Fig. 39.1
Gate control theory
Building on the gate control theory, Shealy et al. [1] implanted a 70-year-old man dying of bronchogenic carcinoma via laminotomy, sewing the 3 × 4-mm electrodes to the dura. That evening in the postoperative setting, they began stimulation with 10–50-Hz and 400-ms pulse width at 0.8–1.2 V and 0.36–0.52 mA. Both his incisional pain and original chest pain were immediately abolished. Small changes were made throughout the remaining day and the next in response to discomforts. Eventually, the patient died on postoperative day 2 from a left hemispheric embolization from subacute bacterial endocarditis. Despite the abbreviated nature of this nascent trial, Shealy et al. [1] confirmed the suspicion that pain was not only modifiable at the spinal cord level, but that the clinical effect of electrical current in the spinal canal was of great importance to the human condition. Since that moment, SCS has undergone rapid evolution towards efficacy and safety.
This chapter will detail in short the scientific foundation of implantable SCS, the clinical use of SCS including patient selection and psychological screening, and trialing and implant techniques, with special attention to concepts that may diminish risk and complications and improve likelihood of success. Throughout the chapter, resources for further reader education will be included. Lastly, future directions for research surrounding spinal cord stimulation are summarized.
Patient Selection and Diagnostic Work-Up
Mechanism of Action
Chronic pain from injury to the central or peripheral nervous system can be managed by stimulating nerve fibers in the dorsal column via percutaneous or surgical electrode array placement, first described by Dr. Shealy in 1967 [1]. The exact mechanism of action of SCS is incompletely understood, however, likely involves both physiologic, orthodromic stimulation, and nonphysiologic, antidromic stimulation, respectively [11]. While several mechanisms of action have been described for SCS, the most accepted theory of pain relief can be explained by the gate theory first described by Melzack and Wall in 1965 [10]. This groundbreaking yet simplistic model describes the interaction of large sensory fibers and nociceptors and how they influence the transmission of neural impulses by second-order projection neurons in the dorsal horn. Placement of electrode arrays in the epidural space depolarizes A-beta nerves orthodromically in the posterior columns and antidromically via dorsal column collateral fibers, thereby inhibiting pain transmission to the brain [11]. Large, A-beta nerve activation causes depolarization of neurons in the dorsal horn and “closes the gate” for the transmission of A-delta and C-fibers to the projection neuron. In addition, large A-beta neuron activation inhibits GABAnergic pathways that influence wide dynamic range neurons that can become hyperexcitable in chronic pain states. Evoked paresthesia is targeted towards the specific region of pain, although the inhibition of pain is most likely related to activation of interneurons that suppress pain and not the antidromic depolarization resulting in the evoked paresthesia noted by the patient. Since the publication of the gate theory of pain transmission, it is clear that there are additional components to the transmission and processing of pain that include descending inhibitory pathways, alterations of neurotransmitters in the brain, as well as other tracts in the spinal cord that may be involved [12, 13]. The supraspinal descending inhibitory effects likely involve serotonin and norepinephrine and clinically may be related to patients noting pain relief several hours after the SCS system is turned off.
Inhibition of the sympathetic system by SCS is another potential mechanism to alleviate pain. Kemler et al. showed that in a group of patients with CRPS, pain relief did not depend on vasodilation, arguing against the inhibition of the sympathetic system by SCS to be vital for neuropathic pain relief [14]. It is possible, however, that stimulation-induced inhibition of the sympathetic system by SCS may be beneficial in patients with peripheral vascular disease where vasodilation may help with nociceptive pain related to ischemia, and it is likely that the underlying disease plays a large factor into the mechanism that ultimately results in pain relief [15].
The type of nerve population depolarized is determined by the placement of the electrode arrays and the stimulation parameters utilized. Different neural structures have different stimulation thresholds. The lowest threshold neural structures are located in the dorsal root entry zone, followed by the lateral fibers in the posterior columns, and the highest threshold fibers are the most medial. The somatotopic organization of the central nervous system persists in the dorsal columns and lead placement, and programming strategies are determined by the target neural element [16]. While importance has been historically placed on the cephalocaudal placement of SCS leads, more attention has been placed on the medial to lateral placement as well with multicolumn arrays, showing promise in more targeted stimulation given the medial to lateral somatotopic organization of nerves in the dorsal spinal cord and their ability to create guarded arrays. For that reason, placement of percutaneous SCS leads is generally preferred to be in the physiologic midline to maximize stimulation of the dorsal columns and minimize stimulation of the dorsal root entry zone. In addition, the frequency of SCS stimulation also effects which nerve population is depolarized [17]. Higher frequencies tend to stimulate a greater proportion of A-beta fibers, while lower frequencies stimulate more A delta and C fibers.
Functional MRI has demonstrated important changes in the way pain is processed in the brain in patients with chronic pain and how SCS may influence the central nervous system. The challenges with functional magnetic resonance imaging (fMRI) evaluating SCS and peripheral nerve field stimulation (PNfS) include minimizing heating of the contacts with pulsed radio frequency (RF) as well as fMRI, revealing widespread areas of activation and inhibition in the brain and the difficulty in interpretation [18]. In a healthy patient with an occipital nerve stimulator system, areas of activation were predominantly seen in the hypothalami, the thalami, the orbitofrontal and prefrontal cortex, the periaqueductal gray (PAG), the inferior parietal lobe, and the cerebellum. Deactivation was seen in primary areas (M1, V1, A1, and S1), the amygdala, the paracentral lobule, the hippocampus, S2, and SMA [19]. Kovaks et al. noted that the effects of stimulation were more pronounced with tonic stimulation rather than burst stimulation [19].
Patient Selection
Selecting the right patient is essential for a positive response to neuromodulation therapies. Among the diagnoses that have noted consistent benefit from SCS are lumbar post-laminectomy syndrome (PLS), radiculopathy, polyneuropathy, and complex regional pain syndrome (CRPS) [20, 21]. In general, patients with a history of spinal surgery with primarily leg pain have been shown to benefit significantly from SCS. North et al. [22, 23] in their seminal study randomized patients who had a history of lumbar surgery into repeat surgery and SCS groups and showed superiority of SCS to reoperation. In addition, in the same cohort of patients, North et al. demonstrated significant cost savings of SCS to repeat back surgery in intention to treat, treat as intended, and final treatment analysis [24]. The cause of post-laminectomy syndrome likely involves changes in peripheral sensitization as well as central windup phenomena in the spinal cord. Anatomically, these patients may present with epidural fibrosis, arachnoiditis, junctional stenosis above or below surgical site, or a completely normal MRI with normal postsurgical changes. In cases of instability with movement on flexion/extension x-rays, surgical referral is warranted. In addition, significant central canal stenosis in the thoracic or cervical spine impinging the spinal cord would be a contraindication for SCS implantation.
While in the USA the primary indications for SCS are radiculopathy, PLS, and polyneuropathy, emerging applications are being shown in Europe. The benefit of SCS in widespread small vessel coronary artery disease with chronic angina has been described [25]. In addition, there has been some evidence that the ability of SCS to cause vasodilation can potentially treat the underlying cause of peripheral vascular disease as well as mask its symptoms [26].
Case reports of more challenging diagnosis to treat with SCS have been reported including postherpetic neuralgia [27], post-thoracotomy syndrome [28], phantom limb pain, stroke central pain syndrome [29], spinal cord injury, and multiple sclerosis [30]. Case reports and small case series have been published describing benefit from SCS therapies in these patient populations, and it is unclear why these diagnoses have such variable response to treatment.
Outcomes/Cost-Effectiveness
Multiple studies have demonstrated clinical efficacy of SCS, with reduction in pain, improvement of function, and reduction in pain medicines well documented (see Table 39.1). In addition, SCS has been shown to be cost effective in several studies. North et al. [24] demonstrated significant cost savings in a cohort of patients who had spinal surgery when comparing SCS to repeat spine surgery. The cost for success for SCS was $48,357 compared to the cost for success with repeat spine surgery being $105,928 (treated as intended). Mekhail et al. compared patients with SCS systems to those without and noted a yearly cost savings of $30,221 per year attributed to less ER visits, less diagnostic tests, and lower utilization of health-care resources [31]. Of note, the timing of SCS implantation may be of great importance as Van Buyten et al. has shown a significant reduction in efficacy when neuromodulation therapies are delayed with 85 % of patients realizing significant pain relief within 2 years of their pain beginning compared to 9 % of patients who have had pain for more than 15 years [32].
Table 39.1
SCS outcome data
References | Number of patients | Follow-up | Results |
---|---|---|---|
Kumar et al. [20] | 410 | 8 years | 74 % had >50 % relief |
Cameron [32] | 747 | Up to 59 months | 62 % had >50 % relief |
Van Buyten et al. [31] | 123 | 3 years | 68 % had good to excellent relief |
Aló et al. [93] | 80 | 30 months | Mean pain scores declined from 8.2 at baseline to 4.8 |
Psychological Evaluation for SCS
Background
Pain is well recognized as being multidimensional and multifactorial. Therefore, any therapy designed to affect pain, particularly in the chronic pain setting, should logically include a comprehensive or multidisciplinary evaluation. The psychological assessment should be considered as an integral and significant aspect of this evaluation process given the well-documented impact of psychosocial variables on the experience of pain.
Scores of studies involving thousands of chronic pain patients utilizing SCS therapy have been published. Although the results are touted as generally positive (the majority of patients report a 50 % or greater reduction is pain), reviews of the literature [33–35] have revealed a loss of pain relief in up to 50 % of patients at 1–2 years post-implant despite their having successfully “passed” a period of trial stimulation and the presence of a functional SCS unit. Indeed, one study [36] reported that 100 % of patients reported success at 16 months but only 59 % at 58 months. Psychological factors may play an important role in understanding this apparent loss of efficacy. An overemphasis on the SCS technology, while potentially economically rewarding (new power supplies, electrodes with more contacts, increased programming options, multiple electrode arrays), may come up short of a solution. In part, it may be equally advantageous to take the position of trying to discover “how to make what works, work better” rather than merely “tinkering with the technology.” It is important to remember that the SCS trial is essentially an “acute” procedure being used to “predict” a “long-term” outcome with a “chronic disease.” While computer modeling provides invaluable information about electrode disbursement patterns at the level of the spinal cord, it should not be mistaken to represent anything other than a guideline to achieving more specific patterns of stimulation, which may or may not be associated with greater and more prolonged pain relief.
Inclusion/Exclusion Criteria
Previous guidelines for “patient selection” have focused on exclusion criteria. For example, Daniel et al. [37] cited personality disorders [38], drug dependence, unstable family and personal relationships, poor vocational adjustment, and involvement in litigation/compensation as “red flags.” Nelson et al. [39] suggested the presence of suicidality or homicidal, severe depression or other mood disorders, somatization/somatoform disorder, alcohol or drug dependency, unresolved compensation/litigation issues, lack of social support, and/or neurobehavioral cognitive deficits to be considered as contraindications of SCS therapy. In a conversation with Dr. Kumar (April 2004), he developed the “Kumar warning signs” which include K – “kannot” possibly live without this device; U – unlimited utilization, overuse of health-care resources; M – misunderstood, “nobody understands me, only you can help me doctor”; A – affective disorder, major psychopathology; and R – “REALLY…its only my pain I have no other problems,” symptoms inconsistent with physical findings. The European Federation of IASP Chapters [51] declared major psychiatric disorders (active psychosis, severe depression or hypochondria, and somatization disorder), poor compliance and/or insufficient understanding of the therapy, lack of appropriate social support, and drug and alcohol abuse or drug-seeking behavior as contraindications.
Doleys [40] adopted a different approach when outlining 16 “hypothesized” positive indicators including a history of compliance with previous treatments, behavior and complaints consistent with pathology, behavioral/psychological evaluation consistent with patient complaints and reported psychosocial status, realistic concerns regarding “illness,” mildly depressed, generally optimistic regarding outcome, and ability to cope with setbacks without responding in an emergent fashion. He went on described patient and physician beliefs “potentially” associated with positive and negative outcomes. In each of the cases above, the features outlined emerged from consensus, “common sense,” clinical experience, and/or generalization of other literatures. None at this point has been experimentally validated and replicated.
The frequency with which a formal psychological assessment is carried out betrays what appears to be an almost universal acceptance of the role of psychological factors in chronic pain and pain therapies, including SCS. A Canadian survey [41] found that only 25 % of 13 participating centers reported routine psychological screening prior to SCS implantation, compared to 61 % of centers in a UK survey [42]. Although a “psychological screening” is required by Centers for Medicare & Medicaid Services (CMS) (Medicare health-care insurance) in the USA, the incidence of it in non-Medicare populations approximated 25 % [43]. The most common reasons given for the discrepancy between the support for psychological screening and its actual utilization were (a) lack of or inadequate insurance coverage, (b) lack of physician insistence, (c) patient refusal, and (d) lack of an appropriate evaluator. The cost of most psychological evaluations approximates 1–3 % or less of the total cost of the SCS trial and implantation in the USA. If screening/pretreatment prevented only 2–3/100 patients from failing treatment and the removal of the device, a cost savings would be realized [43].
Brief Literature Review
Celestin et al. [44] performed a systematic review of outcomes relating to lumbar surgery and SCS. They were able to identify only four SCS studies that met their criteria for the review. A successful outcome was defined as 6 months or more duration, decreased pain, increased function, and reduced health-care utilization. Depression, anxiety, somatization, poor coping, and hypochondriasis tended to be associated with poorer outcomes, but none was found to be statistically significant. Sparkes et al. [45] examined the literature on psychological variables affecting SCS outcomes spanning 1982–2008. In summarizing their review, they noted that the Minnesota Multiphasic Personality Profile (MMPI) [46] was the single most common test administered. The psychological variables studies included depression, hysteria, anxiety, mania, hypochondriasis, paranoia, defensiveness, joy, belief pain out control, catastrophizing, and psychopathic deviate. It was concluded that (a) depression probably correlates negatively, (b) mania possibly has a positive correlation, (c) hysteria was possibly negative, and (d) hypochondriasis was mixed [45].
One of the coauthors (DMD) recently participated as a member of group of clinicians and researchers organized by the American Pain Foundation to develop a consensus guideline for SCS therapy (in preparation). Nineteen studies from among several hundreds covering the period from 1990 to 2010 were selected on the basis of the information in their respective abstracts to be reviewed regarding their reported use of a psychological/psychiatric evaluation prior to SCS trialing or internalization. Twelve of the 19 studies noted using one or more psychometrically validated instruments or questionnaires. The content or makeup of the psychological screening was often based on theoretical bias. For example, Heckler et al. [47] and Molloy et al. [48] tended to favor behavioral/functionally oriented tests/questionnaires, Dumoulin et al. [49] a more psychoanalytic approach, and Lamé et al. [50] focused on the hypothesized impact of catastrophizing.
Only seven studies listed any psychological inclusion or acceptance criteria. Most often, the criteria was stated in the form of a very general statement, for example, “no contraindications,” or “psychologically uncomplicated.” Eight studies outlined psychological exclusion criteria. The often cited exclusion criteria were high levels of psychological distress, psychosis, somatization, alcohol/drug issues, and/or unresolved secondary gain issues, for example, pending litigation. Fourteen studies performed some type of pre post-internalization analysis on one or more of the tests results from the pre-implant screening. In nearly every case, this analysis was carried out for the purpose of determining the effect of SCS therapy. A few studies addressed psychological “predictors.” In one, depression, especially when combined with age and the McGill score, predicted 88 % of 34 patients. Lamé et al. [50] found that catastrophizing did not predict outcomes in a group of CRPS patients. North et al. [51] reported that none of their psychological tests predicted the outcome, and Kupers et al. [52] and May et al. [36] noted that the patients with a “positive screen” did better than patients where caution or reservations were entered. In some cases, the emphasis was on predicting those who would proceed from trial to implant versus “outcome” predictors. A variety of tests/questionnaires were used. The MMPI, Beck Depression Inventory (BDI) [53], and the Oswestry Disability Questionnaire (ODQ) [54] were among the most common. It may be difficult to establish a commonly agreed upon interview format and battery of tests/questionnaires. Perhaps, the best approach at this point is to follow Deyo et al. [55] recommendation to obtain information regarding the qualitative/quantitative aspect of “pain,” along with an assessment of mood, function, and personality features.
Elements of Psychological Evaluation
Unfortunately, the psychological evaluation is often construed as part of patient “selection.” As such, the goal is seen as one of “clearing” the patient, a concept that has never been defined, clarified, or objectified [56]. This approach encourages a dichotomous decision of “go” or “no-go,” with little regard to interventions that might improve the probability of a good long-term outcome [57]. It is herein suggested that the patient selection/evaluation aspect of SCS therapy should be considered as a process and not an event. The process begins with the review of records and the initial consultation and extends through the trial and up to the point that a decision is made to internalize or not. A systematic behavior/psychological assessment is part of the process. Patient selection is best conceptualized as longitudinal and focused on the identification of patient characteristics that implicates the patient as a good candidate for SCS or is potentially modifiable by psychological/behavioral interventions (CBT) designed to enhance the short- and long-term outcomes.
For the psychological evaluation to provide the most useful information and therefore to be of minimum benefit to the patient and clinician, the following components are recommended. First, the assessment should be conducted by an appropriately trained, knowledgeable, and experienced mental health clinician. Second, it should include a face-to-face interview with the patient and when possible the participation of a significant other. Data from the clinical interview should be supplemented by the use of well-known and validated test (s)/questionnaires. At least one of the clinical instruments employed should contain a mechanism for detecting dissimulation (i.e., a “fake-bad” or “fake-good” scale). The use of generic (overall quality of life) and disease-specific (pain rating) measures should be considered. It would be important for the evaluator to have post-trial and/or post-internalization contact with the patients, or least the outcome data, to determine the accuracy of the recommendations generated by the evaluation.
Unfortunately, the psychological evaluation all too often is treated necessary nuisances. Patients are sent to “outside” consultants with little or no interest or experience in chronic pain or SCS therapy merely as a means of satisfying insurance or regulatory guidelines. Worse, computer-scored and interpreted tests are administered by the physician as a mechanism to satisfy the requirement. Even when such tests are administered by a psychologist, the American Psychological Association Ethical Guidelines [58] requires patient contact prior to rendering an interpretation of the test results. Finally, periodic updated brief assessments may well assist in adjustments of the therapeutic algorithm and improving long-term outcomes [59].
Despite the potential limited prognostic value, the evaluation process can and should serve several other functions [60]. First, it can be used to facilitate the development of an individualized treatment plan. Second, it provides an opportunity to properly prepare and educate the patient and significant other for the trial, possible internalization, and long-term treatment. Third, psychological interventions designed to mitigate the impact of maladaptive psychological issues (i.e., poor coping, limited acceptance, etc.) can be implemented and create a patient with a more favorable prognosis. And finally, the psychological evaluation process can be used as a means of addressing potentially modifiable problems and therefore may enhance the overall efficacy of therapy and prevent an overemphasis on the development of absolute exclusionary criteria. It can also help to fulfill the requirements of “informed consent”[61].
Our own approach to the evaluation process includes a clinical interview along with the administration of the BDI, McGill Pain Questionnaire, ODI, and MMPI. The assessment period is also used as an opportunity to educate the patients and significant other as to the various aspects of SCS therapy as well as allowing them to become familiar with the hardware by manipulating it. Addressing expectations that can be supported by the existing outcome literature and identifying functionally related goal which can be measured during the trial are fundamental. We also obtain a “functional level of pain” (FLOP). That is, the patient is asked at what level of pain do they feel they can be more accepting of their condition, existing residual pain, and more functional. Patients needing a pain level of less than 3/10 may be very unrealistic and require further education.
Summary
To the extent that “pain” remains a primary or significant outcome, and given the generally accepted multidimensional and biopsychosocial nature of pain, it seems logical and consistent to recommend a pre-SCS trial/implant psychological screening. In addition to pursuing the identification of “predictors,” emphasis should also be given to the development of treatment algorithms based on the psychological evaluation. This algorithm may well call for pre- and/or post-implant psychological intervention(s).
Clinical Approaches to Spinal Cord Stimulation
Complications
Once the decision to trial SCS has been made, great attention to planning is required. Every effort, increasing awareness of risk, mitigates that risk and compounds the probability of success for the patient and surgeon. Despite a familiarity with the operating room, many implanting physicians’ native medical training falls outside the surgical realm and thus attention to detail becomes paramount. Familiarity with the most common complications sharpens the implanter’s level of surveillance for missteps and pitfalls. Deer and Stewart [61] familiarizes some of the more common complications of SCS in Table 39.2.
Table 39.2
Complications associated with spinal cord stimulation and their diagnosis and treatment
Complication | Diagnosis | Treatment |
---|---|---|
Complications involving the neuraxis | ||
Nerve injury | CT or MRI, EMG/NCS/physical exam | Steroid protocol, anticonvulsants, neurosurgery consult |
Epidural fibrosis | Increased stimulation amplitude | Lead reprogramming, lead revision |
Epidural hematoma | Physical exam, CT, or MRI | Surgical evacuation, steroid protocol |
Epidural abscess | Physical exam, CT or MRI, CBC, blood work | Surgical evacuation, IV antibiotics, ID consult |
Postdural puncture headache | Positional headache, blurred vision, nausea | IV fluids, rest, blood patch |
Complications outside the neuraxis | ||
Seroma | Serosanguinous fluid in the pocket | Aspiration, if no response surgical drainage |
Hematoma | Blood in pocket | Pressure and aspiration, surgical revision |
Pain at generator | Pain on palpation | Lidoderm patches, injection, revision |
Wound infection | Fever, rubor, drainage | Antibiotics, incision and drainage, removal |
Device-related complications | ||
Unacceptable programming | Lack of stimulation in area of pain | Reprogramming of device, revision of leads |
Lead migration | Inability to program, x-rays | Reprogramming, surgical revision |
Current leak | High impedance, pain at leak site | Revision of connectors, generator or leads |
Generator failure | Inability to read device | Replacement of generator |
Reported complication rates are variable and difficult to interpret across populations and studies; however, Turner et al. [63] identified an overall complication rate of 34 %, a rate of surgical revision at 23 %, and deep infection at 0.1 %. Kumar et al. [64] found infection rate of 2.7 %, epidural fibrosis to affect 19 %, and a lead complication rate of 5.3 %. Cameron [33] summated that lead migration complicated 13.2 % of cases, infection affected 3.4 %, and lead fracture 9.1 %. Complication severity is highly variable varying from unwanted paresthesia to epidural abscess, hematoma, and paralysis. Nevertheless, careful preoperative risk assessment moderates risk and should begin not when entering the operating theater but with the patient and as soon as the treatment is considered.
Preoperative Risk Assessment
Risk reduction begins long before consideration of trial. Risk of neural injury is diminished by a thorough survey focusing on the anatomy of the spine and contents. The implanter needs awareness of anatomical or surgical changes, scarring or fibrosis, and canal stenosis or listhesis that could challenge the deployment of a percutaneous or possibly a paddle lead. Imaging, not only the spinal canal at the neural target, but also the approach will greatly aid in safety and avoidance of difficulty [65]. Anatomy may degrade or change and impair the safety of the implant in the years following implant and narrow cervical canals. For example, it may portend further degradation and stenosis with resultant cord compromise in time.
Concomitant disease states beg recognition and exploitation of treatment, and there should be a low threshold for involvement of primary care clinicians or specialists to assist in rejuvenation prior to surgery. Diseases of immunity such as diabetes as well as recent or ongoing infections such as human immunodeficiency virus increase the probability of infection. The bleeding profile should be assessed and any medications or interactions that influence bleeding appreciated and removed from the clinical setting in adequate time to allow normal hematologic function. New-generation anticoagulants without clear data regarding surgical bleeding risk, for example, should encourage partnership with cardiology or hematology. Education regarding preoperative prophylaxis against methicillin-resistant Staphylococcus aureus with antiseptic soaps and use of intranasal mupirocin may reduce operative infection [66, 67]. Hair clipping around the operative area may additionally be of benefit [68, 69]. Compliance issues need attention, and lastly, the patient needs adequate education regarding, and durable documentation reflecting, not only potential harms but also their role and responsibility in the endeavor in the short- and long-term.
The Percutaneous Trial of Spinal Cord Stimulation
The trial not only introduces the patient to the experience of paresthesia and tests the ability to meaningfully ameliorate pain but functions also as a provisional assessment of the technique as well: the appropriateness of neural targets and the approach to them. It is the primacy of pain location and thus neural targets that backwardly determine every part of the testing and implant process. Lastly, the trial serves as a team gathering, not only of the physician and patient, but also of the stimulator representative, nurses, and family members who will be assisting in the trial and in educating the patient about their new, potential treatment.
On the trial day, a concise but thorough pre-procedure review is prudent; the addition of a checklist will ensure thoroughness. Evaluate the patient’s skin, not only at the planned operative site area, but also globally for infection. Begin preoperative IV antibiotics with efficacy against common skin pathogens in light of the local and hospital antibiogram. Within the cerebral spinal fluid (CSF) shunt literature, perioperative antibiotics administration is a significantly effective prophylactic measure [70]. Consultation with infectious disease colleagues is prudent regarding surgical standard of care especially in patients with suspect immunology or history of infection. Check coagulation status with a review of medicines taken and consider platelet count or bleeding profile if necessary. In addition, plan for positioning on the table in regards to the patient’s habitus and the optimum amount of kyphosis at the level of entry [71]. Particularly, obese persons may require significant bolstering under the abdomen in thoracic lead placement. Whatever time is lost in positioning is likely gained by facility of entry and lead positioning.
The skin is the major source of surgical pathogens, and optimization of preoperative skin antisepsis diminishes infection. Several choices of surgical preparatory agents with differing bacteriocidal specificity exist. Alcohols denature proteins and are highly effective against both gram-negative and gram-positive bacteria, even those multidrug resistant, as well as fungi, mycobacteria, and some viruses [72]. Iodine, most commonly Betadine, is effective against gram-positive, gram-negative, mycobacteria, and viruses and fungi [72]. Chlorhexadine gluconate is very effective against gram-positive bacteria, somewhat so against gram-negative bacteria, but minimally so against spores and tubercle bacilli. Chlorhexadine provides residual antiseptic activity and when combined with alcohol may be superior to iodine-based preparations [73]. Cleaning with multiple preparations may further diminish bacterial counts, due not only to the expanded action of the differing agents but also to the increased time of exposure to the agents. Prepping first with iodine-based preps followed by others may confer added benefit as blood, serum, and other protein-rich biomaterials diminish the antimicrobial effect of povidone-iodine [74]. The surgical skin preparation should extend at least 6 cm from the proposed surgical site [62], but there is no reason to limit the size of the prep. Draping with plastic adhesive border prior to the prep delineates the area to be prepped and prevents inadvertent contamination. After the skin preparation and then draping with sterile towels, taking great care not to contaminate the surgeon’s gown, a laparotomy drape or other large surgical drape covers the remaining patient. Drying of the skin permits excellent adhesion for occlusive and impregnated plastic drapes like Ioban™ (3M Health Care, Inc). There is no consensus as to the degree of surgical operative preparation for the trial and permanent implant, but arguably, the greater the prophylaxis, the lower the risk for infection.
The aim of anesthesia, patient comfort and tranquility, must be balanced with the danger of oversedation, as the most sensitive neural monitoring equipment in the awake patient. The appropriate use of local anesthetic and limitation of sedation during periods of electrode placement renders the awake and conversant patient sensitive to minute changes in neurologic status, thus alerting the physician to avoid injury.
Regarding electrode placement, the aim should be to safely depolarize the nerves, which will present paresthesia to overlap the patient’s pain. Holsheimer and Barolat have carried out significant work on neural mapping of the dorsal columns and likelihood ratios of paresthetic coverage in particular [75, 76, 77] (see Figs. 39.2 and 39.3). Alo and Holsheimer additionally summarize certain requirements for clinical efficacy:
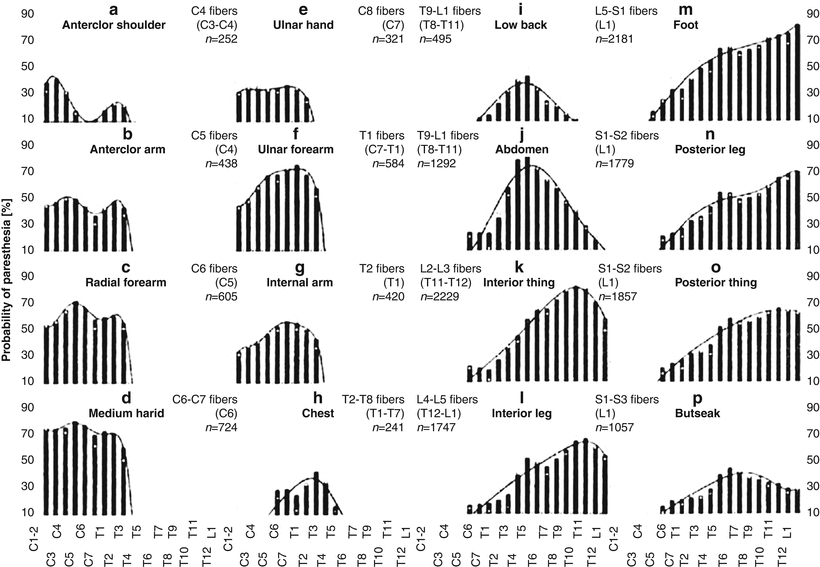
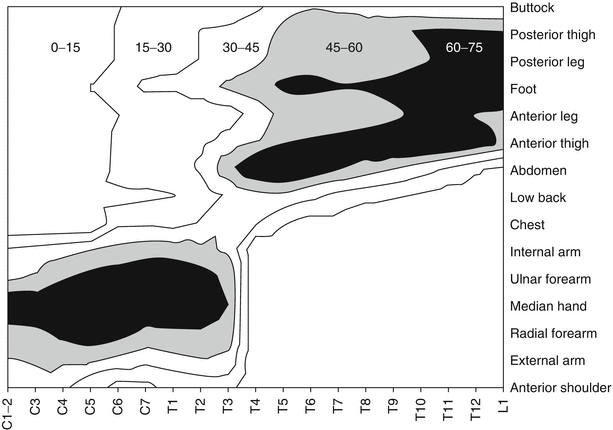
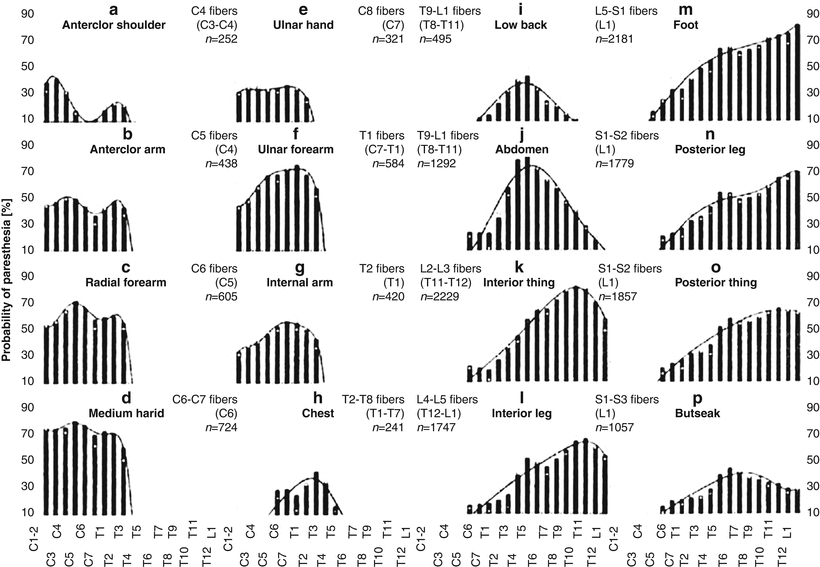
Fig. 39.2
Probability of paresthesia plots for 16 body areas as a function of the vertebral level of stimulation: white squares (original data), bars (averaged data), and curves fitting the averaged data (From Holsheimer and Barolat [75]. With permission)
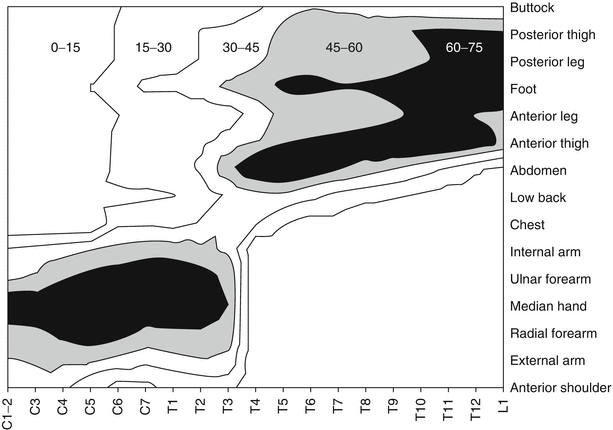
Fig. 39.3
Three-dimensional plot showing probability of paresthesia contours as a function of the vertebral level of stimulation (x-axis) and the body area (y-axis) (From Holsheimer [76]. With permission)
1.
The evoked paresthesia must consistently cover the entire painful area.
2.
The cathode and resultant electrical field must focus on the corresponding dorsal column fibers to allow maximum paresthetic coverage of the painful area.
3.
For coverage of bilateral pain, the cathode field should be focused on the “physiological” midline of the cord. In single-electrode SCS, by implication, this electrode should be centered on the spinal cord midline which may differ by up to 2 mm from the radiological midline.
4.
If the pain is unilateral, the electrode may be displaced by up to 1 mm on the corresponding side of the physiological midline.
5.
When the patient’s pain is both unilateral and segmental, the electrode may be placed more laterally, thereby stimulating primarily the corresponding dorsal root fibers.
6.
Even if requirements 1–5 are satisfied, anatomically precise paresthesia alone does not relieve pain as paresthesia is a necessary but often insufficient criterion for achieving relief.
Once the best location has been selected, then attention is turned to the site of ligamentum flavum entry. Enough lead length must lie in the epidural space proximal to the electrode array to provide stability of the lead in the space, and so entry through the flavum must occur at least one to two vertebral levels caudal to the anticipated array placement. Additionally, so as to allow for appropriate sagittal angle of incidence (less than 45°), appropriate skin entry should occur about one to one-half vertebral bodies inferior to the interlaminar entry point (see Fig. 39.4) [76]. As the depth of the spine increases, as in obese persons, the skin entry site must move inferiorly to maintain the appropriately shallow angle of needle entry. The sagittal angle is important: the flatter angle greatly increases the ease of lead manipulation especially in the first few centimeters of travel from the needle. Additionally, as the angle of incidence, needle to dura, decreases, so too does the risk of neural injury. Too flat of a needle approach, however, blocks the needle’s ability to pass under the inferior edge of the superior lamina and thus through the ligamentum flavum. Additionally, the coronal angle of the needle can be largely estimated by the angle of the chevron made by the spinous process of the inferior lamina usually 10–20° off of midline (see Fig. 39.5). If a lead persists in tracking to the contralateral side of entry, the implanter may halt attempts to pilot the lead and, if planned, introduce the second lead on the other side, providing a firm vector to recurve the lead back cephalad. If the interlaminar space permits, dual needle with unilateral approach may be also performed. While losing the benefit of “banking,” the single-sided approach allows the implanter to easily visualize the depth of the first needle, thus allowing possibly more expeditious placement of the second needle.
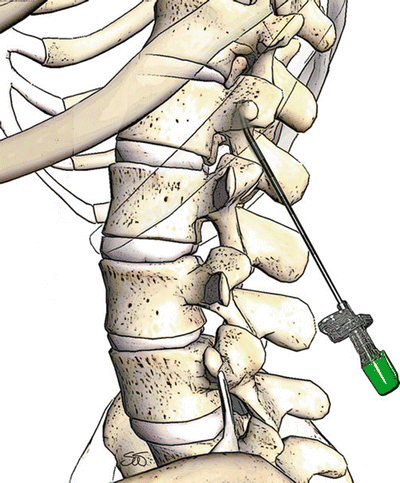
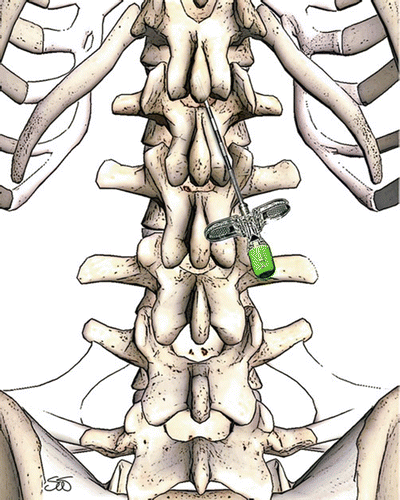
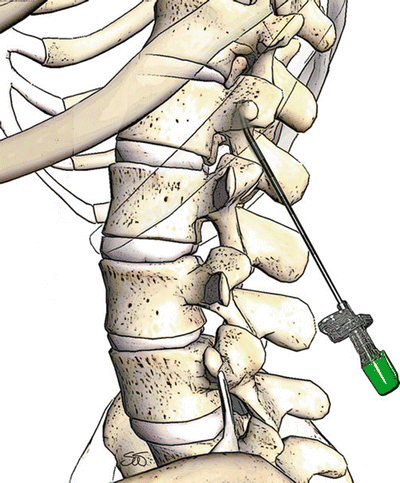
Fig. 39.4
Correct sagittal angle of incidence for thoracic spinal cord stimulator lead placement
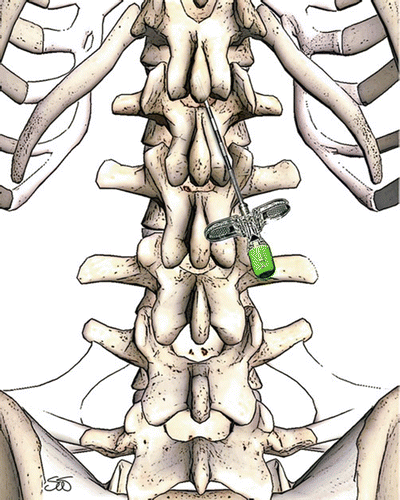
Fig. 39.5
Correct coronal angle of attack to allow midline lead deployment, and to allow for the anatomy of the spinous process
After skin entry has been selected and cutaneous anesthesia provided, a small portal is suggested with a scalpel such as an 11 blade, so both the spinal needle used for deeper anesthesia and the introducer needle passes easily between the skin edges and avoid potential contamination with superficial and intradermal flora. Loss of resistance can be performed either with a medium such as saline or air, or conversely with the lead itself, carefully advancing the needle in either contralateral oblique or lateral view with constant exiting pressure placed on the lead tip with careful advancement of the needle [78]. Entry through the ligamentum flavum should be in the medial third of the interlaminar space, thus allowing the lead entry to be close to midline. This approach when combined with the rule that cephalad lead navigation remains within the confines of the lateral borders of the spinous process projection on anterior-posterior fluoro virtually guarantees posterior epidural lead placement. However, a low threshold for lateral and confirmative views is warranted.
Clearly, the entry through the ligamentum flavum and the initial lead entry into the spine is the most dangerous aspect of the entire procedure as injury to either the dura, nerve roots, or cord can result. To fail to recognize errant placement of either needle or lead and then to subsequently proceed greatly increases the consequences of the error. As noted above, safety and subsequently expediency are served by minimal use of sedation and excellent intra-trial communication between surgeon and patient regarding new paresthesias or pain in the extremities. Gentle loss of resistance, early use of transverse fluoro angles, negative aspiration of cerebral spinal fluid, as well as lead testing for perception amplitudes consistent with epidural placement further reduce risk.
Failure to recognize error compounds the consequences. If it becomes apparent that the dura has been compromised, the surgeon must stop, survey the degree of injury, and then make the decision to either proceed with a different approach or cease and allow the dura to heal before returning. There is no clear consensus on methodology of management. Eldrige et al. detail two cases of SCS lead placement complicated by CSF lead and postdural puncture headache [80
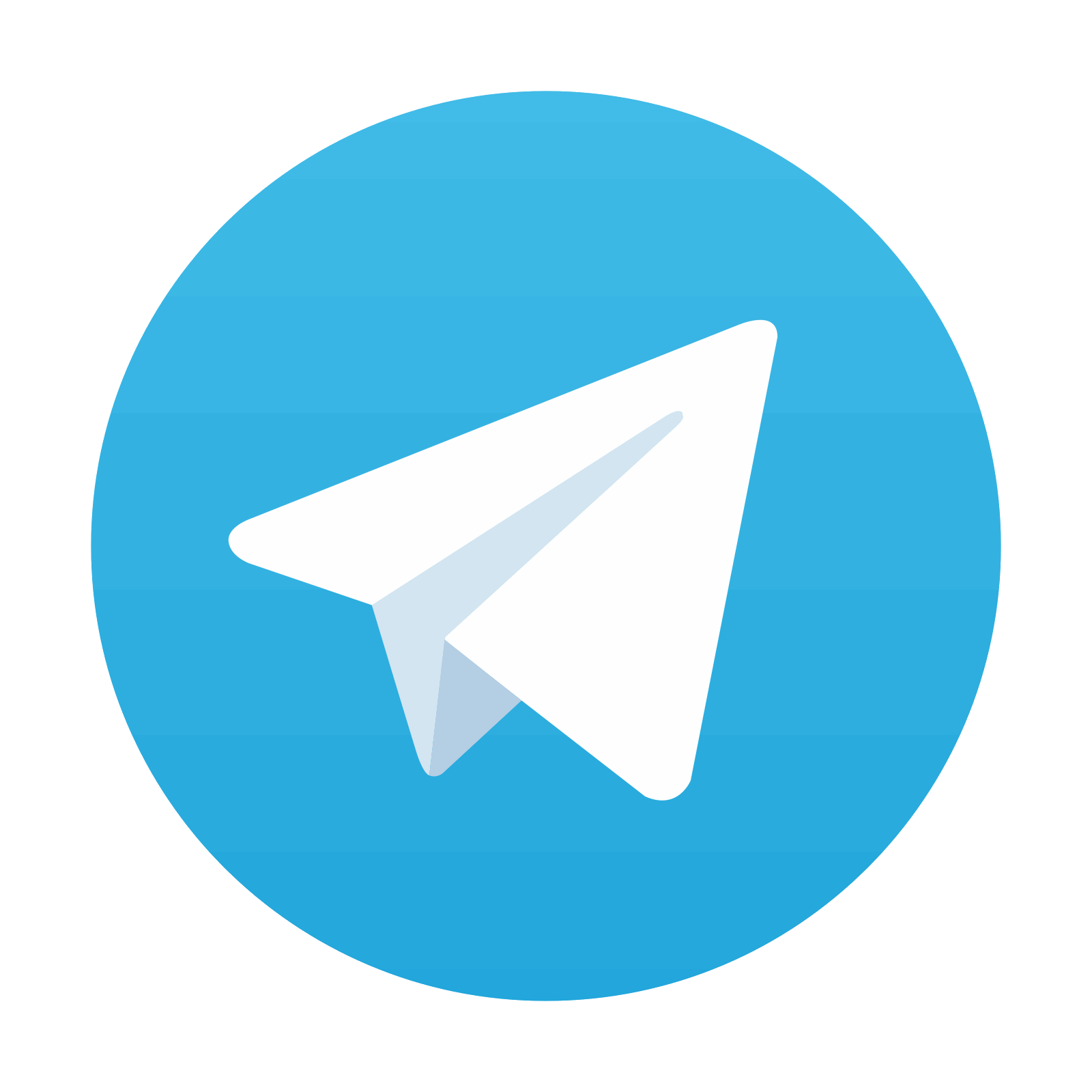
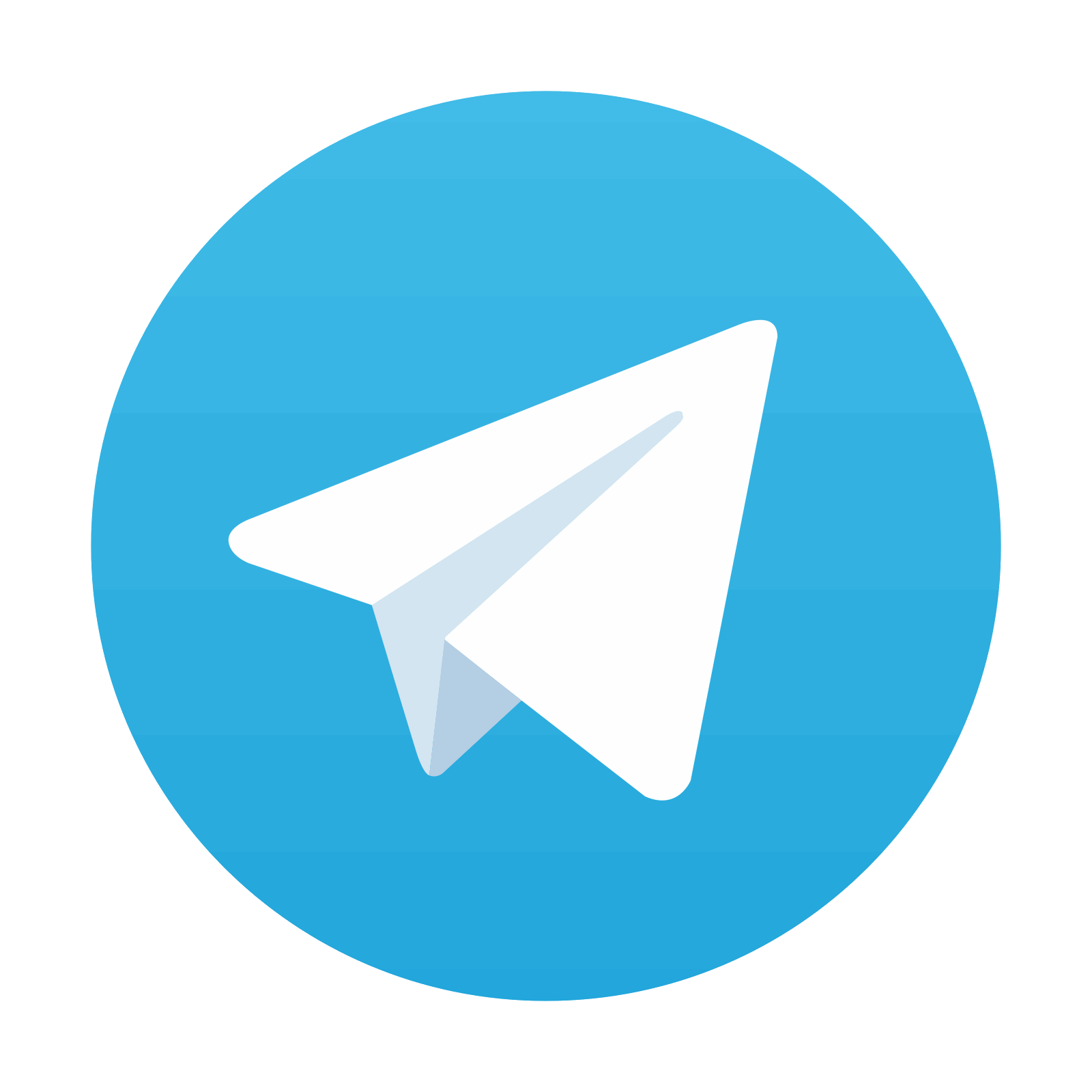
Stay updated, free articles. Join our Telegram channel

Full access? Get Clinical Tree
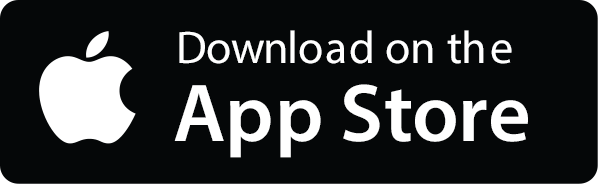
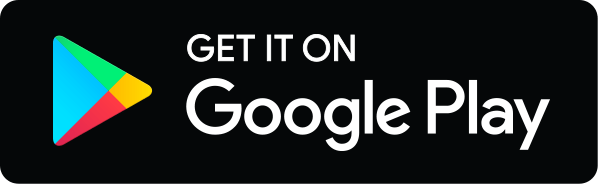