CHAPTER 3 Standard angiographic and interventional techniques
Vascular access
Anesthesia (online videos 3-1 and 3-2)
A local anesthetic is given at the start of every angiographic or interventional procedure. The preferred agent is 1% or 2% lidocaine (Xylocaine), which inhibits sodium channels involved in the conduction of nerve impulses. An intradermal skin wheal is made with a 25-gauge needle. The deeper subcutaneous tissues are anesthetized with a long 22- or 25-gauge needle. Intravascular injection must be avoided by intermittent aspiration. The pain from lidocaine injection is caused by the low pH of commercially available preparations. Discomfort is eased with “buffered lidocaine,” which is prepared by admixing the drug with sodium bicarbonate (1 mL of 0.9% NaHCO3 solution in 10 mL of 1% lidocaine).1 Patients with a lidocaine allergy may receive an ester- rather than an amine-based anesthetic (e.g., 1% chloroprocaine).2
Retrograde femoral artery catheterization (online video 3-2)
In 1953, Sven Ivar Seldinger first described the method for percutaneous arterial catheterization involving a needle, guidewire, and catheter.3 The common femoral artery (CFA) is the safest and simplest arterial access route because it is large, superficial, usually disease free, and can be compressed against the femoral head to close the puncture. However, this approach should be avoided when the patient has a CFA aneurysm, local infection, overlying bowel, or a fresh incision. Within several weeks after placement, synthetic grafts in the groin also may be accessed safely using a single-wall needle.
When the skin is entered over the bottom of the femoral head and the needle is angled at 45 degrees, the needle usually enters the CFA at its midpoint4 (Fig. 3-1). The inguinal crease is a poor landmark for skin puncture.5 If the puncture is low (into the superficial femoral artery [SFA] or deep femoral artery [DFA]), the risk of thrombosis, pseudoaneurysm, or arteriovenous fistula formation is significantly increased.6,7 If the puncture is too high (into the external iliac artery above the inguinal ligament), the risk of retroperitoneal or intraperitoneal bleeding is increased.8 The bony landmarks for the inguinal ligament—a line running from the anterior superior iliac spine to the pubic tubercle—provide only a rough approximation.9
A small, superficial skin nick is made directly over the arterial pulse. A clamp is used to dissect the subcutaneous tissues. Although the advantages of real-time sonographic guidance for femoral artery puncture are obvious (Fig. 3-2), many practitioners continue to rely on the traditional method of manual palpation of the artery unless entry is difficult. A pulsatile artery may be surprisingly hard to puncture if the skin nick is malpositioned, the artery is unusually mobile, underlying disease exists, or vasospasm follows repeated attempts. In these situations, the operator should consider making a second skin nick directly over the arterial pulse or at a slightly higher location, waiting until a strong pulse has returned, or using the opposite groin. It is sometimes possible to catheterize the abdominal aorta even in the face of iliac artery occlusion if some flow can be detected by ultrasound in the CFA and an angled catheter and hydrophilic guidewire are used to traverse the occlusion.
The course of the artery is palpated while an 18-gauge needle is advanced at a 45-degree angle toward the femoral head (Fig. 3-3). It is safer to use a 21-gauge micropuncture needle set in coagulopathic patients (Fig. 3-4). If double-wall technique is used, the stylet is removed after bone is reached, and additional lidocaine is injected. The hub of the needle is depressed and then slowly withdrawn until pulsatile blood returns. Many interventionalists prefer a single-wall entry into the vessel. However, because single-wall needles have a beveled tip, the tip may be partially subintimal despite brisk pulsatile blood return. Slow return of dark blood usually is a sign of venous entry; the site is then compressed and a more lateral puncture is made.
A 0.035- or 0.038-inch Bentson or floppy J-tipped guidewire is carefully inserted and advanced under fluoroscopy. Resistance to passage usually means that the tip of the needle is partially subintimal, up against the sidewall, or abutting common femoral or iliac artery plaque. The wire should never be forced. A small change in needle position (e.g., medial to lateral, shallow to steep angle, slight withdrawal) usually allows the wire to pass; if not, contrast can be injected to identify the reason for resistance. If the guidewire still cannot be advanced, the needle is removed, compression is applied for a few minutes, and the artery is repunctured. Occasionally, the guidewire enters the deep iliac circumflex artery rather than the external iliac artery (Fig. 3-5). In this case, it is withdrawn and redirected.
After the guidewire is advanced to the abdominal aorta, a vascular sheath (or the bare angiographic catheter) is placed (Fig. 3-6). If the iliac arteries are severely diseased, it may be easier and safer to first place the sheath in the external iliac artery and then negotiate a hydrophilic guidewire into the aorta. Catheter advancement often is difficult in patients with marked obesity, heavily diseased arteries, or a scarred groin. In this case, placement of a stiff or super-stiff guidewire, overdilation of the access site by one French (Fr) size, or use of a stiff, tapered catheter (e.g., Coons dilator) may be helpful.
Antegrade femoral artery catheterization (online video 3-2)
Antegrade (“downhill”) puncture of the CFA is sometimes required for infrainguinal procedures.10 The skin puncture is made over the top of the femoral head to enter the middle of the CFA below the inguinal ligament11 (see Fig. 3-1). In obese patients, it is helpful to tape the pannus onto the abdomen. A steep needle angle (>60 degrees) should be avoided because catheters and sheaths may be difficult to insert or may kink after placement. The guidewire often enters the DFA. Access into the SFA is accomplished in several ways12–14:
Brachial artery catheterization
Brachial artery catheterization is less desirable than CFA access because it is associated with a higher rate of adverse events. The neurologic complications that are the unfortunate hallmark of this technique are related to the particular anatomy of the brachial artery (see later discussion). From axilla to elbow, it runs within the medial brachial fascial compartment, a tight space bound by dense fibrous tissue.15 The radial nerve exits this sheath in the distal axilla, the ulnar nerve in the lower third of the upper arm, and the median nerve continues throughout its course. This route may be necessary or advantageous for:
Decades ago, axillary artery puncture was abandoned for the high left brachial artery to diminish the complications associated with the former route.16,17 Many experienced operators now choose a low (distal) brachial artery site for arterial catheterization.18 Theoretically, right arm access exposes the patient to greater risk of embolic stroke with the catheter crossing all three arch vessels. The right arm is preferred if the brachial systolic blood pressure is significantly lower on the left (>20 mm Hg), suggesting significant left subclavian artery disease.
Alternative arterial access routes
Retrograde popliteal artery access is becoming acceptable for certain femoral artery interventions.19 However, it is premature to claim the safety of this novel route compared with more traditional access sites.
There are few reasons to perform direct translumbar arteriography, one being treatment of endoleak after endovascular graft placement (see Chapter 7). At first glance, the technique would appear unduly risky, but it is notable that generations ago, 5- to 7-Fr catheters were inserted directly into the aorta for diagnostic angiography with surprisingly few bad outcomes.20
Femoral vein catheterization (online video 3-4)
Before performing common femoral vein (CFV) catheterization, any existing lower extremity venous sonograms or computed tomography scans should be reviewed to confirm vessel patency. The CFV usually lies 0.5 to 1.5 cm medial to the CFA. Skin entry is made just medial to the arterial pulse and just below the bottom of the femoral head. In some patients, the vein is slightly medial and deep to the artery.21 A single-wall needle is preferred to avoid unknowingly traversing the artery before entering the vein.
Internal jugular vein catheterization (online video 3-5)
The vessel is entered above the clavicle, always with direct sonographic guidance. With the transducer oriented in a transverse plane, the needle is advanced from a lateral approach or directly superior to the vein (Fig. 3-7). A micropuncture set can be used to minimize trauma to the internal carotid artery if it is accidentally pierced. Entry into the venous system is confirmed by following the course of a guidewire advanced toward the right atrium.
Axillary/subclavian vein catheterization
Subclavian vein access to the central venous system is discouraged for several reasons. Venous stenosis or occlusion is much more frequent after placement of subclavian vein catheters.22 There is also a small risk of pneumothorax that is virtually nonexistent with internal jugular access. Finally, bleeding is more difficult to control if the subclavian artery is accidentally entered or venous access is lost. If this route must be used, puncture should always be made with sonographic guidance. The preferred point of entry is the central axillary vein at the level of the coracoid process. With the ultrasound transducer held in a longitudinal plane, the axillary/subclavian artery is identified first. A micropuncture needle is then advanced into the vein, which is situated just inferior to the artery (see Fig. 18-8).
Arterial closure devices (online video 3-6)
For more than 50 years, manual compression has been the standard approach for obtaining hemostasis of vascular catheterization puncture sites. However, this method requires additional operator time and rather prolonged patient bedrest afterward. Gaining hemostasis in anticoagulated patients or after large arterial sheaths (≥7 Fr) are removed can be problematic. Arterial closure devices are meant to reduce time to ambulation while allowing effective and safe vascular closure, even in the face of anticoagulation.23–27 Three categories of devices are currently in use:
No one device is superior to the others, although patches and collagen-mediated products are not appropriate for larger holes (e.g., greater than 8 to 9 Fr). Device failure or need for conversion to manual compression is uncommon (<15% of cases) and rare for experienced operators. Some of these systems significantly reduce time to hemostasis and time to ambulation, particularly in anticoagulated patients.23,28–33 Overall, the complication rate is comparable to manual compression. Still, routine use of these devices is controversial for several reasons:
Complications
Table 3-1 outlines the most common adverse outcomes from femoral artery catheterization.36–39 Minor bleeding or hematoma formation occurs in less than 10% of simple femoral artery catheterization procedures. Major bleeding requiring transfusion or surgical evacuation is relatively rare (<1%), but more likely when sheath size increases or anticoagulants and fibrinolytic agents are used. Blood may collect in the thigh, groin, retroperitoneum, or, rarely, the peritoneal space. Retroperitoneal hemorrhage should be suspected in a patient with an unexplained drop in hematocrit, hypotension, or flank pain (Fig. 3-9).
Table 3-1 Complications of Femoral Artery Catheterization
Type | Frequency (%) |
---|---|
Minor bleeding or hematoma | 6–10 |
Major hemorrhage requiring therapy | <1 |
Pseudoaneurysm | 1–6 |
Arteriovenous fistula | 0.01 |
Occlusion (thrombosis or dissection) | <1 |
Perforation or extravasation | <1 |
Distal embolization | <0.10 |
With proper technique, catheterization-related pseudoaneurysms are relatively uncommon (about 1% to 6%); arteriovenous fistulas are quite rare39,40 (see Fig. 1-34). Most small (<2 cm) pseudoaneurysms close spontaneously. Large or persistent lesions require treatment (Fig. 3-10, see later discussion). Femoral artery thrombosis or occlusion usually is caused by dissection, spasm, or pericatheter clot (Fig. 3-11). Cholesterol embolization from traumatic disruption of an atherosclerotic plaque is a rare but potentially devastating complication of arteriography41 (see Chapter 2).
Other potential adverse events include nausea and vomiting, vasovagal reactions, and contrast media–related reaction or nephropathy. Cardiac events (e.g., arrhythmias, angina, heart failure) and neurologic events (e.g., seizures, femoral nerve injury, stroke) also can occur during vascular interventions.42
The reported frequency of complications from axillary or brachial artery access ranges from 2% to 24%.16–18,20 In contemporary series, catheterization-related events with mid or low brachial artery puncture are less common but not negligible (0.44% [for diagnostic studies with 4-Fr catheters] to 6.5% [for interventional procedures with larger sheaths and anticoagulants]).18,43 This vessel is more prone to thrombosis or pseudoaneurysm formation than the CFA (see Fig. 9-14). Distal neuropathy is a distinct but uncommon sequela of brachial artery puncture related to the tight anatomic space shared by the artery and several peripheral nerves (see earlier discussion). Thus even small hematomas can cause nerve compression. Sensory or motor neuropathy is reported in about 2% to 7% of patients who undergo this procedure.16–18,43 The deficit is more likely to become permanent if early surgical decompression is not accomplished as soon as the problem is suspected. The other devastating neurologic complication of retrograde brachial artery catheterization is cerebral embolization of pericatheter clot, which has been reported in up to 4% of cases but is much less common in actual practice.17
Treatment of postcatheterization pseudoaneurysms and arteriovenous fistulas
Ultrasound-guided compression repair is effective in many cases of postcatheterization pseudoaneurysms.44–46 In this technique, the ultrasound transducer is used to compress the neck of the pseudoaneurysm while flow is maintained in the SFA (Fig. 3-12). Patients are then kept at bedrest for 4 to 6 hours. Follow-up sonography is required to confirm permanent thrombosis. Pseudoaneurysm closure is successful in about 75% to 85% of cases. However, the method is painful (usually requiring moderate sedation), time-consuming, and sometimes ineffective, particularly in patients receiving anticoagulation.47 Compression repair is not advised when flow in the neck cannot be obliterated or for lesions located above the inguinal ligament.
Ultrasound-guided percutaneous thrombin injection has become the first-line treatment for angiography-related pseudoaneurysms.46–51 Thrombin injection also has been used to treat postcatheterization brachial artery pseudoaneurysms.52 The procedure is quick, relatively painless, and highly effective. After excluding an arteriovenous fistula and using real-time ultrasound guidance, a 22- or 25-gauge needle is inserted into the body of the pseudoaneurysm away from the neck (see Fig. 3-10). Bovine thrombin (1000 units/mL) is injected into the lesion over 5 to 10 seconds. Most pseudoaneurysms require well under 1000 units for complete thrombosis. Clot formation is monitored with color Doppler imaging. The success rate is 90% or greater, even in the face of anticoagulation. Complete closure may be more problematic with complex pseudoaneurysms.48 A failed first attempt should be repeated. However, the patient and operator should be aware that prior exposure to thrombin (topical or otherwise) can lead to antibody formation and the small risk of anaphylactic reaction. Although complications are rare, there are several reports of limb-threatening embolization or downstream thrombosis.53–55 The presence of a wide or short aneurysm neck may predispose to this serious event.
Arteriovenous fistulas are much less common than pseudoaneurysms after femoral artery catheterization (Fig. 3-13 and see Fig. 1-34). Many fistulas close spontaneously. Repair is recommended if they persist for more than 2 months, increase twofold or more in size, or become symptomatic. As an alternative to operation, covered stents have been deployed to close fistulas. However, the published experience is too limited to endorse this approach as a routine measure.56–58 In rare instances, embolization of a long track is feasible (see Fig. 8-53).
Basic angiographic and interventional tools
Catheters and guidewires (online videos 3-1 and 3-7 to 3-9)
The primary characteristics of guidewires are listed in Box 3-1. All wires have a relatively soft, tapered segment of variable length at the working end. Standard guidewires are made of a stainless steel coil wrapped tightly around an inner mandril that narrows at the working end of the wire. A central safety wire filament is incorporated also to prevent complete separation if the wire breaks. Hydrophilic guidewires are extremely useful in diseased or tortuous vessels. Standard guidewire diameters are 0.035 and 0.038 inch. Finer-gauge wires (e.g., 0.014 and 0.018 inch) are available for use with microcatheters or small-caliber needles. Standard guidewire lengths are 145 cm and 175 cm. A long (260 to 300 cm) exchange wire may be needed for selective catheter changes. The more commonly used guidewires are outlined in Table 3-2.
Table 3-2 Commonly Used Guidewires
Type | Function |
---|---|
Standard (0.035- or 0.038-inch) | |
Bentson and floppy J tip wires | Standard access wire |
Newton LT/LLT | Standard working wire |
Hydrophilic wires (e.g., Terumo) | Use in tortuous or diseased vessels |
Extra stiff wires (e.g., Amplatz) | Insertion of larger devices, resistant catheter passage |
Exchange wires (e.g., Rosen) | Exchange of long angiographic catheters or devices or remote distance from access |
Tapered wires (e.g., TAD wire) | Placement of devices into sensitive territories |
Moveable core wires | Variable floppy working segment |
Microwires (0.012- to 0.018-inch) | |
Cope mandril | Standard micropuncture access wire |
Transcend | Floppy, steerable microwire |
Fathom | |
Syncro | Floppy, highly steerable and trackable microwire |
V-18 | Steerable, stiffer microwire |
BMW | Steerable, stiffer microwire |
Platinum plus | Steerable, stiffer microwire |
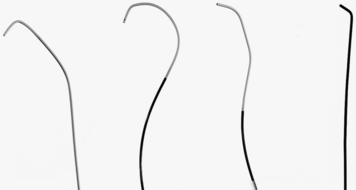
Figure 3-14 Basic straight angiographic catheters. Left to right, spinal, cobra, headhunter, and angled shapes.
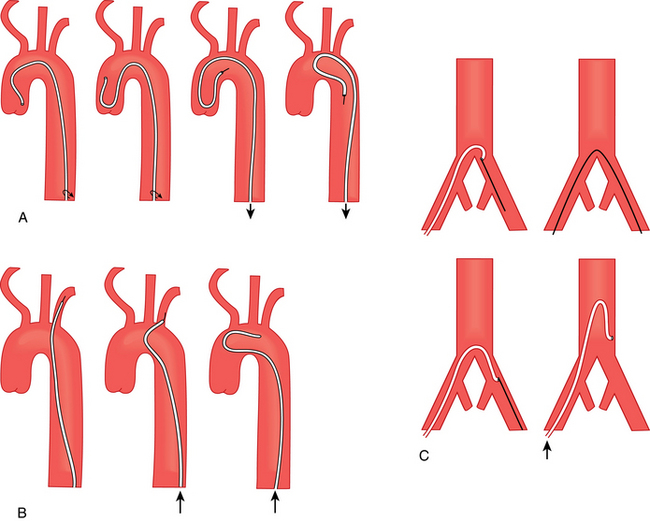
Figure 3-16 Methods for reforming a Simmons catheter.
(Adapted from Kadir S. Diagnostic angiography. Philadelphia: WB Saunders; 1986. p. 74.)
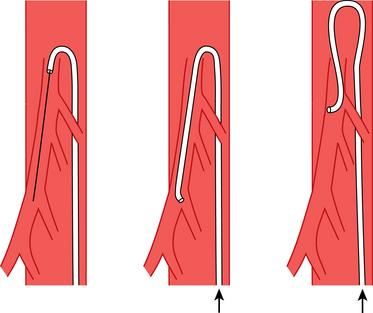
Figure 3-17 Method for forming a Waltman loop.
(From Kadir S. The loop catheter technic. Med Radiogr Photog 1981;57:22. Reprinted courtesy of Eastman Kodak Company.)
Pressure measurements
Intravascular pressure monitoring is primarily used to determine the hemodynamic significance of stenoses, assess the results of revascularization procedures, and diagnose pulmonary artery or portal venous hypertension. A pressure gradient is far more accurate than multiple angiographic images for proving the significance of a vascular stenosis.61 Hemodynamic measurements must be obtained with meticulous attention to detail to minimize artifacts.
The pressure gradient across a stenosis in a tube with flowing fluid is defined by Poiseuille’s law:
In the equation, ΔP = pressure gradient, Q = blood flow, L = length of the stenosis, η = blood viscosity, and r = radius. In medium-sized arteries, blood flow is unchanged until the luminal diameter is reduced by 50%, which corresponds to a cross-sectional area reduction of 75% (Fig. 3-19). Blood flow falls precipitously as the diameter stenosis approaches 75% (about a 95% reduction in cross-sectional area). The relationship between flow reduction and luminal diameter becomes more complex with diffuse disease or tandem lesions. Pressure gradients are affected by blood flow. For example, as the peripheral arterial resistance in the legs drops with exercise, the magnitude (and therefore the clinical significance) of proximal pressure gradients increases.
The thresholds used to define a significant arterial pressure gradient are controversial. Resting systolic and mean gradients from 5 to 34 mm Hg have been suggested.62–64 Absolute or relative gradients after flow augmentation (intraarterial injection of a vasodilator) are favored by some experts. As a general rule, a resting systolic gradient of 10 mm Hg or greater is considered significant in the arterial system. In the central veins, a focal gradient of 3 to 6 mm Hg or greater can be flow-limiting.
Pressure gradients are most accurate when simultaneous measurements are obtained from endhole catheters on either side of a stenosis. However, often it is more practical to use a single catheter to measure a “pullback pressure” across the lesion. With this method, however, the gradient may be spuriously elevated if the diameters of the catheter and vessel are similar (e.g., arteries ≤5 mm in diameter). A useful tool for determining hemodynamic significance of lesions in medium- and small-caliber arteries is a pressure guidewire (e.g., PrimeWire Prestige).65,66
Contrast agents
Iodinated contrast agents can produce numerous systemic effects after intravascular administration67 (Box 3-2). The severity of these alterations depends largely on the osmolality of the preparation. At similar iodine concentrations, low osmolar contrast materials (LOCMs; ionic dimers and nonionic agents) have a significantly lower osmolality (600 to 800 mOsm/kg) than high osmolar contrast material (HOCMs; ionic monomers) with osmolality at 1400 to 2000 mOsm/kg. Iodixanol (Visipaque) is the only isosmolar agent (290 mOsm/kg) currently available in the United States.
In most centers, nonionic agents are chosen for all intravascular applications. Minor side effects, such as nausea, vomiting, and local pain, are much less common with these drugs.67 The overall incidence of adverse events with LOCM is less than 1%. The frequency of moderate to severe reactions is estimated at about 0.1% to 0.2% for HOCM and 0.01% to 0.02% for LOCM. The frequency of fatal reactions is less than 0.005% and not significantly different between the two classes of material.68 There are only small differences in imaging quality among the various agents at the same iodine concentration,69,70 The evidence is strong but not indisputable that contrast nephropathy is less likely in at-risk patients with use of iodixanol.71–75 At centers in which cost issues are of particular concern, an argument can be made for selective use of nonionic material.
Carbon dioxide has been used extensively as a contrast agent for digital imaging in a variety of arterial and venous beds76–80 (Fig. 3-20). The gas rapidly dissolves in blood and is eliminated from the lung less than 30 seconds after injection. There is no risk of allergic reaction or nephrotoxicity. An airtight system of reservoir bag, tubing, and syringes is constructed to purge a delivery syringe of room air and substitute instrument grade CO2 (Online Video 3-10). For abdominal aortography or inferior venacavography, a 60-mL syringe is required. The catheter is then primed with the gas before rapid injection. Some patients experience discomfort with injection. The quality of images is generally inferior to those obtained with iodinated contrast. In addition, complications can arise from gas trapping and “vapor lock,” especially in the pulmonary artery, abdominal aortic aneurysms, and the inferior mesenteric artery. The agent cannot be used in arteries above the diaphragm because of the risk of intracerebral embolization.
Gadolinium-based contrast materials can be used in individuals with a history of anaphylactic reaction to iodinated agents and normal renal function. However, they are not safe for intravascular use in patients with acute renal failure, chronic kidney disease (eGFR [estimated glomerular filtration rate] <30 mL/min), or dialysis dependence. In these populations, there is clear dose-related causation between some of these drugs and the highly debilitating disorder of nephrogenic systemic fibrosis81–83 (NSF, see Chapter 2).
Pharmacologic adjuncts
Antiplatelet agents
Aspirin (acetylsalicylic acid, ASA) is a moderate inhibitor of platelet aggregation. It works by irreversibly inactivating cyclooxygenase (COX), a critical enzyme in the production of a key enzyme (thromboxane A2) required for platelet function.84,85 The drug is rapidly absorbed from the stomach; platelet function is inhibited within 1 hour of ingestion and continues for the lifetimes of existing platelets (about 7 to 10 days). Aspirin prolongs the bleeding time without significantly affecting other coagulation parameters. Patients often are maintained on a daily dose of 325 mg for at least several months after recanalization procedures.
Thienopyridines are more potent oral antiplatelet agents that irreversibly inhibit binding of adenosine diphosphate (ADP) to platelet receptors, thus preventing platelet-fibrinogen binding and αIIBβ3 integrin (glycoprotein [GP] IIb/IIIa)–mediated platelet activation and aggregation.86,87 The first-generation agent ticlopidine (Ticlid) is rarely prescribed because of certain relative drawbacks. The second-generation drug clopidogrel (Plavix) is in widespread use. The standard loading dose is 300 mg orally, with typical daily dosage of 75 mg. The new third-generation agent prasugrel may be useful in patients who are “nonresponders” to clopidogrel.86 Combination therapy (aspirin + clopidogrel) is favored in many situations for patients with coronary artery disease. However, current recommendations favor monotherapy for primary prevention of cardiovascular events in the subset of individuals with peripheral arterial disease.88,89 For interventionalists, clopidogrel (alone or in combination with aspirin) may be useful in some patients following arterial recanalization procedures. These agents also show promise in preventing restenosis after angioplasty, stent insertion, or bypass graft placement. The major downside to thienopyridines is bleeding. In patients requiring certain invasive procedures, clopidogrel must be withheld for 7 to 10 days to reverse the bleeding tendency.
Cilostazol (Pletal) is a phosphodiesterase III inhibitor that has antiplatelet, antithrombotic, smooth muscle antiproliferative, and vasodilatory effects.90–92 There is abundant evidence that long-term therapy (50 to 100 mg orally twice daily) increases exercise ability and overall quality of life in patients with intermittent claudication. There is also growing support for its additive benefit in preventing restenosis after some endovascular recanalization procedures.93 Significant drug interactions can occur with certain cytochrome P450 inhibitors (e.g., diltiazem, erythromycin, and omeprazole).
αIIBβ3 Integrin (GP IIb/IIIa) receptor inhibitors are a class of potent cell receptor antagonists that act on the final common pathway to platelet aggregation. Although interplatelet binding is inhibited, platelet attachment to subendothelial elements is maintained. Although these parenteral drugs have great potential for enhancing revascularization in acute coronary syndromes, the experience in peripheral arterial disease has been somewhat disappointing.94–96 As such, these agents should not be used routinely but instead should be reserved for selected cases, such as slow response to thrombolytic agents, thrombophilic states, need for rapid revascularization, or infrageniculate interventions (Table 3-3). It is important to carefully monitor platelet levels, which can fall precipitously during treatment.
Antithrombin agents
Heparin is a polyanionic protein that binds with antithrombin (AT), among other plasma proteins and cells.97 The resulting complex inhibits clot formation by inactivating thrombin and factor Xa. This effect is dependent on a specific pentasaccharide sequence present on unfractionated heparin and other synthetic drugs (see later discussion). Because thrombin is the critical enzyme in clot formation, heparin is a potent antithrombotic agent. The drug is cleared from the body in two phases. Rapid initial clearance by fairly indiscriminate binding to plasma proteins and endothelial cells is followed by slower clearance by the kidneys. The biologic half-life varies widely among individuals, but it is roughly 1 hour at typical therapeutic doses (5000 units IV bolus followed by 500 to 1500 units/hr infusion). Protamine, a cationic protein derived from salmon sperm, completely reverses the anticoagulant effect (see Chapter 2).
Because heparin pharmacokinetics are unpredictable, its effect must be measured. During vascular procedures, the antithrombotic response can be followed with the activated clotting time (ACT), which reflects whole blood clotting.98 Normal and therapeutic ranges are specific to each manufacturer’s device. The activated partial thromboplastin time (PTT) is used to monitor long-term anticoagulation. The therapeutic range is 1.5 to 3.5 times the control value.99 One protocol for adjusting heparin doses based on the PTT obtained every 4 to 6 hours was recently proposed by a panel of experts.100 Patients who are extremely resistant to heparin may require titration by direct heparin assay or a switch to a low molecular weight heparin (LMWH) agent (see later discussion).
The major complications of heparin therapy are bleeding, heparin-induced thrombocytopenia (HIT) (see Chapter 1), and osteopenia (with long-term use). The risk of bleeding is a function of drug dose, concomitant use of thrombolytic agents, recent surgery or trauma, baseline coagulation status, kidney function, and age. To screen for HIT, platelet levels should be monitored two or three times a week.
LMWH has more predictable and persistent anticoagulant activity than unfractionated heparin.97,101,102 This class of drugs includes enoxaparin (Lovenox), dalteparin (Fragmin), reviparin, and tinzaparin (Innohep). The primary mechanism of action is inhibition of factor Xa and thrombin mediated through antithrombin. Unlike unfractionated heparin, LMWH exhibits almost no indiscriminate cellular or protein binding. As such, clearance is dose-independent, and the half-life (about 4 hours) is much longer. The dose must be reduced in patients with renal disease; the drug is avoided altogether in severe renal insufficiency (eGFR <30 mL/min).
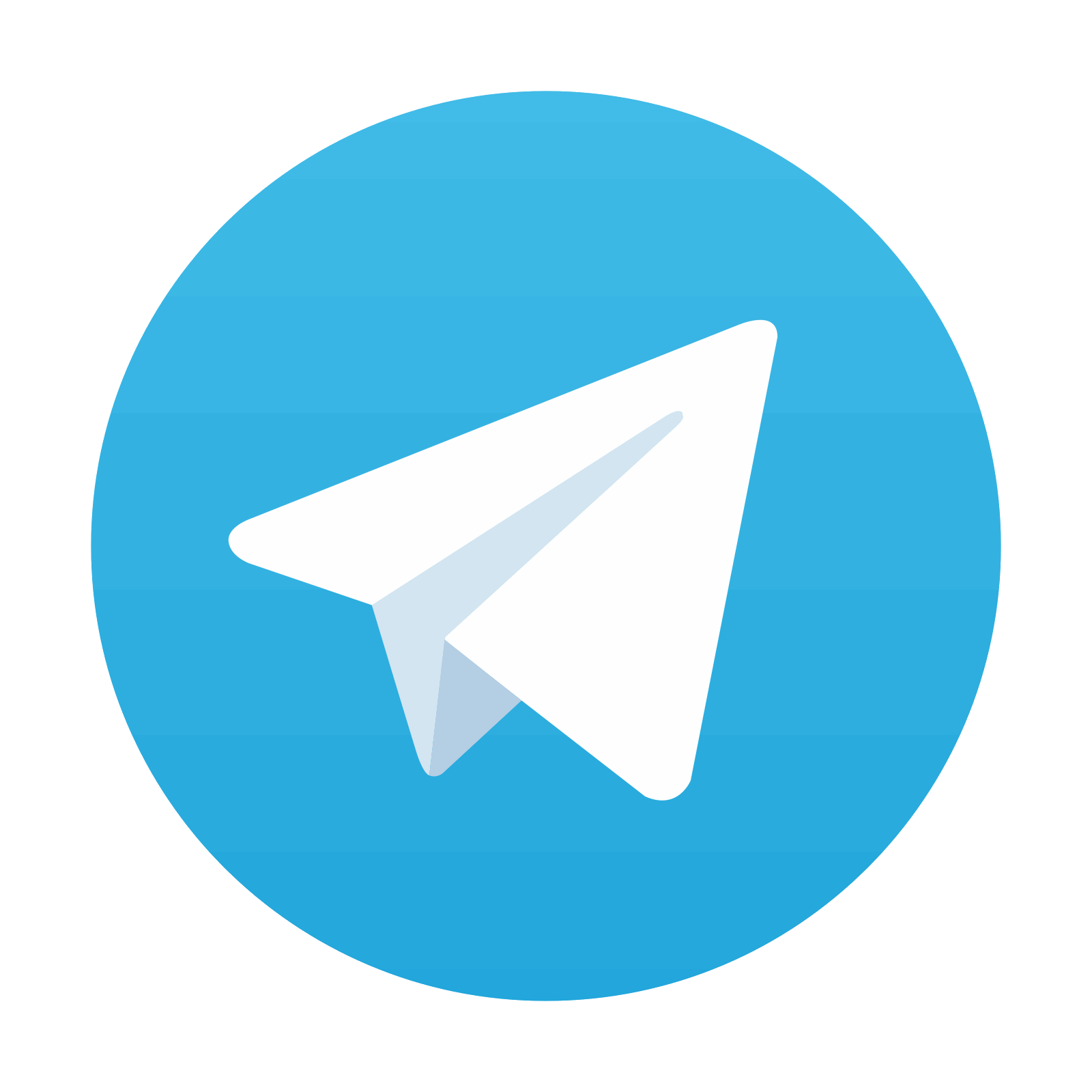
Stay updated, free articles. Join our Telegram channel

Full access? Get Clinical Tree
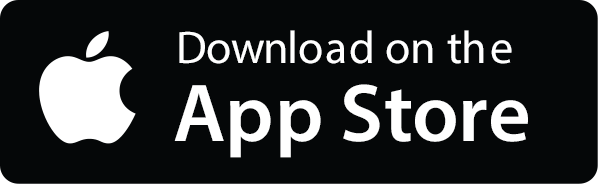
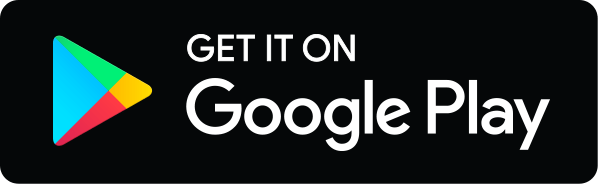