Fig. 15.1
Structure of a typical mammalian cell.
The nucleus contains chromosomes , which have a threadlike structure of two arms connected by a centromere (Fig. 15.2). Chromosomes are formed of genes, which are the basic units of heredity in the cells of all living species . Genes are composed of deoxyribonucleic acid (DNA) molecules. The structural relationship of DNA molecules, genes, and chromosomes is shown in Fig. 15.2. The sequence of genes in the chromosome characterizes a specific chromosome. Two categories of cells—namely, germ cells (reproductive cells such as oocytes and spermatozoa) and somatic cells (all other cells)—are based on the number of chromosomes they contain. Whereas germ cells contain n number of individual chromosomes, somatic cells contain 2n number of chromosomes in pairs, where n varies with species of the animal. In humans, n is equal to 23; therefore, there are 23 chromosomes in germ cells and 46 chromosomes in somatic cells.
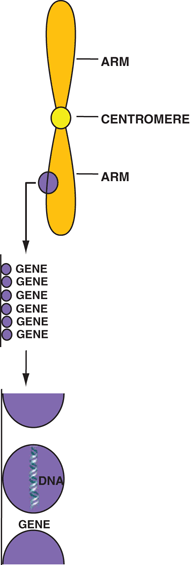
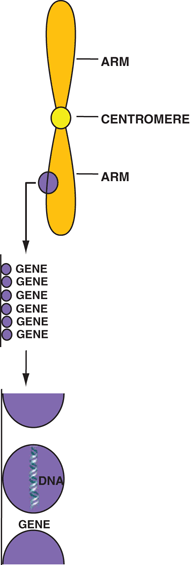
Fig. 15.2
Structural relationship of chromosomes, genes, and DNA molecules.
In the cytoplasm of the cell exist four important organelles—ribosomes, endoplasmic reticula, mitochondria, and lysosomes—that carry out the cellular metabolic activities. Ribosomes are made up of protein and ribonucleic acid (RNA) and are responsible for protein synthesis in living matter. Endoplasmic reticula are tubular structures mostly responsible for protein synthesis. Mitochondria are ellipsoidal structures with a central cavity and contain specific enzymes to oxidize carbohydrate and lipid to produce energy. Lysosomes are small organelles in the cytoplasm that contain enzymes capable of lysing many nutrients and cells.
The entire cytoplasm is enclosed within a cell membrane made of lipids and proteins. Its primary function is to selectively prohibit or permit the passage of substances into and out of the cell.
The growth of living matter is caused by proliferation of cells by cell division—a process in which a cell divides into two cells. The cell division of somatic cells is called mitosis and that of germ cells is called meiosis . Both mitosis and meiosis, designated as M, consist of four phases: prophase, metaphase, anaphase, and telophase. Each of these phases involves the rearrangement of the number of chromosomes and represents the progression of cell division (Fig. 15.3) and is described below.
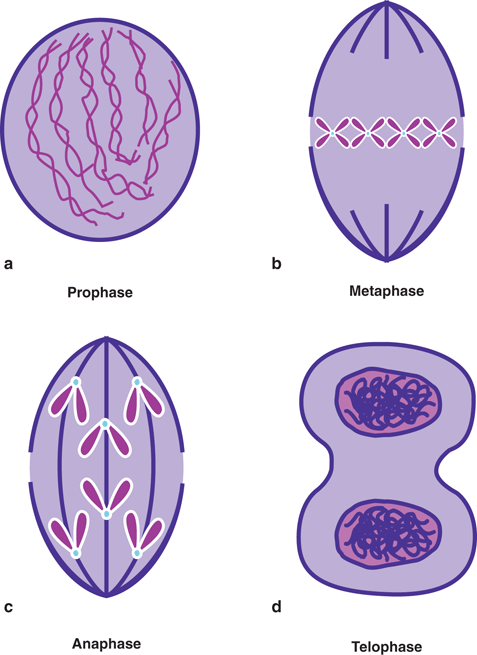
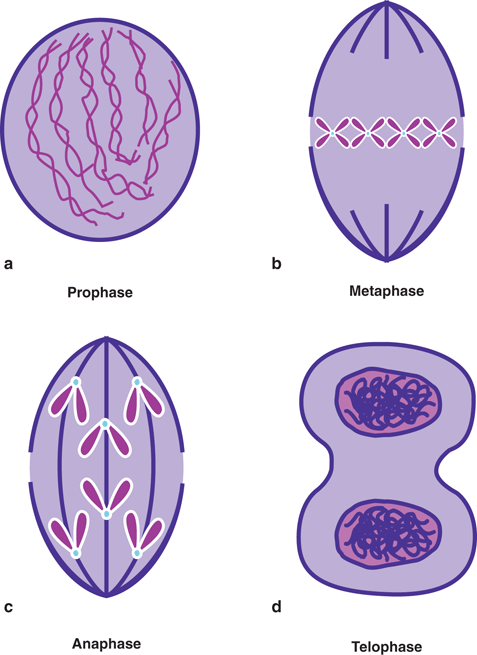
Fig. 15.3
Different phases of mitosis. See text for details.
In prophase, the chromosome thickens in the shape of a dumbbell with a constriction at the center, called centromere. The nuclear membrane breaks open, leading to the mixture of cytoplasm and nuclear material, and spindles made of fibers are formed extending from one end (pole) of the cell to the other. Next in the metaphase, the chromosomes move to and line up at the central (or equatorial) plane of the cell, and the centromeres divide into two, each attaching to the spindle. Anaphase then follows and two chromatids move to the two poles of the cell. The last step of cell division (telophase) involves the deconvolution of the chromosomes leading to the regeneration of the nuclear membrane and nucleoli around both poles. Division of cytoplasm (cytokinesis) sets in, and ultimately two daughter cells are formed.
Before cell division, each cell undergoes a long period, termed interphase, in which DNA molecules are synthesized. In DNA synthesis , two new DNA molecules are produced from each DNA molecule, which are exact replicas of the original DNA molecule. This period of DNA synthesis is designated the “S” phase, which takes place around the middle of the interphase. The period between the telophase and the S phase is termed G1, and the period between the S phase and the prophase is termed G2 (Fig. 15.4). During the G1 and G2 periods, no functional activity related to cell division occurs. The period of the entire cell cycle including the M and S phases varies with the types of cells. The S phase normally is the longest and G1 is the most variable phase in the cell cycle. The duplicate DNA molecules lead to two identical chromosomes during mitosis, which are termed sister chromatids.
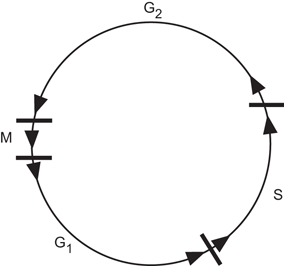
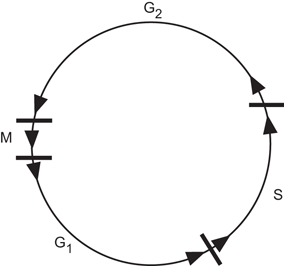
Fig. 15.4
The cell cycle. S is the DNA synthesis phase. M is the period of mitosis during which the prophase, metaphase, anaphase, and telophase take place. G 1 is the period between the telophase and S, and G 2 is the period between S and the prophase.
One important difference between mitosis and meiosis is that in meiosis, for a given series of cell division, every alternate cell division skips DNA synthesis, thus keeping the number of chromosomes the same in germ cells.
Effects of Radiation
DNA Molecule
The nucleus of the cell is the most sensitive part to radiation and this sensitivity has been attributed to the DNA molecule . To understand the effect of radiation on the DNA molecule, a knowledge of its structure is essential. It has a double-helical structure consisting of two strands, which are like the two rails of a ladder (Fig. 15.5a). The strands are composed of sugars interlinked by phosphate bonds. The two strands are connected to each other by rungs made of four bases: thymine (T), adenine (A), guanine (G), and cytosine (C) (Fig. 15.5b). The bases are bonded to the sugar molecule on the strands on both sides, and are paired to each other by hydrogen bonds. These four bases are arranged in a very specific manner to form a specific gene in every living species and provide the unique characteristics to these species.
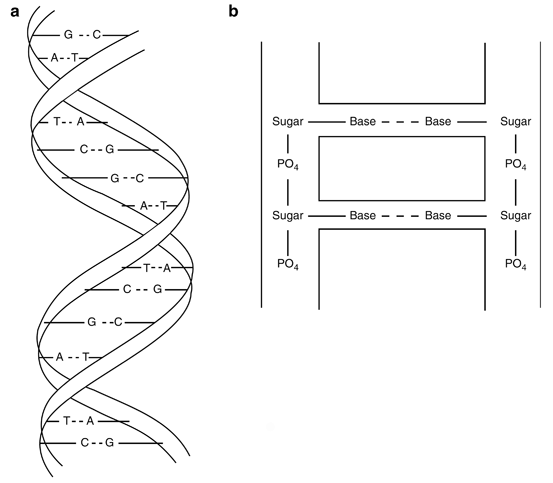
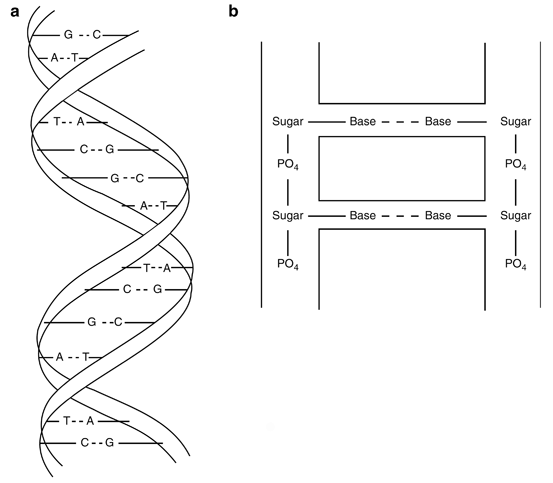
Fig. 15.5
a Double-helical structure of DNA molecule composed of four bases: adenine (A), guanine (G), thymine (T), and cytosine (C). b Configuration of a DNA molecule: strands are formed by sugar molecules bonded by phosphate groups. The rungs of the ladderlike structure are formed by bases connected to each other by the hydrogen band (dashed line) and to the sugar molecule on the strands on both sides.
Radiation damage to the DNA molecule can be due to
(a)
Loss of a base
(b)
Cleavage of the hydrogen bond between bases
(c)
Breakage of one strand of the DNA molecule (single strand)
(d)
Breakage of both strands of the DNA molecule (double strand)
These radiation effects on DNA molecules are illustrated in Fig. 15.6. These changes result in so-called mutations , which have adverse effects on the genetic codes . The number of mutations increases with increasing radiation exposure. At low-dose exposures, the breaks are single stranded and can be repaired by joining the broken components in the original order. At higher exposures, however, double strand breaks occur and the odds for repair decrease. Also, high-LET radiations cause more damage to the DNA molecule because of the double strand breaks. If the cell is not repaired, it may suffer a minor functional impairment or a major consequence (cell death). If DNA damage occurs in germ cells, future offspring may be affected.
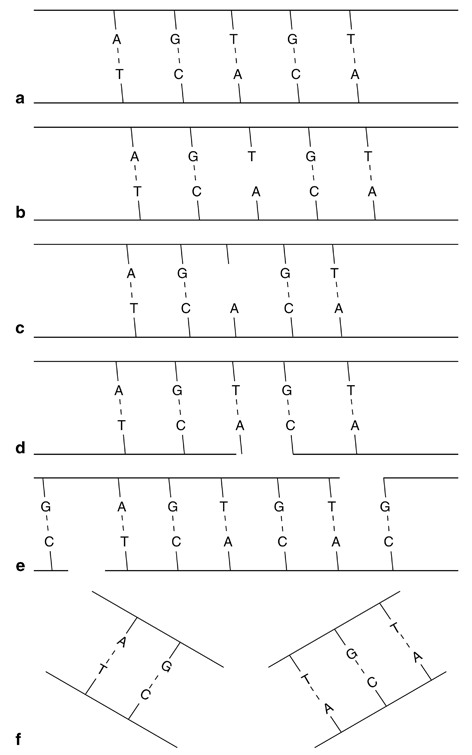
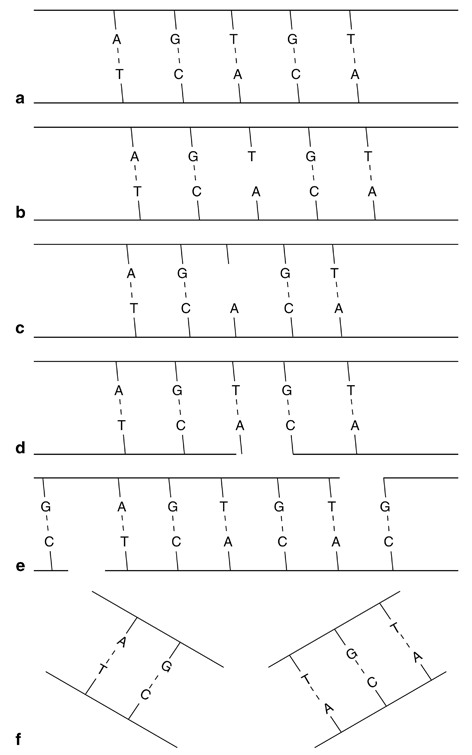
Fig. 15.6
Illustration of radiation effects on DNA molecules: a Normal DNA molecule; b hydrogen bond is broken without the loss of the base; c hydrogen bond is broken with the loss of the base Τ; d single strand break that can repair; e double strand breaks which are well separated and can repair; f double strand breaks that are too close to repair.
Chromosome
Chromosomes are likely to be affected by mutations of the DNA molecules . However, chromosomes themselves can be cleaved by radiation producing single or double breaks in the arms. These structural changes are called aberrations , anomalies, or lesions. These aberrations are categorized as chromatid aberrations and chromosome aberrations . In chromatid aberrations, irradiation occurs after DNA synthesis prior to mitosis and thus only one chromatid will be affected. On the other hand, in chromosome aberrations, irradiation occurs after mitosis prior to DNA synthesis and hence the broken chromatids will be duplicated producing daughter cells with damaged chromosomes.
Whether chromosome aberrations are induced by single-strand breaks or double-strand breaks in the structure determines the fate of the cell. In single-strand breaks, the chromosome tends to repair by joining the two fragments in a process called restitution , provided sufficient time is allowed. The cell becomes functionally normal and replicates normally (Fig. 15.7a). However, if the fragments are replicated during DNA synthesis prior to restitution, two strands with centromeres and two strands without centromeres will be produced. Random combination of these fragments will then produce acentric and dicentric chromatids as illustrated in Fig. 15.7b. Such chromosomes suffer severe consequences due to the mismatch of genetic information .
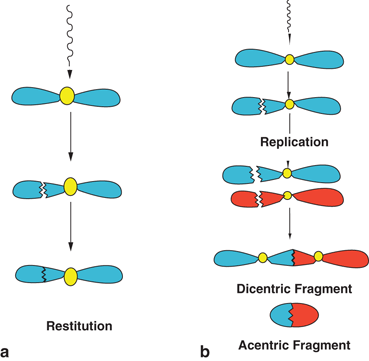
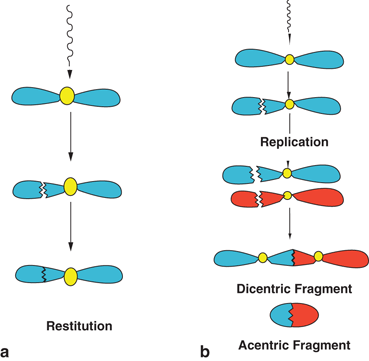
Fig. 15.7
a Illustration of restitution, in which the fragments produced by a single-strand break in one arm of the chromosome by radiation join together to produce the original chromosome. b Formation of dicentric and acentric chromosomes by combination of the fragments, after replication from a single-strand break in a chromosome.
If radiation produces single-strand breaks in two separate chromosomes, then there are four ways of recombining the broken ends as shown in Fig. 15.8. The dicentric and acentric combinations (Fig. 15.8a) are similar to those formed after replication of single strands in the same chromosome shown in Fig. 15.7b. However, these cells suffer severe consequences because of the mismatch of genetic information from two separate damaged chromosomes. The translocation is a process in which two fragments—one with a centromere from one chromosome and one without a centromere from another chromosome—combine to form a new chromosome (Fig. 15.8b). In another scenario, radiation can cause two breaks in one arm of a chromosome, resulting in three fragments, only two of which combine with the loss of the third. Such a process is called deletion (Fig. 15.9a). Translocation and deletion, although not as harmful to the cell, cause late effects such as carcinogenesis and hereditary effects due to mismatch or loss of genetic material. An alternative to deletion is the combination of all three fragments into a chromosome with changes along the broken line as shown in Fig. 15.9b. This process is called inversion , which has all the original genetic material except a change in the sequence of genes and hence is not as detrimental to the cell.
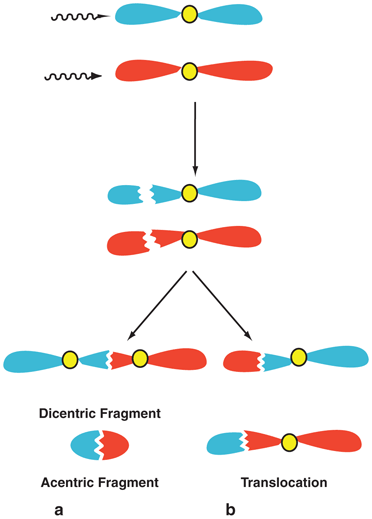
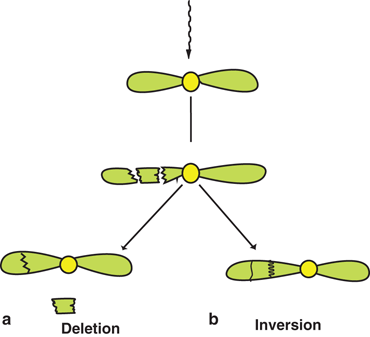
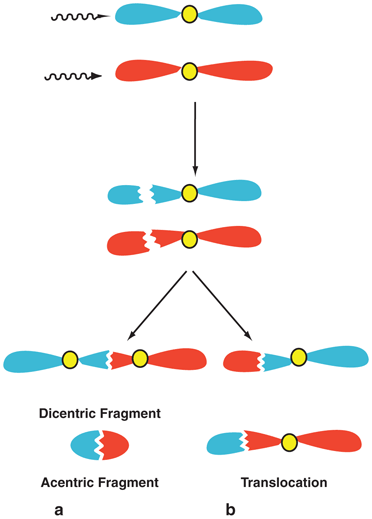
Fig. 15.8
Single-strand breaks in one arm of each of two separate chromosomes. Combination of these four fragments leads to dicentric and acentric chromosomes a or translocation b.
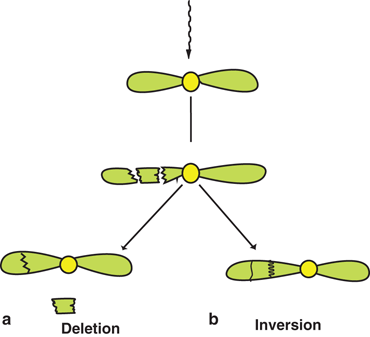
Fig. 15.9
Two breaks in one arm of a chromosome producing three fragments. a In deletion, two of the fragments combine with the loss of the third, or b in inversion, all three fragments combine with the interchange of positions.
Repair of chromosomes after irradiation depends on the sites of break in the DNA molecule or the chromosome, the total radiation dose, the dose rate , and the LET of the radiation. Chromosome aberrations by double-strand breaks occur more frequently at high-dose rates than at low-dose rates because of less time to repair and fewer chances of combining two fragments in correct sequence of genes. High-LET radiations cause more double-strand breaks in chromosomes than low-LET radiations, and thus repair becomes difficult in the former. For example, α-particles, protons, and neutrons will cause more chromosome aberrations than γ-rays.
Direct and Indirect Actions of Radiation
The DNA molecule of a cell is the most sensitive target to radiation. Radiation damage to the cell can be caused by the direct or indirect action of radiation on the DNA molecules . In the direct action, the radiation hits the DNA molecule directly, disrupting the molecular structure (Fig. 15.10). Such structural change leads to cell damage or even cell death. Damaged cells that survive may later induce carcinogenesis or other abnormalities. This process becomes predominant with high-LET radiations such as α-particles and neutrons, and high radiation doses.
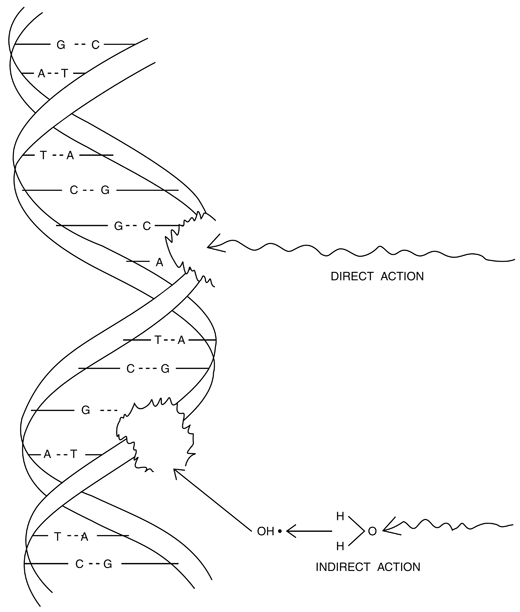
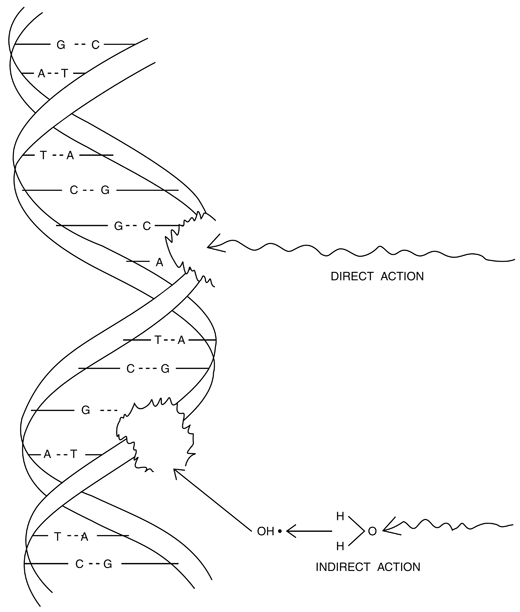
Fig. 15.10
Illustration of direct and indirect action of radiation on the DNA molecule. In direct action, radiations hit the DNA structure directly, whereas in indirect action, radiations produce free radicals in the cytoplasm, which react adversely with the DNA molecule to cause structural damage.
In the indirect action , the radiation hits the water molecules, the major constituent of the cell, and other organic molecules in the cell, whereby free radicals such as perhydroxyl (HO2•) and alkoxy (RO2•) are produced. A variety of reactions that can occur after radiation interacts with water molecules is shown below.
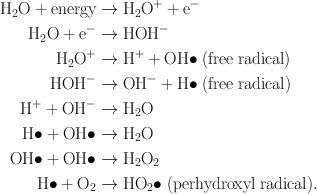
Free radicals are characterized by an unpaired electron in the structure, which is very reactive, and therefore reacts with DNA molecules to cause a molecular structural damage (Fig. 15.10). Hydrogen peroxide, H2O2, is also toxic to the DNA molecule. The result of indirect action of radiation on DNA molecules is the impairment of function or death of the cell. The number of free radicals produced by ionizing radiation depends on the total dose but not on the dose rate . It has been found that the majority of radiation-induced damage results from the indirect action mechanism because water constitutes nearly 70 % of the composition of the cell.
Radiosensitivity of Cells
In living matter, there are two types of cells: differentiated and undifferentiated. Undifferentiated cells do not have any specific physiologic function except to develop into mature cells. They undergo mitosis and serve as the precursors for mature cells. In contrast, all mature cells are differentiated and perform specific functions in the living body. For example, red blood cells (RBCs) are mature and differentiated cells performing the function of oxygen carriers , whereas erythroblasts are undifferentiated cells that develop into RBCs through mitosis.
According to the law of Bergonié and Tribondeau, undifferentiated cells that are undergoing active mitosis are most sensitive to radiation , and differentiated or mature cells are least affected by radiation. For example, in a sample of mixed RBCs, erythroblasts are most damaged and mature RBCs are least affected by radiation. Undifferentiated cells that are killed by radiation may be replaced by new cells, but those that survive with defective DNAs can induce late effects, such as cancer (see later). In contrast, the S phase of DNA synthesis in the cell cycle is least radiosensitive. Radiosensitivity is best assessed by cell death. For differentiated cells , it means loss of cellular function, whereas for undifferentiated cells it means loss of reproductivity.
Groups of cells and their relative radiosensitivity are listed in Table 15.1. As can be seen, lymphocytes, though mature cells, are most sensitive to radiation, owing to a large nucleus; nuclear material is more radiosensitive. Nerve cells and muscle cells are totally differentiated cells and therefore are highly resistant to radiation . The tissue or organ that contains more radiosensitive cells will be highly radiosensitive and vice versa. For example, bone marrow containing radiosensitive erythroblasts is very radiosensitive, whereas nerves and muscles containing radioresistant cells are less radiosensitive. Following irradiation of blood, depressed blood counts are observed as follows: lymphocytes on the same day, granulocytes in 3 days, platelets in 6 days, and RBCs in 10 days.
Table 15.1
Different types of cells and their radiosensitivity
Types of cellsa | Radiosensitivity | |
---|---|---|
VIM | Mature lymphocytes | Highly sensitive |
Erythroblasts | ||
Spermatogonia | ||
DIM | Myelocytes | Relatively sensitive |
Intestinal crypt cells | ||
Basal cells of epidermis | ||
MCT | Osteoblasts | Intermediate sensitivity |
Spermatocytes | ||
Chondroblasts | ||
Endothelial cells | ||
RPM | Spermatozoa | Relatively resistant |
Granulocytes | ||
Erythrocytes | ||
Osteocytes | ||
FPM | Nerve cells | Highly resistant |
Muscle cells | ||
Fibrocytes |
Cell Survival Curves
When mammalian cells are irradiated, not all cells are affected to the same extent. Different factors such as the total dose, the dose rate , the LET of the radiation, the particular stage of the cell cycle (M, G1, S, or G2) and the type of cell will affect the radiation-induced damage. Some cells may die and some will survive. The cellular response to radiation is illustrated by what is called the cell survival curve . It is obtained by plotting the dose along the linear X-axis and the surviving fraction along the logarithmic Y-axis. Surviving cells are those cells that retain all reproductive as well as functional activities after irradiation, whereas the death of cells is indicated by the loss of their function in differentiated cells and by the loss of reproductive activity in undifferentiated cells . It should be noted that thousands of grays are needed to kill differentiated cells, whereas only hundreds of grays are needed for undifferentiated cells.
Typical cell survival curves are shown in Fig. 15.11. For high-LET radiations such as α-particles and low-energy neutrons, the survival curves are nearly a straight line starting from the lowest doses. In contrast, for low-LET radiations (e.g., x- and γ-radiations), the survival curve exhibits an initial shoulder, followed by a straight line. This straight line portion on the semilog plot is an exponential curve on a linear plot. This curve based on a multitarget model is characterized by three parameters: D 0 (dose at which 37 % of cells survive), the extrapolation number n , and the quasithreshold dose D q , and they are related by the expression.
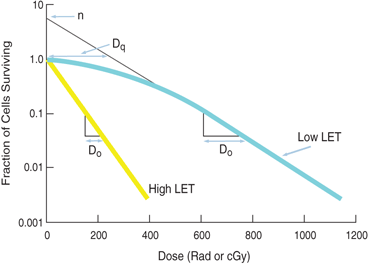
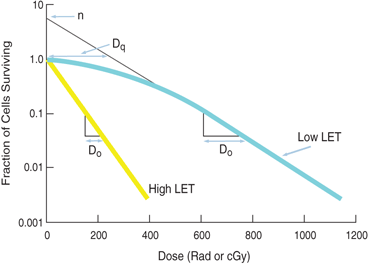
Fig. 15.11
Typical cell survival curves. The cell survival curve for low linear energy transfer (LET) radiations shows a shoulder of width D q , which is called the quasithreshold dose. After D q , the plot becomes linear on a semilog scale, indicating an exponential dose–response relationship. The extrapolation number n is obtained by extrapolating the linear portion of the curve back to the ordinate. D 0 is the dose obtained from the slope of the linear portion of the curve, at which 37 % of the cells survive. The survival curve for high-LET radiations shows no or little shoulder, indicating D q to be zero and n to be unity.

(15.1)
The quasithreshold dose, D q , is the dose given by the width of the shoulder of the curve. The D q indicates that, at low doses, almost all cells repair after irradiation, and cell killing is minimal, which is due to very limited radiation damage to the cell.
D 0 is determined from the slope of the straight line portion of the survival curve. It is the dose that kills 63 % of the total number of cells. The value of D 0 is a measure of radiosensitivity of a given type of cell. For example, a large value of D 0 for a type of cell means that the cells are less radiosensitive and vice versa.
The extrapolation number n is obtained by extrapolating the straight line portion of the survival curve back to the Y-axis. Its value depends on the width of the shoulder of the survival curve, that is, the quasithreshold value, D q . Its value for mammalian cells varies between 1 and 10.
Although Eq. (15.1) has some merit in expressing cell killing by radiation, the linear-quadratic model provides a more accurate description of the radiation-induced cell killing. This model is mathematically expressed as

(15.2)
where S is the survival fraction of the cells irradiated with dose D and α and β are constants. For low-LET radiations, βD2 is negligible at low doses, and the cell survival is proportional to the dose only, making the survival curve linear (Fig. 15.12). At higher doses, the cell survival is proportional to the square of the dose, and the curve tends to bend becoming concave downward (Fig. 15.12). For high-LET radiations, β is zero, and so the survival curve becomes linear.
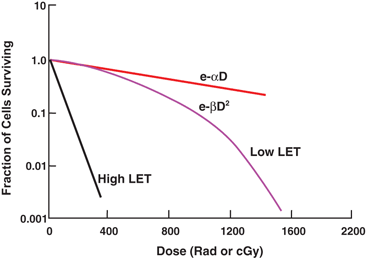
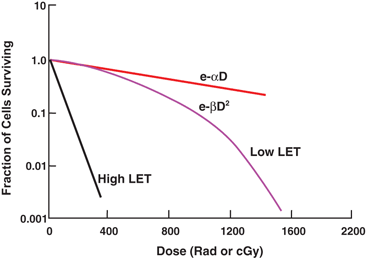
Fig. 15.12
Cell survival curves based on linear-quadratic model. The initial slope of the linear-logarithmic plot gives cell killing proportional to e −α D and the latter part to
which bends at higher doses. With high-LET radiations, β is zero, and the curve is exponentially expressed simply by e −α D .

Factors Affecting Radiosensitivity
As already mentioned, various factors affect the radiation damage in the cell and hence the survival curve. The dose rate , the LET of the radiation, the presence of chemical molecules and the stage of the cell cycle all affect the survival curve.
Dose Rate
The dose rate , that is, the delivery of dose per unit time, is an important factor in cellular damage. The higher the rate of dose delivery, the greater will be the cell damage. At low-dose rates, only single-strand breaks of DNA molecules occur, and so cells have time to repair, whereas at high-dose rates double-strand breaks occur, and so repair is less likely to occur because of the shorter time available to the cells between ionizing events. Figure 15.13 illustrates the effects of two dose rates on the cell survival curve . The dose-rate effect is very important in radiation therapy, because unless an appropriate dose rate is prescribed, intended therapeutic effect may not be achieved . When a total dose is given to a patient in fractions over a period of time, it should be kept in mind that the interval between fractional doses should be short enough to keep repair of damage to abnormal cells to a minimum.
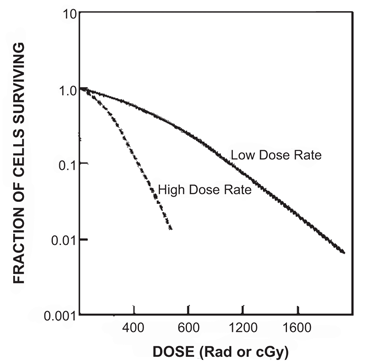
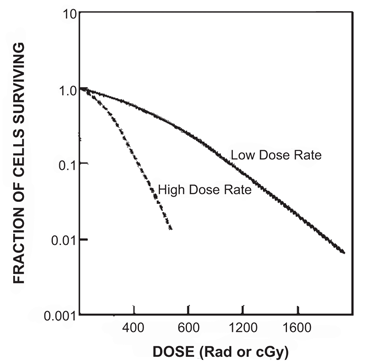
Fig. 15.13
The cell survival curves indicating the effect of dose rates. At high dose rates, the shoulder of the curve is reduced, with smaller values of D q . The opposite is true at low dose rates.
Linear Energy Transfer
High-LET radiations (e.g. α-particles, neutrons) do not exhibit a dose-rate effect on the survival curve. Also at high-dose rates (above 100 rad/min) of low- and moderate-LET radiations, no dose-rate effects are observed on the survival curve in contrast to low-dose rates. Thus, high-LET radiations exhibit no shoulder (i.e., no D q ) on the survival curve resulting in an extrapolation number of 1 (Fig. 15.12). High-LET radiations are densely ionizing radiations causing more double-strand breaks in the DNA molecules, and thus leading to more cell deaths than low-LET radiations, which are sparsely ionizing radiations (e.g. x-rays, γ-rays). Radiation damage by high-LET radiations is so severe that the chances of repair are minimal, and even if repair takes place, the cell is likely to be defective.
Chemicals
Several chemicals, if present during irradiation, have been found to augment or diminish the effects of radiation on cells . Agents that enhance the cell response to radiation are celled radiosensitizers , and those that protect cells from radiation-induced damage are called radioprotectors .
Radiosensitizers
Oxygen
Oxygen is the best-known sensitizer encountered in radiation biology. It has been found that hypoxic cells are resistant to radiation, whereas oxygenated cells are highly radiosensitive . Such radiosensitization by oxygen is called the oxygen effect and is measured by a quantity called the oxygen enhancement ratio (OER) . The OER is given by the ratio of the dose required to produce a given radiation damage to cells in the absence of oxygen to that required to produce the same damage in the presence of oxygen. The oxygen effect occurs only when oxygen is administered simultaneously with radiation . It increases with O2 tension up to 30 mm Hg, and remains constant at higher O2 tension. For mammalian cells, the oxygen concentration required to produce a radiation response midway between hypoxic and aerobic conditions is approximately 0.5 %. The OER value reaches a maximum of 3.0 for x- and γ-radiations, whereas it is about unity for high-LET radiations such as α-particles.
Figure 15.14 illustrates the effects of oxygen on the survival curve. The presence of oxygen makes the curve much steeper, indicating the augmentation of cellular damage at smaller doses relative to the situation of no oxygen. The mechanism of the oxygen effect is not clearly understood but is most likely related to DNA strand breaks. It has been postulated, however, that oxygen combines with already formed free radicals, R•, to produce peroxidyl group RO2•, which is more damaging to the DNA molecules. While normally R• could recombine with complementary molecular components to repair the cell,
is an altered chemical entity and cannot help in cell repair. The oxygen effect is most predominant for γ– and x-rays, and is practically absent for high-LET radiations (e.g., α-particles).

