Chapter 37 Radionuclide imaging
Chapter contents
37.1 Aim
The aim of this chapter is to introduce the reader to the basis of the technology used in radionuclide imaging. The information available on a nuclear medicine scan will be compared with the information available on other forms of medical imaging.
37.2 Basic concept of radionuclide imaging
The basic concept of radionuclide imaging involves a three-stage process:
37.3 Production of artificially produced radionuclides
37.3.1 The nuclear reactor
The nuclear reactor (or pile) produces heat energy by controlled fission (see Sect. 19.9). The heat generated within the reactor raises the temperature of a coolant which in turn is used to heat water to produce steam. The steam can then drive very powerful electric generators. This is the basis of nuclear power stations.
A simplified diagram of such a reactor is shown in Figure 37.1 (see page 272). The controlled fission is produced by using the neutrons of fissile decay to produce further fission in other atoms. Thus, a sustained reaction can be set up where one neutron released during the fission of a nucleus will interact with another nucleus to produce fission of that nucleus with the release of further neutrons.
37.3.2 The technetium generator
The technetium generator used in nuclear medicine is an important example of the production of artificial radionuclides. As mentioned in Section 37.3.1, if molybdenum-98 is placed in a neutron stream, the nuclei of the molybdenum atoms can be made to absorb the neutrons to produce molybdenum-99. The capture of a neutron raises the energy of the resulting molybdenum-99 nuclei and each loses this energy by the prompt emission of a gamma-ray.
A molybdenum-99/alumina column is in the centre of the generator, as shown in Figure 37.2 (see page 272). The molybdenum-99 has a half-life of 67 hours and decays to form technetium-99m by β−-particle emission, as shown below:
37.3.3 Production of radionuclides using a cyclotron
The type of cyclotron used in nuclear medicine to produce artificial radionuclides by the bombardment of stable substances will briefly be described. A simple diagram of such a device is shown (see Fig. 37.3) (see page 272). The cyclotron consists of an evacuated cylinder which has an ion source placed at its centre. Ions from this source are influenced by strong axial and radial magnetic fields. This causes acceleration of the ions in circular paths of increasing radius. This ion beam achieves significant velocity and can be made to interact with materials placed at the exit port of the cyclotron. This interaction causes nuclear changes in these materials and we can produce neutron-deficient nuclei (see Sect. 19.5.1) which are capable of positron emission. Such materials form the basis of the radiopharmaceuticals used in positron emission tomography (PET) scanning. Figure 37.4 (see page 272) shows a photograph of such a medical cyclotron.
37.4 Clinically useful radionuclides
Radionuclides are used to diagnose and to treat certain conditions. When they are used for diagnosis, they may be labelled (chemically linked) to a certain radiopharmaceutical, thus encouraging their uptake by specific body parts. The labelled radionuclide may then be injected into, or ingested by, the patient. Such diagnostic techniques in nuclear medicine have three main uses:
1. To provide numerical or graphical information on organ physiology, e.g. technetium-labelled diethylene triamine penta-acetic acid (DTPA) will give information on the rate of excretion of this radionuclide by the kidneys, thus giving information on renal function.
2. To produce an image of organ physiology on a gamma camera, e.g. as pertechnetate may be injected into the patient to produce images of the skeletal physiology; this is for very useful early detection of metastatic spread into bone.
3. To produce information on organ physiology using PET scanning (see Ch. 40
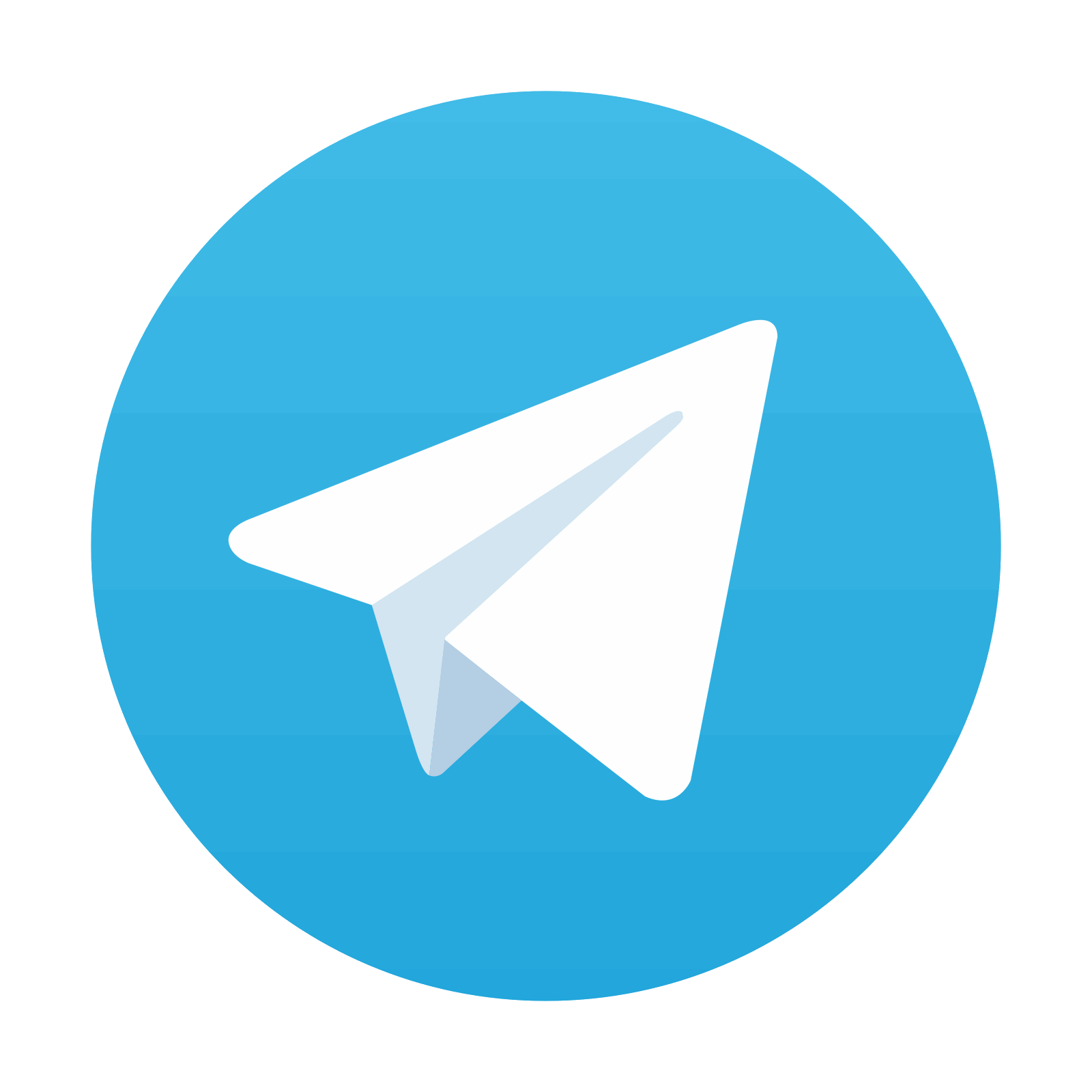
Stay updated, free articles. Join our Telegram channel

Full access? Get Clinical Tree
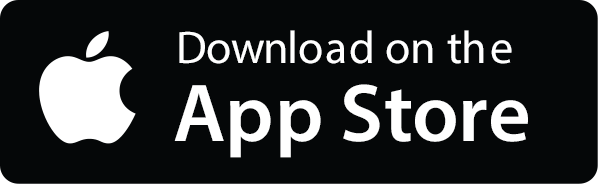
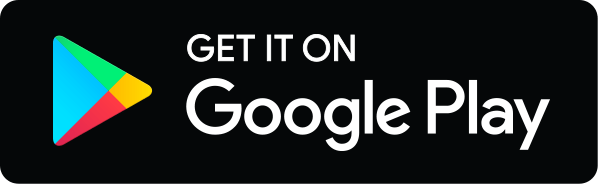