3 This section refers mainly to the Suggested protocol heading considered for each examination in Part 2, although pulse sequences are sometimes mentioned under the Technical issues subheading of Image optimization. A summary of the mechanisms and uses of the most commonly used pulse sequences are described. All pulse sequences are described using their generic name. Table 3.1 provides a comparison of the acronyms used by the main manufacturers to describe their pulse sequences and imaging options. The parameters given in Table 2.1 should be universally acceptable on most systems with field strengths of 1.5 T and 3 T. However, weighting parameters in particular are field strength dependent, and therefore, some modification may be required if you are operating at extremely low or high field strengths. Only a brief overview is provided here. For a more detailed explanation, please refer to Chapters 2 and 5 of MRI in Practice or an equivalent text. Table 3.1 Comparison of manufacturer acronyms (see How to use this book for abbreviations) An SE pulse sequence (also known as conventional spin echo (CSE)) usually uses a 90° excitation pulse followed by a 180° rephasing pulse to produce an SE. Some SE sequences use a variable flip angle, but traditionally the excitation pulse has a magnitude of 90°. This amplitude of the flip angle is consistently assumed in the protocols. SE sequences can be used to generate one or several SE. One echo is usually used for T1 weighting while two echoes are used for proton density (PD) and T2 weighting. SE pulse sequences are the most commonly implemented sequences as they produce optimum SNR and CNR. Fast spin echo (FSE) uses a 90° flip angle followed by several 180° rephasing pulses to produce several SE in a given TR. Each echo is phase encoded with a different amplitude of gradient slope, so that data from each echo are collected and stored in a different line of k-space. In this way, more than one line of k-space is filled per TR, and the scan time is reduced accordingly. The echo train length (ETL) (also known as the turbo factor) refers to the number of 180° rephasing pulses and therefore echoes that correspond to the number of lines of k-space filled per TR. The longer the ETL, the shorter the scan time as more lines of k-space are filled per TR. FSE can be used to produce either one or two echoes as in SE. The echo train may be split so that data are collected from the first half of the echo train to acquire the first echo, and from the latter half to acquire the second echo. This strategy is commonly used to produce PD and T2 images that demonstrate similar weighting to SE. However, T2 images can be acquired without a PD image. A T2 image alone, rather than a dual echo, is often acquired in Part 2. It is of course perfectly justified to use a dual echo sequence if this is required. For more information, see Technical Issues in Brain in Part 2. FSE sequences have been further modified to include 3D acquisitions and single-shot techniques. Single-shot FSE (SS-FSE), which is also termed HASTE (half acquisition single-shot turbo spin echo), combines long ETLs that fill all of k-space in one shot with half-Fourier acquisition techniques that acquire only half of k-space and then transpose data into the other half. This technique allows very rapid acquisitions, which enables multiple-slice breath-hold and real-time imaging. Some contrast characteristics of FSE differ from conventional SE. Fat remains bright on T2-weighted images, and fat suppression techniques may be needed to compensate for this. The multiple 180° RF pulses used in FSE sequences cause lengthening of the T2 decay time of fat so that the signal intensity of fat on T2-weighted FSE images is higher than in SE. This sometimes makes the detection of marrow abnormalities difficult. Therefore, when imaging the vertebral bodies for metastatic disease, a short tau inversion recovery (STIR) sequence should be utilized. Muscle can appear darker than usual especially on the T2-weighted images. This is again due to the multiple 180° pulses causing a MT effect. In addition, certain artefacts may be prominent in FSE sequences. Image blurring is often a problem in long ETL sequences. This occurs because each line of k-space contains data from echoes with a different TE. In long ETL sequences, the very late echoes have a low signal amplitude and, as the outer lines of k-space are filled with data from these echoes, there are insufficient data to provide adequate resolution. Image blurring is most commonly seen at the edges of tissues with different T2 decay times. It may be reduced by decreasing the size of the FOV in the phase direction (depending on how the manufacturer implements a rapid FSE sequence) or by selecting a broad receive bandwidth. However, while the latter does improve overall image quality by reducing blurring, it also reduces the SNR. Lastly, FSE is not always compatible with options such as phase-reordered RC, and therefore, conventional SE or breath-hold sequences are often the sequence of choice when respiratory artefact is likely to be troublesome. IR pulse sequences begin with a 180° pulse that inverts the net magnetization vector into full saturation. When the inverting pulse is removed, the magnetization begins to recover and return towards B0. After a specific time TI (inversion time), a 90° excitation pulse is applied which transfers the proportion of magnetization that has recovered to B0 into the transverse plane. This transverse magnetization is then rephased by a 180° rephasing pulse to produce an echo. In IR-FSE, several 180° rephasing pulses are applied as in FSE, so that more than one line of k-space can be filled per TR, so reducing the scan times. Conventional IR is most commonly used to produce heavily T1-weighted images. However, it and IR-FSE may also be implemented to eliminate the signal from certain tissues by applying the 90° excitation pulse when the magnetization in that tissue has recovered into the transverse plane and therefore has no longitudinal component. In this way, signal from tissue is nulled by the excitation pulse. There are two main uses of this technique. STIR uses a short TI that corresponds to the null point of fat so that the excitation pulse specifically nulls the signal from fat. In Part 2, STIR is used as a fat suppression technique in conjunction with an FSE sequence to produce T2 weighting by using long TEs and ETLs. FLAIR utilizes a long TI corresponding to the null point of cerebrospinal fluid (CSF) so that the excitation pulse specifically nulls the signal from CSF. Again, long TEs and ETLs that enhance T2 weighting are commonly used to enhance the signal from pathology especially periventricular lesions. In all IR sequences, the TI is field strength dependent. In FLAIR sequences combined with long ETL FSE, if the TR is not long enough to allow full recovery of z magnetization after the last echo in the train has been collected, a shorter TI than usual may be required to null the CSF signal adequately. This is because if only partial z magnetization has recovered at the end of the TR period, this is converted into only partial –z magnetization after inversion, and therefore, the magnetization in CSF does not take long to reach its null point.
Pulse sequences
Introduction
Pulse sequence/imaging option
General Electric
Philips
Siemens
SE
SE
SE
SE
FSE
FSE
TSE
TSE
Coherent GRE
GRASS
FFE
FISP
BGRE
FIESTA
BFFE
True FISP
Incoherent GRE
SPGR
T1 FFE
FLASH
Steady-state free precession (SSFP)
SSFP
T2 FFE
PSIF
IR
IR
IR
IR
STIR
STIR
STIR
STIR
Fluid-attenuated inversion recovery (FLAIR)
FLAIR
FLAIR
FLAIR
Pre-saturation
SAT
REST
SAT
Gradient moment nulling
FC
FC
GMR
RC
RC
PEAR
RC
Signal averaging
NEX
NSA
AC
Partial averaging
Fractional NEX
Half scan
Half Fourier
Oversampling
No phase wrap
Fold over suppression
Oversampling
Rectangular/asymmetric
Rectangular
Rectangular
Under-sampling
FOV
FOV
FOV
FOV
Spin echo
Fast spin echo or turbo spin echo
Inversion recovery (IR/IR-FSE)
Coherent gradient echo (T2*)
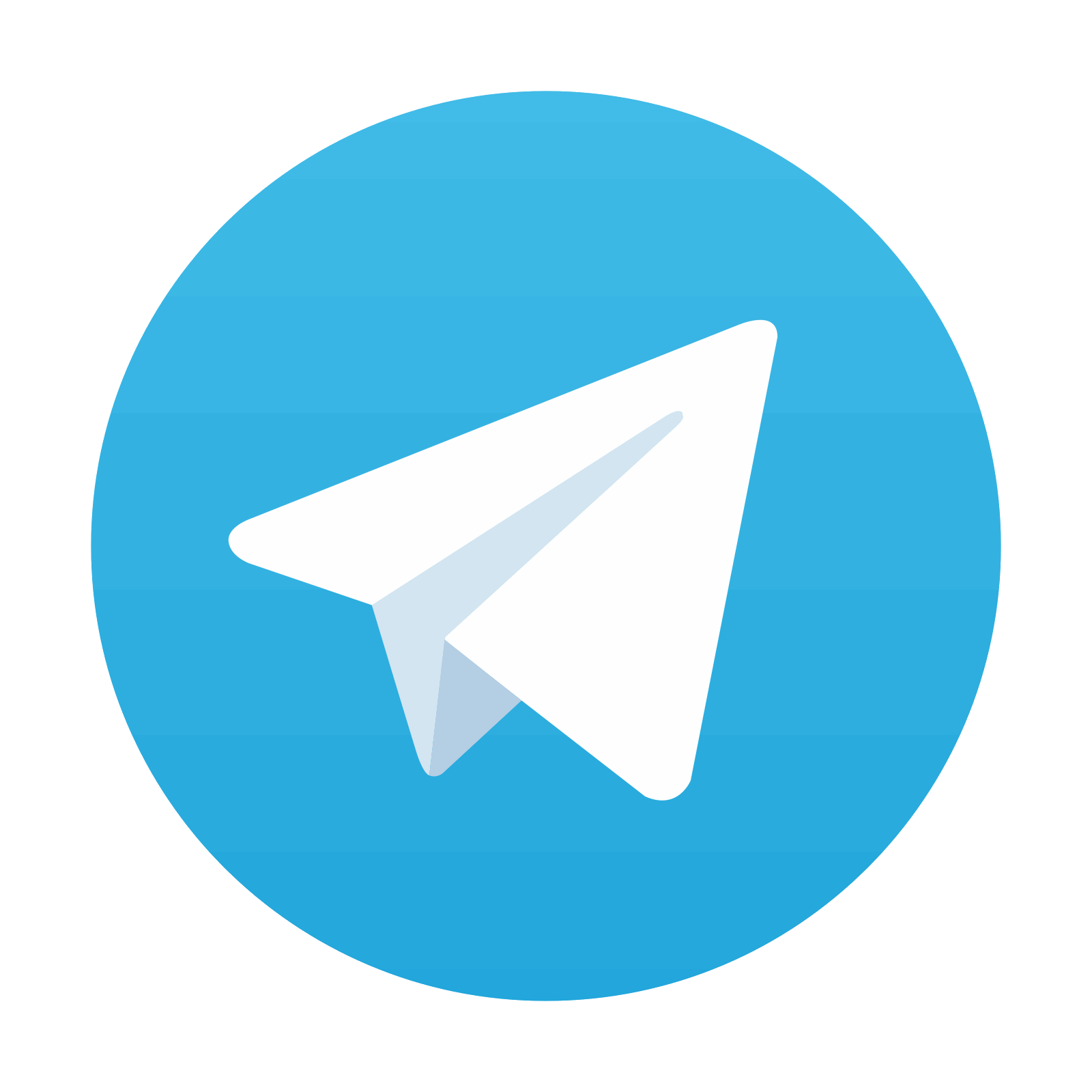
Stay updated, free articles. Join our Telegram channel

Full access? Get Clinical Tree
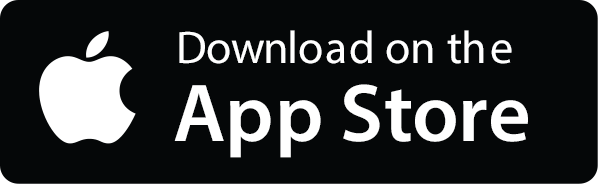
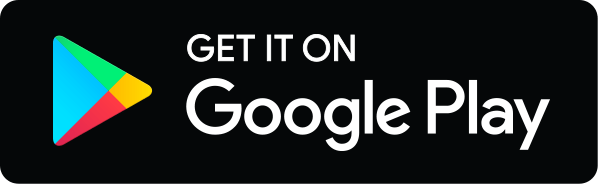