Fig. 1
Classical action of the glucocorticoid signaling mediated by the GR. Unliganded receptor is located in the cytosol associated with several heat shock and other chaperone proteins including HSP90, HSP70, HSP40, P23, and FKBPs (shown by light blue shades around GR). Ligand binding leads to conformational alterations in the GR, and by doing so GR dissociates from these associated proteins, and ligand bound GR is free to translocate to the nucleus. This process appears to be phosphorylation dependent. Once in the nucleus, GR dimerizes and binds to site-specific DNA binding sequences and interacts with several other coregulatory proteins including coactivators and proteins from the basal transcription machinery such as SRCs, CBP/p300, DRIP/TRAP, TBP, GRIP1, and several others (shown by different shapes and shades) in the nucleus, and subsequently leading to transcriptional regulation. The cofactor(s) bound are determined by their levels in particular cell types and by the state of the folded structure of the GR. The complex alters local chromatin structure, e.g., by catalysing histone acetylation or deacetylation, and affects the stabilization of the transcription pre-initiation complex [TATA-box-binding protein (TBP)–TBP-associated factors (TAFs)–RNA polymerase II (RNA Pol II. The activity of kinases and phosphatases regulating signaling pathways also contribute to this process by altering the state of phosphorylation of both receptor and cofactors (not shown)
The molecular mechanism of ligand-dependent regulation of GR activity has been illustrated by the crystal structure of ligand-bound LBD which highlights the importance of the conformational dynamics of helix-12 in forming the AF2 surface on the LBD allowing interactions with LxxLL motifs found in coregulatory proteins, including coactivators and/or corepressors (Bledsoe et al. 2002; Rosen and Miner 2005 ) . This phenomenon has extensively been exploited for the evolution of small molecule selective glucocorticoid receptor modulators driven by the demand of lowering the unwanted side effects, while keeping the beneficial anti-inflammatory effects (He et al. 2014; Rosen and Miner 2005) . Potency and efficacy are two key pharmacokinetic parameters of glucocorticoids and the observed mechanisms of action based on the LBD structure to increase potency and efficacy of several newly designed glucocorticoids (Fig. 2) have served as starting leads for the development of novel therapeutics for the treatment of inflammatory diseases (Simons 2008; Simons 2010; Frey et al. 2004) . Potency is an important aspect of this evolution as many undesirable side effects are associated with the use of high-dose glucocorticoids, which can be minimized by highly potent glucocorticoids that may achieve similar treatment effects at lower doses.
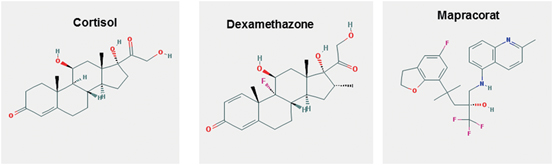
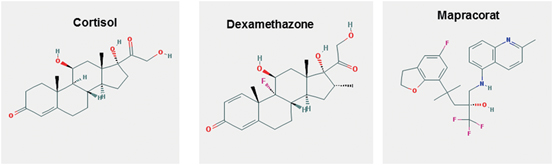
Fig. 2
Structure of some glucocorticoid molecules. (source: PubChem structure search)
Given its biological and pharmaceutical importance, there has been enormous interest in elucidating the 3-dimensional structure of full length GR. Since GR is highly homologous to mineralocorticoid (MR/NR3C2), androgen (AR/NR3C4), and progesterone (PR/NR3C3) receptors, when determined the structure should serve as a model for understanding the roles of ligand binding, GRE binding, coactivator recruitment, and receptor dimerization among others in the signaling pathways mediated by these steroid receptors. In this article, we discuss the current understanding of the available structural information of the GR with a special emphasis on the importance of conformational dynamics that plays a critical role in the receptor action, and how this information can be used in structure-based drug design, which could serve as lead compounds for the development of novel therapeutics for the treatment of inflammatory diseases.
2 Glucocorticoid Receptor Isoform Expressions and Cell/Tissue Specific Transcriptional Activity of Glucocorticoid-Responsive Genes
The human GR protein is encoded by exons 2–9 located on chromosome 5, and due to alternative splicing gives rise to several GR protein subtypes (Fig. 3). The GR NTD is encoded primarily by exon 2, the DBD is encoded by exons 3 and 4, and the hinge region and LBD are encoded by exons 5–9 (Lu and Cidlowski 2005) . Alternative splicing in exon 9 near the end of the GR primary transcript generates two receptor isoforms, termed GRα and GRβ (Oakley and Cidlowski 2011) . GRβ is prevalent in many cells and tissues but generally is found at lower levels than GRα. Elevated levels of GRβ have been associated with glucocorticoid resistance in a variety of inflammatory diseases. The GRα-A generated from the first translation initiation codon is often termed as the classic full-length receptor protein consisting of 777 amino acids (Yudt and Cidlowski 2001; Oakley and Cidlowski 2011). Other GRα isoforms resulting from alternative RNA splicing and translation initiation of the GRα transcript that possess additional unique properties may differentially regulate GR target genes depending on their selective and relative expression in specific tissues/cells (Oakley and Cidlowski 2011) .
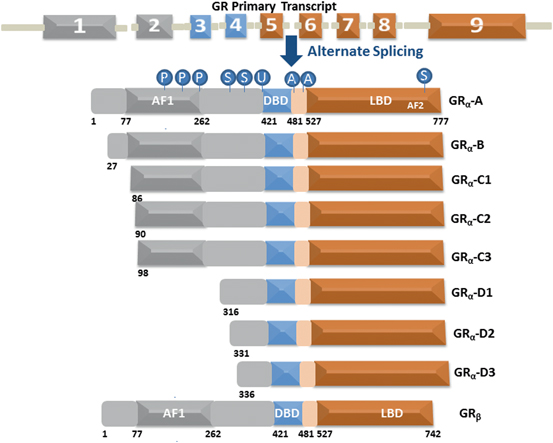
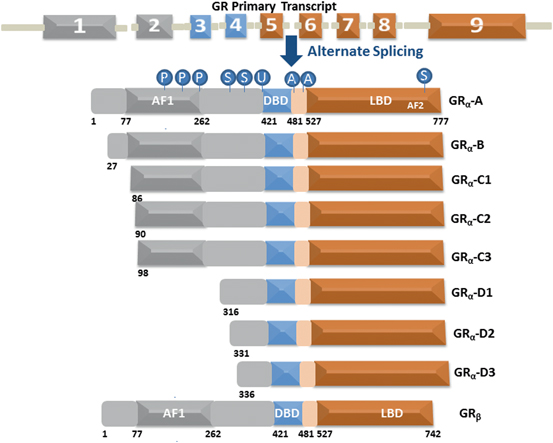
Fig. 3
The GR undergoes alternative processing to yield multiple functionally distinct subtypes. Human GR contains 9 exons with the protein coding region formed by 2–9. The GRα undergoes alternative translation initiation in exon 2, generating GRα-A, GRα-B, GRα-C1, GRα-C2, GRα-C3, GRα-D1, GRα-D2, and GRα-D3 isoforms with truncated NTD. GR is a modular protein containing NTD, DBD, LBD, and a flexible “hinge region” separating the DBD and the LBD. The NTD and LBD possess transcriptional activation function regions AF1 and AF2, respectively. GR undergoes multiple posttranslational modifications including phosphorylation (P), SUMOylation (S), ubiquitination (U) and acetylation (A)
Each individual GR isoform originating from alternative processing of the GR gene is subject to a variety of post-translational modifications that further modulate function and expand the repertoire of receptor subtypes available for glucocorticoid signaling. Normally these splice variants have shortened NTD and unhindered DBD and LBD sequences that makes them capable of producing DBD/DNA and ligand/LBD binding with similar affinity as that of GRα-A. Since they all possess intact DBD and LBD including the hinge region, it is logical to argue that in the absence of ligand binding all the receptor isoforms should also possess similar affinity for HSP90 and other chaperone machinery proteins and nuclear translocation signals resulting into their localization to the cytosols. However, recent studies have shown that they display differences in their cytosolic and nuclear distribution (Lu and Cidlowski 2005; Oakley and Cidlowski 2011) .
This raises an important question: does NTD sequences play role in nuclear translocation, nuclear export and/or cytoplasmic retention of the receptor? This is an important question and answering it may not only play a critical role in defining the tissue/cell specific effects of GR isoforms, but may also provide a better understanding of target-specific gene regulation leading to the design of new therapeutic drug. The GRα translational isoforms display a similar affinity for glucocorticoids and a similar capacity to interact with GREs following ligand activation; however, they show marked differences in other properties. It has been reported that the human GR isoforms that lack AF1 sequences (e.g., GRα-D) are virtually inactive in their ability to transactivate glucocorticoid-responsive genes (Lu and Cidlowski 2005; Oakley and Cidlowski 2011) . On the other hand, isoforms that lack amino acid sequences (GR-C isoforms; C1, C2, and C3) prior to or in the early part of AF1 produce differing GR activities in a cell-dependent manner, suggesting that NTD sequences around AF1 may play roles in regulating GR activity (Kumar and Thompson 2010; Kumar and Thompson 2012) . Recent evidence suggests that isoform GRα-C3, which lacks amino acids located on the N-terminal flanking sequences of AF1, appears to be most active in displaying the transcriptional activity of glucocorticoid-responsive genes than the other hGRα isoforms, suggesting that this flanking region may be inhibitory to GR activity (Kumar and Thompson 2010; Kumar and Thompson 2012) .
It has been hypothesized that the increased activity in the N-terminal truncated GR may be due to its effects on AF1 conformation and subsequent coactivator interaction (Lu and Cidlowski 2005; Kumar and Thompson 2012). In a recent study, it was found that the removal of all flanking sequences around AF1 affects its structure such that otherwise intrinsically disordered AF1 acquires more helical structure when in direct proximity of the DBD (Kumar and Thompson 2010). The results from this study show that placing AF1 immediately upstream from the DBD leads to acquisition of an ordered conformation in AF1. Furthermore, when proximity to the DBD caused AF1 folding, this region bound CREB binding protein (CBP/p300), but not steroid receptor coactivator-1 (SRC-1), suggesting that specific region adopts differing folded configurations to bind various factors. Also, deletion of NTD sequences flanking both sides of AF1 increased GRE-mediated AF1 activity, suggesting that these flanking sequences play an inhibitory role in regulating GR AF1 structure and activity. Together these results provide a mechanism explaining why certain N-terminally truncated GR isoforms and possibly other steroid hormone receptors differ in their actvities.
Previous studies have shown that the binding of a two-domain GR fragment (consisting of entire NTD through the end DBD) to a consensus GRE sequences leads to imposition of higher secondary/tertiary structure in the NTD/AF1 whereas the AF1 bound directly to the DBD had structure and did not gain any further secondary/tertiary structure when the DBD was bound to a GRE (Kumar et al. 1999; Kumar and Thompson 2003) . It therefore appears that in the full two-domain GR protein, relatively more energy is required to fold AF1 than in the construct wherein AF1 is directly hooked to DBD. This energy presumably is provided by DBD binding to its GRE. Though preliminary in nature, this maybe an important phenomenon and a detailed analysis of these observations could in fact provide a rationale for promoter specific GR activity. Yamamoto and coworkers have shown the differential effects of binding various GRE sequences on the functions of the GR that correlated with slight variations in DBD structure and lent strong evidence supporting the suggestion that a DNA sequence-specific allosteric coupling between the DBD and AF1 plays a critical role in GR activity (Meijsing et al. 2009) .
3 Structure of the Glucocorticoid Receptor and Control of the Glucocorticoid-Mediated Receptor Actions
Although structures of the independently expressed recombinant proteins of the DBD and LBD of the GR have provided major advances in our understanding of the molecular basis of transcription regulation by GR (Fig. 4), a complete description of structure-function relationships and mechanism of action of GR necessitates structural studies of the full-length receptor protein. However, as yet the structure of full length GR has been elusive as has been the case with all other members of the steroid receptor subfamily of proteins. The three-dimensional structures of the DBD and LBD, expressed as independent polypeptides, have been solved, and show overall folds that represent globular proteins with natively-ordered conformations (Luisi et al. 1991; Bledsoe et al. 2002) . Solution NMR structure of the DBD was the first structure determined for any region of the GR followed by crystal structure of DBD complexed with DNA sequences, showing that DBD contains two perpendicularly oriented α-helices. One of these helices is responsible for site-specific DNA recognition at the GREs sequences (Härd et al. 1990; Luisi et al. 1991) . Consistent with the known cooperative binding kinetics of the DBD to the GRE, the structure shows a DBD dimer on the palindromic GRE. As a consequence of the unnatural four base-pair spacer, however, the two monomers in the dimer show a differential binding to the GRE. When in solution DBD structure was determined by NMR, a significant conformational flexibility in the parts of the second zinc finger was observed, which was well resolved in the crystal, perhaps due to stabilization upon formation of the dimeric DBD-DNA complex (Hard et al. 1990; Meijsing et al. 2009) . Further, molecular dynamics simulation and free energy perturbation studies suggest that the binding of the DBD to the GRE distorted conformations for bases at positions 5 and 6, propagation of which through the DNA may facilitate cooperative binding of another monomer at half-site (Baumann et al. 1993; Meijsing et al. 2009; Eriksson and Nilsson 1995) .
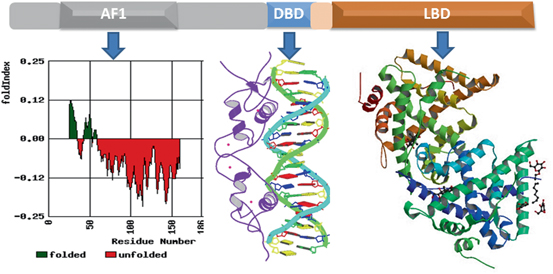
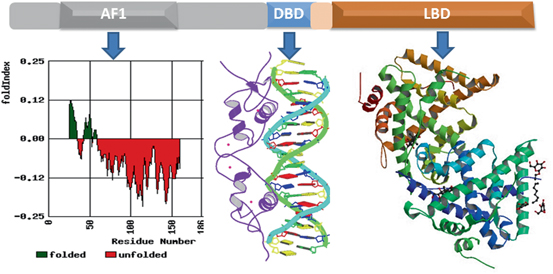
Fig. 4
Structure of the GR protein. Upper panel shows topological diagram of GR. Lower middle panel shows 3-dimensional structure GR DBD in complex with GRE (Structure 1GLU from PDB protein databank; Luisi et al. 1991). Lower right panel shows 3-dimensional structure of ligand-bound GR (PDB structures 1 M2Z; Bledsoe et al. 2002). Lower left hand panel shows Index of the probability of AF1 sequences for the propensity to fold. The red color shows the probability of unfolded and green color for the probability of folded regions. Fold Index was performed (http://bip.weizmann.ac.il/fldbin/findex) as described (Prilusky et al. 2005)
Recent structural analyses have highlighted the allosteric properties of DNA binding suggesting that receptor binding sites associated with glucocorticoid-regulated genes influence the composition of coregulatory complexes recruited by the GR (Watson et al. 2013; Meijsing et al. 2009) . The binding of the GR-DBD to different response elements was found to adopt different conformations depending on the DNA architecture (Meijsing et al. 2009). The altered structure of the DBD depended on the base sequence of the response element to which it was bound, and demonstrated functional consequences as to the strength and selectivity in gene induction (Meijsing et al. 2009; Schiller et al. 2014) . These structural studies reveal the conformational dynamics imparted by DNA recognition/binding and highlight the potential regulatory role of different DNA sequences or binding coregulators, which could lead to cell or promoter selective GR function.
The crystal structure of the GR LBD consists of 12 helices that fold overall into a globular structure consisting of three sets of helices that form the sides and top of the globule, making a central pocket, the ligand-binding site where the steroid molecule binds (Bledsoe et al. 2002) . The helix-12 contains the sequence for the important AF2 function, the site upon which binding of coregulatory proteins depends. Helix-12 changes position upon ligand binding, flipping from an “open” position to one closed over the bound ligand resulting into agonist-bound LBD presenting a surface favorable for binding the coactivators whereas some ligands that act as antagonists appear to cause helix-12 conformation to close in a position that creates an unfavorable surface for coactivator binding (Carson et al. 2014; Schoch et al. 2010; Tao et al. 2008) . In spite of having lot of similarities with the crystal structures of several other nuclear receptors, there are some unique features such as formation of a dimer interface, which is different to that seen in the ER structure (Bledsoe et al. 2002; Brzozowski et al. 1997) . Importantly, mutations that can disrupt this dimerization results into the loss of glucocorticoid-mediated GR transactivation, but not transrepression activity (He et al. 2014; Bledsoe et al. 2002) .
From these crystal structures, it was initially thought that both the DBD and LBD have a stable, well-ordered globular structure; however, as we learned more and more about them, it became clear that each is more structurally dynamic than once believed. The LBD responds to various ligands in a gene-selective fashion, implying that such “selective modulators” affect LBD/AF2 structure (De Bosscher 2010) . Availability of LBD crystal structure and further understanding of the conformational dynamics of the structural model of helix-12 folding/unfolding with agonist and antagonist ligands explains some data that has extensively been used to design small molecule selective GR modulators for clinical use. However, still unexplained is the fact that some antagonists act as agonists in specific tissues/cells. Compared to DBD and LBD, structurally the NTD is least explored even though AF1 located in the NTD was established long before AF2 to be the major transactivation region and that much of the GR’s transcriptional activity depends on this domain (Miesfeld et al. 1987; Hollenberg et al. 1987; Dahlman-Wright et al. 1994; Dahlman-Wright et al. 1995) .
The major obstacle in determining the structure of NTD has been due to the fact that NTD exists in an intrinsically disordered conformation or as an ensemble of conformers that lacks any significant stable secondary/tertiary structures (Dahlman-Wright et al. 1995; Baskakov et al. 1999) . This phenomenon has been observed for many transcription factors including the steroid receptors (Kumar and McEwan 2012) . In recent years, it has become quite evident that intrinsically disordered proteins are highly dynamic capable of adopting structural flexibilities that can be manipulated for the interaction with the target molecules such that in multi-protein assembly specific components of protein sub-sets could either be included or excluded in a very rapid fashion. The flexible AF1 domain is ideally suited to provide such modulated surfaces in the GR, which requires assembly of specific coregulatory proteins to regulate target gene expression in a promoter and cell/tissue specific manner. Available data strongly suggest that conditional folding/stabilization of the GR NTD/AF1 is the key for these interactions and subsequent transcriptional activity (Henriksson et al. 1997; Ford et al. 1997; Almlof et al. 1998; Warnmark et al. 2000; Kumar et al. 2001; Khan et al. 2012; Khan et al. 2011a, b) . How NTD/AF1 folds and what kind of functional folded conformation it adopts are open questions, actively being pursued. The data indicate that such a folded conformation could be caused by the presence specific solute molecules such as osmolytes, which are present in cells under physiological conditions (Kumar et al. 1999; Kumar et al. 2001; Kumar et al. 2007; Khan et al. 2011a) .
These studies have shown that when GR AF1 is incubated in increasing concentrations of osmolytes, the protein cooperatively folds into a compact, monomeric structure such that AF1 selectively binds TATA box-binding protein (TBP), CREB-binding protein (CBP) and a member of the steroid receptor coactivator-1 (SRC-1) family of proteins (Kumar et al. 2001; Kumar et al. 2007; Khan et al. 2011a) . Another study demonstrates that when a two-domain form of the GR lacking LBD but containing the entire NTD region through the complete DBD binds to a DNA oligomer containing a complete palindromic GRE, secondary and tertiary structure is induced in the NTD/AF1 (Kumar et al. 1999) . It therefore seems appropriate to think of the DNA sequence of a GRE as an allosteric ligand that can modulate the structural dynamics of the NTD/AF1 (Lefstin and Yamamoto 1998) . It has been proposed that this allosteric effect results in a partially folded, “cocked” AF1 conformation, such that it can readily recognize certain coregulatory proteins critical for transcriptional regulation (Kumar and Thompson 2003; Kumar et al. 1999; Kumar and McEwan 2012) . This means that a considerable amount of binding energy must be diverted to cause conformational changes in the NTD/AF1 through intramolecular rearrangements. Together, these data suggest that GR and by extension other steroid receptor mechanism of action should include the role of DNA as an allosteric effector, with both local and remote DBD-specific effects. This model of the DNA as allosteric ligand in no way rules out the possibility of further structural modulations in NTD/AF1 (or the entire GR) as a result of steroid binding to LBD, protein:protein interactions involving both AF1 and AF2, and of course post translational modifications.
GR-mediated glucocorticoid signaling is a multifaceted process involving cross talk with various regulatory kinase pathways (Beck et al. 2009; Chen et al. 2008) . Thus, signaling cascades that induce phosphorylation of the GR and its coactivator proteins are critical factors in determining the physiological actions of the GR. It has been shown that under physiological conditions, site-specific phosphorylation plays a crucial role in allowing the intrinsically disordered AF1 domain of the GR to adopt functionally active conformation such that the resulting structurally modified forms of AF1 suits for its varied interactions with other critical coregulatory proteins and possibly additional modulations in its structure essential for gene regulation by the GR (Garza et al. 2010; Chen et al. 2008) . It has been proposed that these interactions give a set of functionally active folded structure to AF1 region and form the basis for the multiprotein assemblies involved in GR-mediated regulation of transcription (Kumar and Thompson 2012; Kumar and McEwan 2012; Simons et al. 2014) . Characterization of phosphorylation and other posttranslational modifications in inducing AF1 structure formation in facilitating protein-protein interactions should be of particular importance in understanding the mechanism by which kinase(s) regulate the transcriptional regulation of the glucocorticoid-mediated target genes in the nucleus. Further, phosphorylation-induced functional conformation in GR AF1 may also shed light on why most of the major known phosphorylation sites are located in the NTD of the GR and several other nuclear receptors.
There are many examples in which the intrinsically disordered region of a protein undergoes disorder-to-order transition upon interaction with particular binding partner (Reid et al. 2002; McEwan et al. 1996; Kumar and McEwan 2012; Demarest et al. 2002; Kumar et al. 2004a) . Coregulatory proteins influence or modulate the transcriptional activity of the GR by multiple mechanisms. In addition to the LXXLL motifs of the p160 steroid receptor coactivators (SRCs) that bind to AF2, an amino-terminal fragment of SRC2/TIF2, binds to the NTD of both PR and GR (Wang et al. 2007) and increases the α-helical content of the GR AF1 domain, suggesting that coactivators augment the transcriptional activity of GR-agonist complexes by inducing more ordered structures beyond the LBD/AF2 region (Khan et al. 2012) . Studies have also shown that NTDs of steroid receptors including GR, MR, PR, ER are capable of undergoing a disorder-to-order that results in an increase in α-helical content and tertiary folding upon interaction with the TATA-binding protein (TBP). Additionally, this TBP-induced folding of the NTD was observed to be associated with enhancement of AF1-mediated transcriptional activity (Kumar et al. 2004b; Kumar et al. 2013; Warnmark et al. 2001; Fischer et al. 2010) . A potential mechanism by which TBP affects NTD/AF1 activity is to facilitate binding of SRC-1 to NTDs and cooperate with SRC-1 to stimulate NTD-dependent transcriptional activity via reorganizing or stabilizing NTD/AF1 structure for recognition by alternative surfaces of SRC-1 and possibly other proteins in the assembly of coactivator complexes (Kumar et al. 2004b; Khan et al. 2011b; Kumar et al. 2013; Warnmark et al. 2001; Fischer et al. 2010) . These data collectively suggest that of the AF1/NTD, rapidly and reversibly adopt multiple structural conformations that can sample the cellular environment until a protein target of proper concentration/affinity are found to selectively stabilize a functional conformation of the NTD. The significance of these findings lies in the possibility of therapeutically targeting AF1 surfaces to achieve tissue-restricted effects with small molecule drugs that can inhibit interactions with protein binding partners such as TBP. Therefore, identifying potential target molecules that could modify AF1-TBP binding may provide the additional selectivity needed to target GR-selective genes and thereby reduce the number of undesirable side effects in current endocrine-based therapies.
4 Influence of Conformational Dynamics in the Regulation of the Cell/Tissue Specific GR Target Genes
The recent X-ray studies of other nuclear receptors such as the structure of the HNF4α homo-dimer bound to DNA and complexed with a coactivator peptide, and the structure of DNA-bound full-length PPARγ/retinoid X receptor (RXR/NR3B1)α heterodimers revealed the possible problems associated with the structure determination (Chandra et al. 2008; Chandra et al. 2013) . Further, these studies revealed how allosteric communication between LBD and DBD may trigger extensive physical and functional inter-domain interactions that define their total activity as opposed to each domain functioning entirely independently. However, these studies failed to account for the structural and functional contributions of the NTD due, mainly, to the role of NTD’s flexible conformation that could trigger allosteric regulation within and outside the domain. Allosteric communication between receptor domains has been demonstrated in GR and other nuclear receptors (Chandra et al. 2008; Chandra et al. 2013; Watson et al. 2013; Kumar et al. 1999; Zhang et al. 2011; Helsen et al. 2012; Brodie and McEwan 2005) .
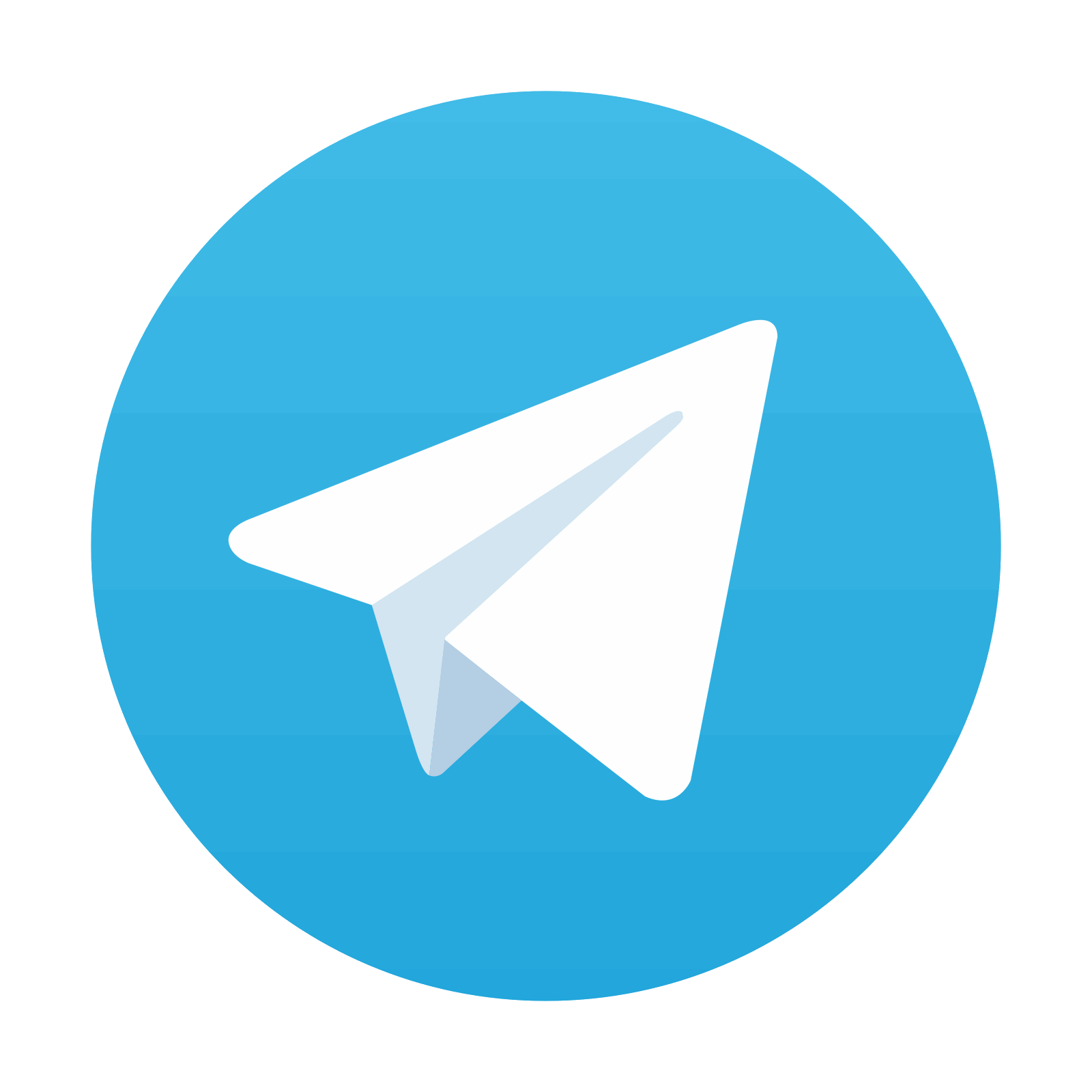
Stay updated, free articles. Join our Telegram channel

Full access? Get Clinical Tree
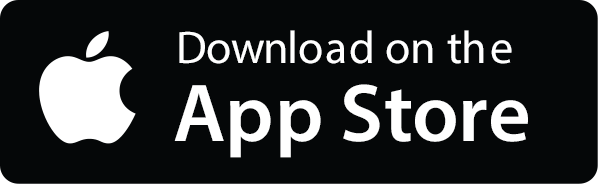
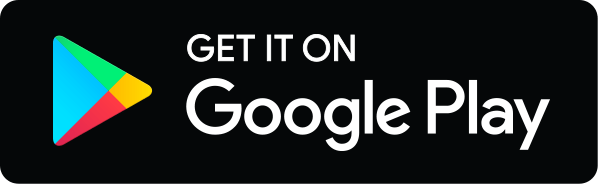