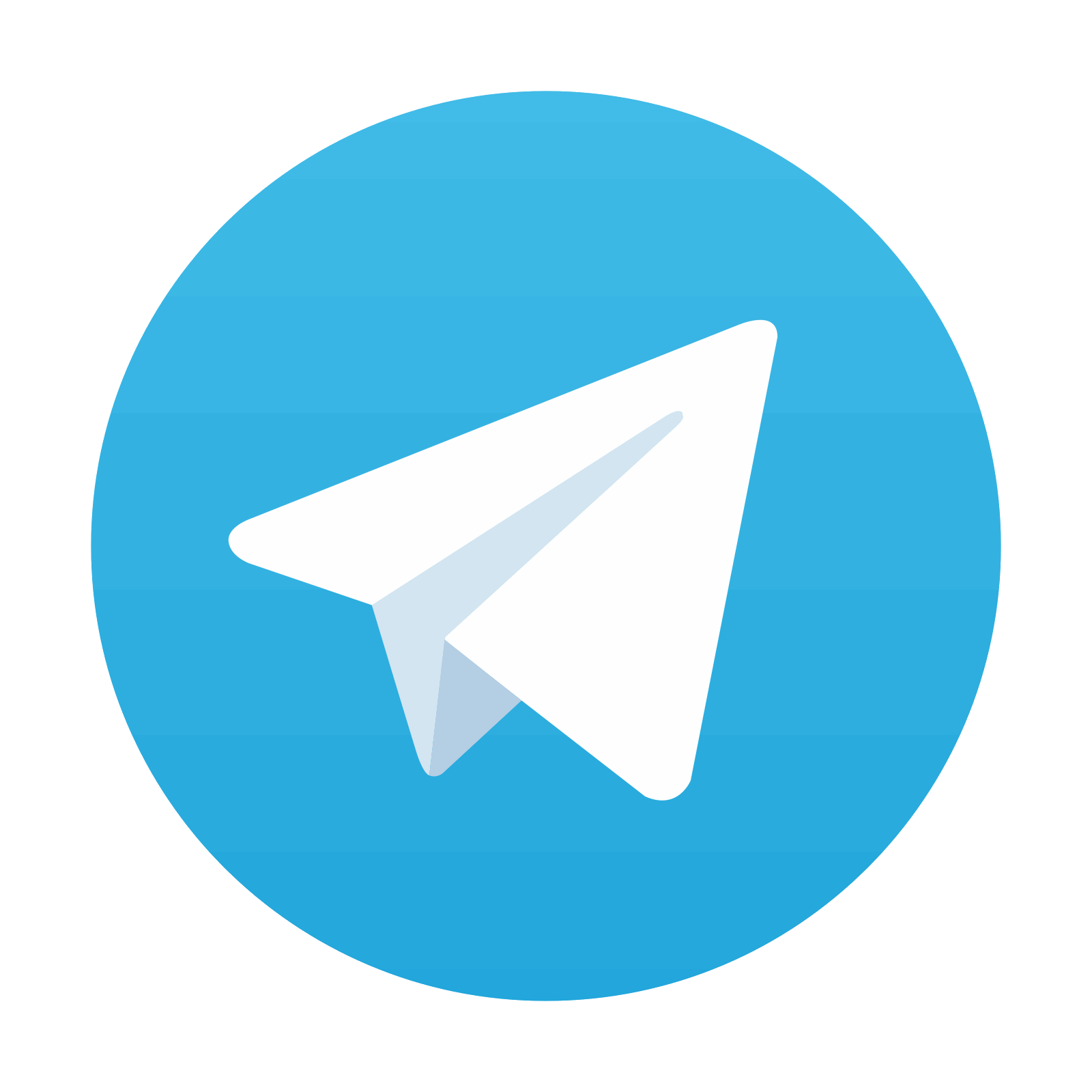
Stay updated, free articles. Join our Telegram channel

Full access? Get Clinical Tree
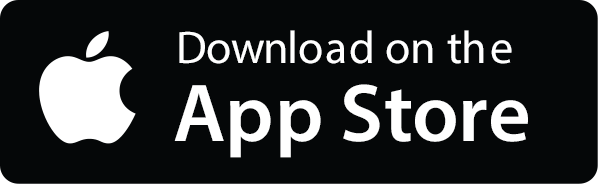
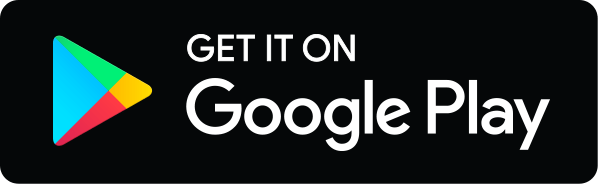