Fig. 1
Size parameter in E. coli analysis by imaging flow cytometry. Examples of rods, roundish and intermediate-sized bacteria on images (panels) and on dotblot (area vs. aspect ratio intensity)
However, IFC has a number of limitations: (1) it does not allow interrogation of individual cells repeatedly creating time-lapse series; (2) the amount of collected light is limited, because of the short time in which cell pass the flow cell (typically milliseconds). Though comparing with a conventional cytometry the amount of collected light is larger due to lower flow rate and time delay approach (collecting light over longer interval of time) using custom-modified charge-coupled device (CCD) camera for capturing of images; (3) because of the lower flow rate the number of events collected per unit of time is lower than in conventional flow cytometry. Besides necessity of image analysis of each cell makes generation of large data sets inconvenient.
6 Examples of IFC Applications
Recent studies show that IFC allows to localize and quantitate molecules (peptides, proteins, protein complexes, nucleic acids (DNA, RNA, miRNA), glycolipids, molecular assemblages, etc.) not only in the single cells, but also in multicellular clusters and small aggregates and to map their spatial distribution to the cellular compartments. Single cell measurements allow revealing information hidden in population averages.
Using fluorescent molecules as markers allows IFC to perform analysis of host-pathogen interaction, quantification of intracellular parasites and has a potential of providing high-throughput analysis of host-protein interactions and assessment of drugs for parasitic and microbial infections [16, 17].
IFC is extremely helpful in the cases when live cells could be associated with dead cells and apoptotic bodies [3], adherent platelets/platelet fragments [4], or extracellular vesicles (EVs). In these situations use of conventional flow cytometric techniques and excluding events only by FSC/SSC parameters may result in the interpretation of negatively stained cells as positive ones. This was shown for apoptotic markers [18] and/or the CD41a+ expression [19]. CD41a is a glycoprotein (Ca++-dependent heterodimer) that serves as a receptor for adhesion proteins on the surface of platelets and mediate cell-to-substrate and cell-to-cell adhesion. Many cancer cells floating in the blood vessels are temporarily coated with platelets, possible to escape immune surveillance [19, 20] and their analysis by IFC is the most promising approach.
IFC is also very helpful in distinguishing false-positive events in cell death evaluation [21] and during analysis of activated cells. The cellular suspensions always have level of spontaneous apoptosis >0 % and constantly produce extracellular vesicles (EVs) in response to apoptotic and activation stimuli. The EVs are lipid vesicles produced by all cells, and can carry nucleic acids and a number of proteins originated from cell-progenitors. Therefore EVs can be positively stained with DNA and lipid dyes, and/or fluorochrome-conjugated antibodies and attach to negatively stained cells. Annexin V+ staining is a marker of significant subset of EVs (microparticles, size range 100–1000 nm). Only IFC allows verification and exclusion of a subset of cells with EVs attached to their surface and obtaining proper statistics on the cell populations.
7 Conclusions
While IFC boasts over a 30-year history, its development within last decade (Imagestream-based IFC with IDEAS software including the advantages highlighted in this review) is indeed exploding. The applications of IFC are extensive and include but not limited to immunophenotypic multiparametric analysis combined with morphometric assessment of cells. An interesting road lies ahead when the automated search of morphological differences between cellular structures will become easy available and useful tool for future research and drug discovery.
Acknowledgements
The work was supported in part by NIH S10 RR023459 grant, Harvard Pilot grant, and the Russian Foundation for Basic Research grants 13-04-40189-H and 11-04-01749a. We are also grateful to Aleksandra Gorelova (Harvard University) for help with the editing and preparation of the manuscript.
References
1.
Oheim M (2011) Advances and challenges in high-throughput microscopy for live-cell subcellular imaging. Expert Opin Drug Discov 6:1299–1315. doi:10.1517/17460441.2011.637105 CrossRefPubMed
2.
3.
Henery S, George T, Hall B, Basiji D, Ortyn W, Morrisey P (2008) Quantitative image based apoptotic index measurement using multispectral imaging flow cytometry: a comparison with standard photometric methods. Apoptosis 13:1054–1063. doi:10.1007/s10495-008-0227-4 CrossRefPubMed
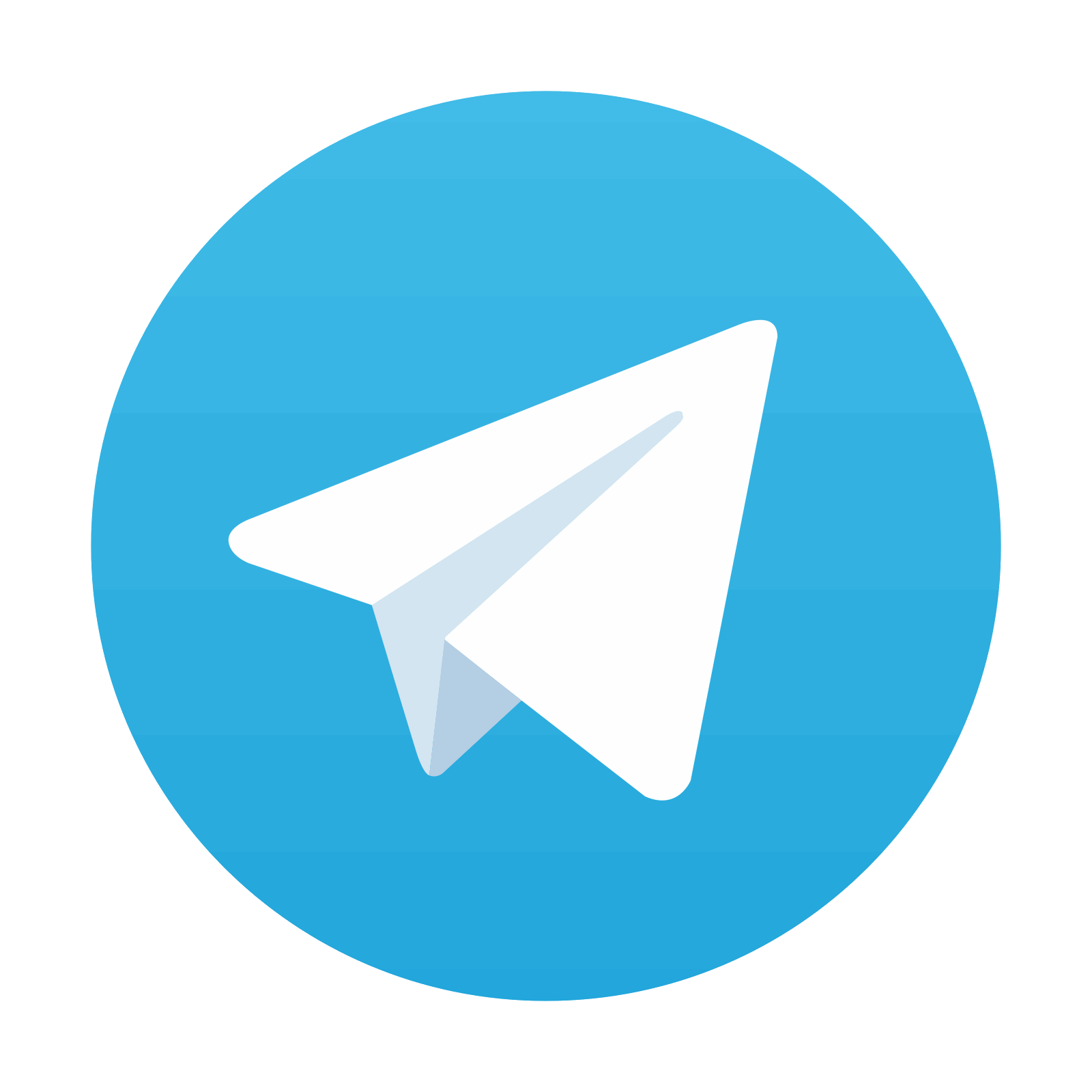
Stay updated, free articles. Join our Telegram channel

Full access? Get Clinical Tree
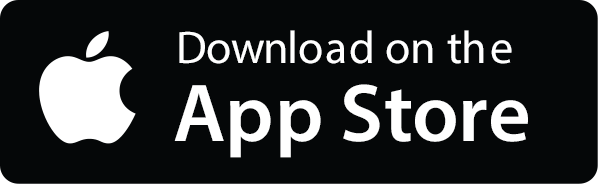
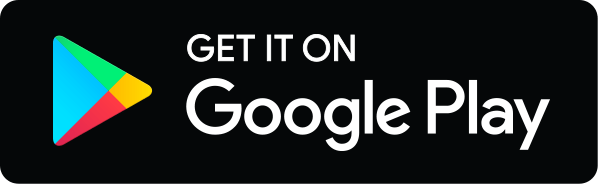