Fig. 2.1
(a) Right stellate ganglion block with needle at C7. (b) Contrast spreads from C5 to T2. (c) Anatomic illustration of the ganglion stellatum (aka cervicothoracic ganglion) (Fluoroscopic images courtesy of Lawrence Poree, MD Ph.D. Illustration courtesy of Rogier Trompert Medical Art. http://www.medical-art.nl; reprinted with permission from van Eijs et al. [5])

Fig. 2.2
Lumbar sympathetic block. (a) Needle placed on the anterolateral surface of the L3 vertebral body. (b) Contrast spreads from L2 to L4 (Fluoroscopic images courtesy of Lawrence Poree, M.D., Ph.D.)

Fig. 2.3
15-mm active tip R-F (Racz-Finch) curved blunt needle for lesioning the splanchnics at T11–T12 placed over the splanchnic nerve dissected on a cadaver (With permission from Raj et al. [7])
Similarly, local anesthetic blocks and radiofrequency lesions of the lower portion of the sympathetic chain is targeted to treat pelvic and perineal pain. For bladder and uterine pain, the superior hypogastric plexus block is employed, whereas for perineal, rectal, and vaginal pain, the ganglion of impar is the target. The superior hypogastric plexus is located on the anterior lateral border of the lower third of the L5 vertebral body and accessed via an oblique fluoroscopic view of the anterior lateral surface of the L5 vertebra or an L5–S1 transdiscal approach [9, 10]. The ganglion of impar is accessed by passing a needle through the sacrococcygeal ligament [11, 12]. Neurolysis of these structures with alcohol or phenol is typically reserved for those with cancer pain; however, botulinum toxin has emerged as a novel tool to aid in providing sympathetic neurolysis beyond the duration of local anesthetic but without the long-term sequel of alcohol or phenol [13, 14].
In addition to the sympathetic chain, another anterior column target for neurolysis is the ramus communicans (Fig. 2.4) [15, 16]. These nerves contribute to nociceptive innervation of the intervertebral disc. Radiofrequency ablation of these nerves at two adjacent levels was first reported to provide pain relief in patients with single level of discogenic pain over 20 years ago, but only one randomized clinical trial has been published on the procedure in that time period [17]. These nerves can be accessed via a 20° oblique fluoroscopic view with a 2-gauge spinal or RF needle advanced to the vertebral body just anterior to the posterior edge. The proper location is identified when sensory stimulation produces a sensation in the back at less than 1.5 V and motor stimulation at twice the sensory stimulation fails to cause contractions of the leg muscles. Once the proper location is identified, a radiofrequency lesion is made at 80 °C for 60 s. One randomized clinical trial compared radiofrequency lesioning of the ramus communicans with a sham treatment. The RF-treated group had significantly lower VAS scores and improved SF-36 scores as compared to the sham-treated group 4 months after treatment [18]. Although there are few studies and only one RCT, the quality of evidence supporting this procedure secured it a level 2B+ positive recommendation using a modified grading system [19, 20]. This procedure is also reportedly effective in the treatment of pain due to vertebral fractures as the ramus communicans also innervates the vertebral bodies. However, further studies are needed to make this a recommended procedure [21].


Fig. 2.4
Illustration of spinal innervation and targets for neural blockade/neurolysis including the sympathetic ganglia, ramus communicans, and ramus medialis (facet nerve) (Illustration courtesy of Rogier Trompert Medical Art. http://www.medical-art.nl. Reprinted with permission from Kallewaard et al. [20])
In the posterior column, the most common targets for paraspinal neurolysis are the medial branches of the spinal posterior rami (aka ramus medialis or facet nerves). These nerves branch off the spinal nerves as they exit the intervertebral foramen to innervate the facet joints (aka zygapophyseal joint). With aging and injury, the facet joint may become sclerotic and hypertrophied and contribute to chronic back pain. Denervating the joint by ablating the medial branch nerves relieves the pain and improves range of motion. The primary target for denervation is the medial branch nerve as it passes over the junction of the transverse process and pedicle in the lumbar spine and in the middle of the facet pillar in the cervical spine [22, 23]. Pain relief after a local anesthetic blockade of these nerves is the diagnostic criteria used to determine which spinal segments are contributing to a patient’s back pain. Two of these nerves are lesioned for each painful facet joint as each joint is innervated by two separate medial branches. Radiofrequency neurolysis at 80° for 90 s is the most common technique for neurolysis although cryoneurolysis is also effective. Two recent analyses of the available literature using the Cochrane Musculoskeletal Review Group criteria for interventional techniques for randomized trials and the Agency for Healthcare Research and Quality (AHRQ) criteria for observational studies evaluated the efficacy of radiofreqency neurotomies of the medial branch nerves to treat facet joint pain and found that the available evidence supported recommending this procedure for the treatment of lumbar and cervical facet joint pain. This recommendation is based on quality of evidence reaching a level II-1 or II-2 when utilizing the grading criteria developed by the US Preventive Services Task Force [24, 25].
Percutaneous facet fusion, a new interventional pain management procedure, has recently been introduced as another technique to address facet joint pain. This fluoroscopically guided technique identifies the facet joint in an oblique view, and using a percutaneous portal system, a drill is advanced to the facet joint. A hole is made large enough to insert an 8-mm bone dowel into the joint which is allowed to fuse over the course of 6 weeks. This technique presumes that fusing the facet joint will relieve facet joint pain, but more clinical trials are needed to fully evaluate the effectiveness of this procedure as a stand-alone procedure for facet pain [26].
Spinal Bone and Connective Tissue Targets for Interventional Pain Management
As patients age, the bone and connective tissue components of the spine are subject to a wide array of degenerative processes that contribute to chronic pain, including but not limited to, vertebral fractures, disc herniations and ruptures, and hypertrophy and sclerosis of facets joints and ligamentum flavum. In the past 10–20 years, various minimally invasive image-guided interventional procedures have been developed to address each of these conditions with varying degrees of success.
Vertebral fractures, a condition common to patients with osteoporosis, can cause both acute and chronic pain. Two fluoroscopically driven procedures have emerged to address this condition, vertebroplasty and kyphoplasty. These vertebral augmentation procedures involve fluoroscopic placement of a needle into the fractured vertebral body and introduction of bone cement in an effort to stabilize the vertebral fracture and regain vertebral height and reduce pain [27]. A systematic review of the available studies from 1980 to 2008 graded the level of evidence using the North American Spine Society guidelines and concluded that there was good evidence to recommend vertebral augmentation in the treatment of vertebral fractures, although only one of the 74 studies was a randomized clinical trial. Subsequently, four additional randomized clinical trials were published that offered conflicting recommendations, two supporting and two not supporting vertebral augmentation for vertebral fractures. The two in support were both open-label trials randomized to kyphoplasty versus medical management in one study [28] and vertebroplasty versus medical management in the other [29] with both having an inclusion criteria of edema noted on MRI. Each study reported significant decreases in VAS scores 1 month posttreatment in the augmented groups versus the medical management groups. Less of a difference was noted at the 1-year point, presumably due to fracture healing. In the kyphoplasty study, quality of life, mobility, and function also showed greater improvement in the surgical versus nonsurgical group. The two studies which did not support vertebral augmentation for vertebral fractures were sham versus vertebroplasty. All patients had radiographic evidence of vertebral fractures and back pain for less than a year, but not all had MRI evidence of edema [30, 31]. Each study reported trends of pain improvement in the vertebroplasty group at the 1-month time point that did not reach statistical significance. As each group continued to improve, there was no discernable difference between them in pain, physical functioning, or disability scores. The authors concluded that there was no significant difference between patients treated with vertebroplasty or a sham procedure. To help resolve these conflicting results, a more rigorous sham-controlled study was designed to include MRI evaluations by two independent radiologists, outcome measurements at 1 day, 1 week, 1,3,6, and12 months after treatment to include VAS, disability, and quality of life scores [32]. The results of this study are pending.
The intervertebral disc is another source of chronic spinal pain targeted by interventional procedures [33]. Derangements of the intervertebral disc can become a source of both acute and chronic back pain and is estimated to constitute up to 45 % of all cases of low back pain [19].
Herniated disc or extruded disc fragments can create pain as a result of a mass effect on neural structures including the spinal cord and exiting nerve roots. In addition, annular tears can allow leakage of the acidic nucleus pulposus leading to neural irritation of the sinuvertebral nerves that innervate the outer annulus as well as spinal nerves if the nucleus pulposus extends beyond the borders of the disc (Fig. 2.4). Diagnosis of this discogenic pain is most often determined by provocative discograms whereby 1–2 ml of contrast is injected into the disc and observed to reproduce concordant pain. The structural integrity of the disc is also evaluated by measuring intradiscal pressure to see if and at what pressure contrast may leak outside the normal boundaries of the nucleus pulposus up to a maximum of 100 psi, the normal pressure of a lumbar disc in the seated position (Fig. 2.5) [15, 19, 34]. Early interventional procedures attempted to treat discogenic pain with intradiscal injections of chymopapain, but anaphylaxis and clinical benefit less than that obtained with surgical discectomy lead to the abandonment of this chemonucleolysis technique [35]. In the intervening 20 years, a number of intradiscal procedures have been introduced utilizing a variety of lesioning, injection, and decompressive technologies, including intradiscal electrothermal therapy (IDET), annuloplasty and other radiofrequency lesioning techniques, injection of corticosteroids, ozone, hypertonic dextrose, and methylene blue, as well as nucleoplasty and other percutaneous disc decompression techniques [36–38]. A recent multicenter analytical review of the available studies of these procedures made the following recommendation [19]: “Intradiscal corticosteroid injections and RF treatment of the discus are not advised for patients with discogenic low back pain. The current body of evidence does not provide sufficient proof to recommend intradiscal treatments, such as IDET and biacuplasty for chronic, nonspecific low back complaints originating from the discus intervertebralis. We are also of the opinion that at this time, the only place for intradiscal treatments for chronic low back pain is in a research setting. RF treatment of the ramus communicans is recommended.” (See section above on paraspinal targets for a review of RF lesioning of the ramus communicans.) The authors went on to conclude “…provocative discography remains the gold standard for the determination of the diagnosis of discogenic pain.”


Fig. 2.5
(a) A/P fluoroscopic image of needles placed within lumbar disc. (b) Lateral view with injection of contrast, note posterior leakage of contrast at the L3–4 disc (Fluoroscopic images courtesy of Lawrence Poree, MD Ph.D)
Minimally invasive lumbar decompression (MILD) is another new interventional pain management technique that targets the hypertrophic ligamentum flavum in patients with lumbar spinal stenosis and neurogenic claudication [39]. As patients age, the ligamentum flavum hypertrophies in part due to replacement of the normal elastin with collagen in the posterior fibers of the ligamentum flavum [40]. Mechanical stress of the ligament causes an inflammatory response with infiltration of macrophages and fibroblast that in turn leads to scar formation. In addition, loss of disc height leads to buckling of the ligament and further narrows the spinal canal [41]. In later stages, calcification and ossification of the ligament develops and contributes even further to thickening and inflexibility of the ligamentum flavum [42]. Until recently, this condition was treated initially with epidural steroid injections, and when this therapy no longer provided significant benefit, patients were treated with an open surgical decompression. The MILD procedure, performed with local anesthetic and minimal sedation, uses the placement of epidural contrast and fluoroscopy to outline the anterior border of the ligamentum flavum in a region where ligamentum flavum hypertrophy was identified on MRI images (Fig. 2.6). A small 5.1-mm trocar is advanced to the inferior lamina of interlaminar space to be treated. Removal of the trocar’s stylet leaves a working portal through which instruments are passed and are used to remove osteophytes and the posterior fibers of the hypertrophied ligamentum flavum. Initial clinical trials revealed that this procedure showed statistically and clinically significant reduction of pain and improvement in the mobility as measured by VAS, ZCQ, SF-12v2, and ODI [43]. These improvements persisted at the 1-year follow-up [44]. A multicenter, randomized clinical trial is currently underway to compare the long-term benefits of the MILD procedure compared with epidural steroid treatments.


Fig. 2.6
(a) Axial MRI of lumbar spine showing spinal stenosis secondary to hypertrophy of the ligamentum flavum (LF). (b) Fluoroscopic image in contralateral oblique view showing epidurogram and failure of contrast to flow cephalad. (c) Tissue sculptor used to remove posterior portion of hypertrophic ligamentum flavum. (d) Epidurogram after decompression shows improvement in epidural flow (Images courtesy of Vertos Medical Aliso Viejo, CA)
Intraforaminal Targets for Interventional Pain Management
Of course, the most important interventional pain management target within the vertebral foramen is the dorsal root ganglion (DRG). The primary sensory afferent neurons in the ganglion are the principle link between peripheral nociceptors and the processing centers of the central nervous system. Injury of these nerves is common from mechanical trauma resulting from lateralized herniated disc or spondylolisthesis, chemical irritation from leakage of nucleus pulposus [45–48], and injury caused by infectious agents such as herpes zoster. All of these injuries can initiate a cascade of inflammatory mediators including cytokines that contribute to the development and maintenance of chronic pain [49]. Thus, it comes as no surprise that foraminal injection of glucocorticoids is a common target for interventional pain physicians [50, 51]. A recent analysis of the available literature using the Cochrane Musculoskeletal Review Group criteria for interventional techniques for randomized trials and the criteria developed by the Agency for Healthcare Research and Quality (AHRQ) criteria for observational studies evaluated the efficacy of transforaminal epidural steroid injections and found that the available evidence supported recommending this procedure for the treatment of lumbar radiculitis. The quality of this evidence was ranked utilizing the US Preventive Services Task Force and found to reach a level II-1 for the short term and level II-2 for long-term management of lumbar nerve root and low back pain [52].
More recently, patients with acute lumbosacral radiculopathy due to intervertebral disc herniation have reportedly improved with transforaminal injections of clonidine [53]. The mechanism for this improvement remains uncertain; however, there may be multiple targets for intraforaminal clonidine. Chung and others observed that peripheral nerve injury leads to sympathetic nerve fiber spouting around the DRG, and this observation was hypothesized to contribute to the development of sympathetically mediated neuropathic pain [54]. Thus, clonidine, an alpha-2 agonist with sympatholytic actions on sympathetic nerve endings, may reduce the effects of increased sympathetic innervation of the DRG after nerve injury. Another possibility is via direct anti-inflammatory action. Liu and Eisenach demonstrated decreased hyperexcitability in rodent-injured nerves after clonidine was applied perinurally and attributed this to inhibition of pro-inflammatory cytokines and prostaglandins [55]. A peripheral site of action for the antinociception activity of an alpha-2 agonist was also suggested by Poree et al. in an animal model of neuropathic pain. In this model, the antinociceptive actions of dexmedetomidine, an alpha-2 agonist, was antagonized by prior treatment with a peripherally restricted alpha2AR antagonist that does not cross the blood-brain barrier. The authors suggested the DRG as a possible peripheral site of action for dexmedetomidine after nerve injury [56].
The DRG has also been targeted for electrical and pulsed radiofrequency stimulation. Although high temperature lesioning radiofrequency energy is successfully employed to denervate medial branch nerves, this technique is avoided in the larger mixed nerves as it may lead to deafferentation pain and painful neuromas. Pulsed and low temperature radiofrequency treatments do not cause neural destruction but instead expose the nerves to a high voltage low to moderate temperature environment. In a prospective randomized double-blind study, 67 °C RF was reported to provide long-term relief of cervical brachial pain [57]. A recent retrospective chart review of 50 patients who received pulsed (42 °C) and moderate temperature (56 °C) radiofrequency treatment of the DRG for lumbar radiculitis reported that all patients received at least a 50 % improvement in their pain [58]. Another group reported that when low temperature (42 °C) pulsed RF was used alone, 30 % of the patients received greater than 50 % pain relief [59]. The observation that even low temperature electric fields applied to the DRG could provide long-lasting pain relief has prompted the recent development of an implantable DRG stimulation system to provide a continuous electric field around the DRG [60]. Excellent results from multiple prospective clinical trials have resulted in approval of DRG stimulation for the treatment of chronic pain in Europe and Australia with clinical trials currently underway in the USA (Fig. 2.7).


Fig. 2.7
Stimulating electrodes placed over dorsal root ganglia within the right T8–9, T10–11, and T12–L1 foramen. Stimulating electrodes placed over dorsal root ganglia within the right T8–9, T10–11, and T12–L1 foramen (Courtesy of Eric Grigsby MD, Napa Pain Institute, Napa, CA. and Jeff Kramer, Ph.D. Spinal Modulation, Menlo Park, CA [60]
Intraspinal Targets for Interventional Pain Management
Targeting the intrathecal space with opioids and local anesthetic has been available for cancer pain management since it was first reported in 1899 [61]. However, widespread utilization of intraspinal (epidural and intrathecal) analgesics outside of the operating room was not practical until the advent of long-term catheters and implantable pumps in the 1980s [62]. Dupen epidural catheters had an antimicrobial sleeve located at the skin exit site, thereby reducing the risk of infection and allowing for intraspinal delivery via an external pump for more than a year. While these catheters are no longer commercially available, they have been replaced by long-term epidural catheters attached to subcutaneous ports which provide even greater protection from infection [63]. For even longer-term intrathecal infusions and even greater protection against infection, implantable pumps have emerged as the preferred method for intrathecal delivery in the past 30 years. While these pumps are initially more expensive than externalized systems, they become cost neutral after 3 months and actually provide a cost savings thereafter as compared with externalized pumps [64, 65]. Most of the current systems are computer controlled, and some have the option for patient-controlled activation of programmed bolus doses [66]. The advantage of intrathecal management over systemic administration is one of inhibition of nociceptive transmission at the spinal level and reduced systemic toxicity. In a randomized clinical trial comparing intrathecal drug delivery systems (IDDS) to comprehensive medical management (CMM), cancer patients treated with IDDS had less medication-induced toxicity, greater pain control, and longer survival than did CMM patients [67]. Nonetheless, it is estimated that only 10–20 % of patients with cancer-related pain fail comprehensive medical management using the World Health Organization guidelines and require more advanced pain management interventions such as IDDS [68]. Guidelines on appropriate selection of patients and intrathecal medication admixtures for patients with intractable cancer-related pain has recently been updated and includes the use of medications approved by the FDA for approved IDDS, medications that are by expert consensus, commonly used for IDDS therapy, and medications that are experimental and are recommended only as a means to provide greater analgesia in the final stages of life (Fig. 2.8) [70–72]. The common pharmacological targets for IDDS therapy include the mu-opioid receptors, calcium channels, sodium channels, and α-2 adrenergic receptors. Figure 2.9 shows the presynaptic and postsynaptic location of the receptors in the dorsal horn that forms the pharmacological basis of IDDS therapy, although, only morphine and ziconitide (aka SNX-111), a novel N-type voltage-sensitive calcium channel antagonist, are currently FDA-approved analgesics for IDDS therapy [70, 73–75]. As IDDS therapy gains greater acceptance for the treatment of intractable cancer pain, the appropriate position in a continuum of care for chronic non-cancer pain remains a source of debate. A recent review aimed at addressing this issue systematically evaluated the available literature using the Agency for Healthcare Research and Quality (AHRQ) criteria for observational studies and the Cochrane Musculoskeletal Review Group criteria for randomized trials. The level of evidence was determined using five levels of evidence, ranging from level I to III with three subcategories in level II, based on criteria developed by the US Preventive Services Task Force (USPSTF) [76]. The authors found 20 studies that met both the inclusions and exclusion criteria. Based on their analysis, they concluded that high-quality evidence supported a moderate recommendation for intrathecal infusion systems for cancer-related pain and that moderate quality of evidence supported a limited to moderate recommendation for non-cancer-related pain.


Get Clinical Tree app for offline access

Fig. 2.8
Polyanalgesic algorithm for intrathecal therapy for cancer pain. With permission from Deer et al. [71])

Fig. 2.9
Possible arrangement of pre- and postsynaptic receptors on structures in the dorsal horn of the spinal cord and potential sites of action of opioid and non-opioid spinal analgesics. Presynaptic release of the neurotransmitter glutamate (Glu) results in activation of the postsynaptic α-amino-3-hydroxy-5-methyl-4-isoxazolepropionic acid (AMPA) receptor, which controls a rapid-response sodium (Na + ) channel. Substance P (SP) interacts with the neurokinin (NK-1) receptor and results in activation of second messengers. With prolonged activation, the N-methyl-d-aspartate (NMDA) receptor is primed, Glu activates the receptor, the magnesium (Mg 2+
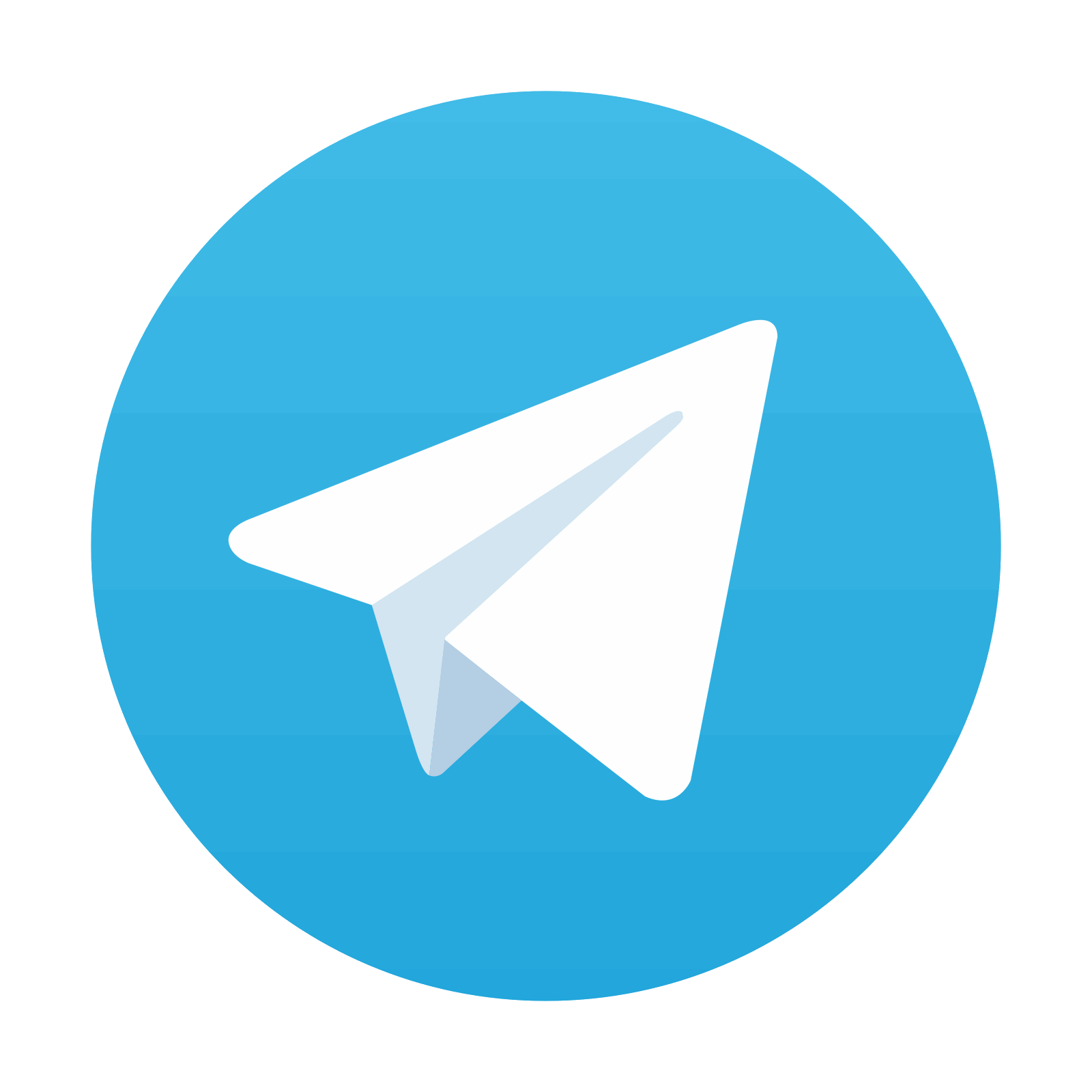
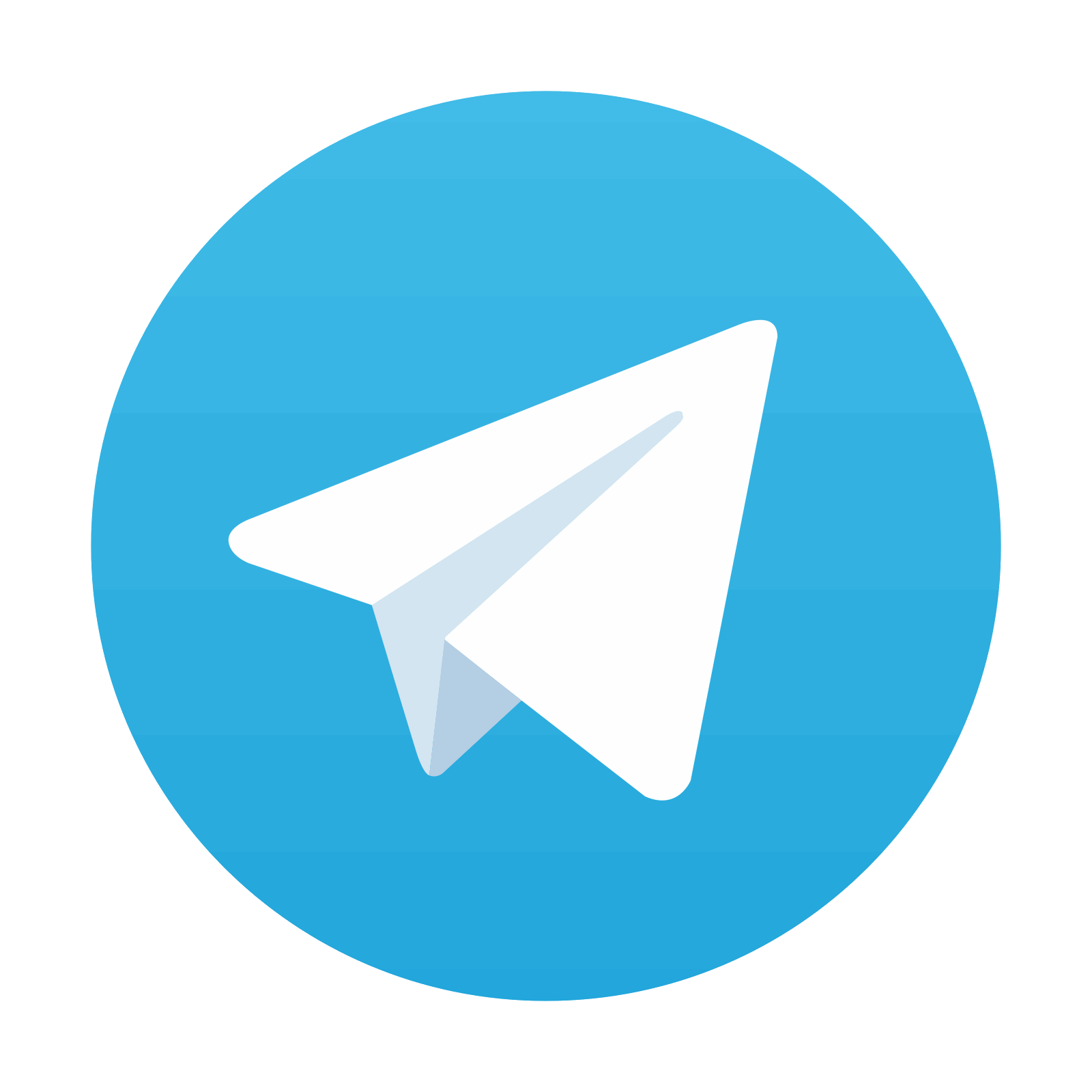
Stay updated, free articles. Join our Telegram channel

Full access? Get Clinical Tree
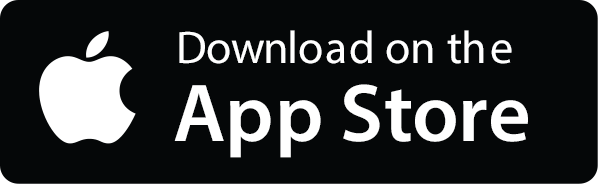
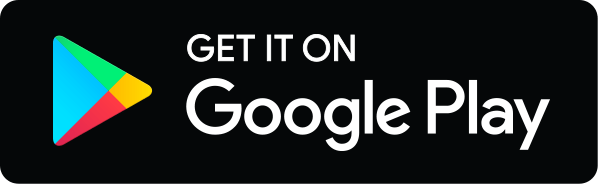
