Fig. 1
Biocontinuum of adverse and late effects of the skin surface (with permission from Rubin and Casarett 1968)
More commonly, the skin is exposed to therapeutic radiation incidentally, during treatment of relatively superficial but noncutaneous malignancies such as breast cancer, or head and neck cancer. One of the most significant advances in radiation therapy technology over the past several decades is the advent of high-energy treatment units (megavoltage linear accelerators), which provide treatment that is more skin sparing than their predecessor, lower-energy, treatment machines. Depending on the specific energy used and the depth from the skin of the target lesion, the radiation dose at the skin surface might range from 5 (e.g., for a prostate treatment) to 90 % or more (e.g., for a breast or head and neck treatment).
The side effects of therapeutic radiation treatment are typically divided into acute and late effects. Acute effects are those that occur during fractionated treatment, or within a relatively arbitrary number of days of cessation of treatment (i.e., within 90 days). These symptoms are mainly the result of permeability changes in the tissue stroma (i.e., inflammation) and depletion of rapidly dividing tissue stem cells. These occur, progress, and regress in a relatively well-described series of events. Both the timing and severity are related to the volume of skin exposed, the radiation dose, and the fractionation schedule. The severity may be modified by treatment technique (e.g., the use of bolus materials), patient factors, and concomitant radiosensitizers.
Late effects are usually defined, solely for the purpose of evaluating treatment toxicity, as those which occur more than 90 days after radiation treatment. In truth there are signs and symptoms which may develop months to years later. These are often related to long-term loss of stromal microvasculature, and fibrotic replacement of normal tissue architecture. They are more loosely correlated to the dose and volume of radiation, but are more likely to be affected by clinicopathologic factors that affect local circulation and inflammatory response, as well as by genetic predisposition.
Treatment for acute radiation toxicity is generally supportive, addressing the symptoms and attempting to ameliorate the loss of normal skin barrier function. Late radiation toxicity is more effectively prevented than treated, although there is now accumulating evidence that late radiation changes can be ameliorated with appropriate medical and management. The Biocontinuum of adverse early and late effects are shown in Fig. 1.
2 Anatomy and Histology
2.1 Anatomy
The skin can be divided into its surface sectors and the lymph node region that drains a sector (Figs. 2, 3a, b).
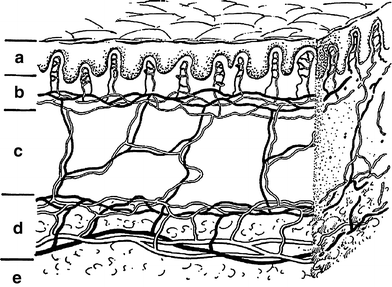
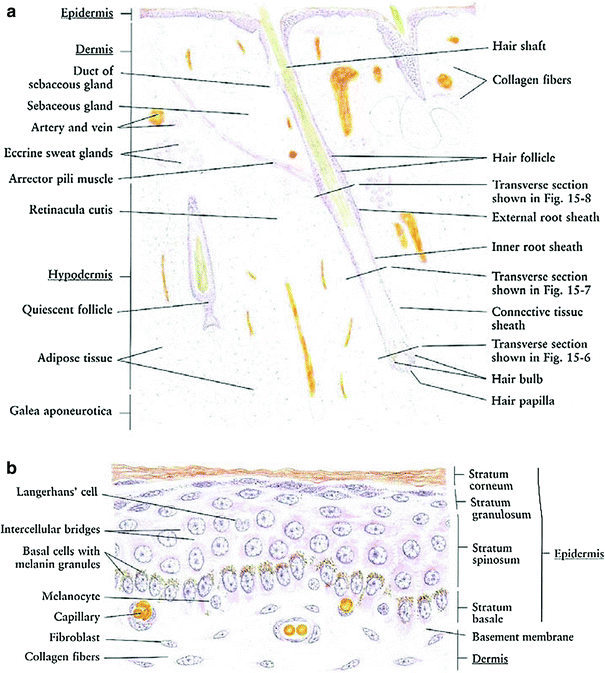
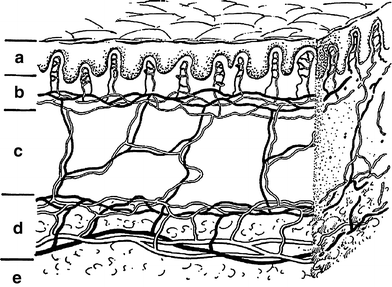
Fig. 2
Schematic drawing of skin showing the epidermal, dermal, and subcutaneous shells while emphasizing the critical microvascular components. The part labeled a is the epidermis (epidermal shell). b is the papillary dermis containing microvessel tufts arising from the papillary plexus. c is the rete dermis with arcuate vessels connecting the subdermal with papillary plexus. d is the dermal subcutaneous junction with the dermal plexus. e is the subcutaneous layer (From Archambeau et al. 1995, IJROBP LENT SOMA)
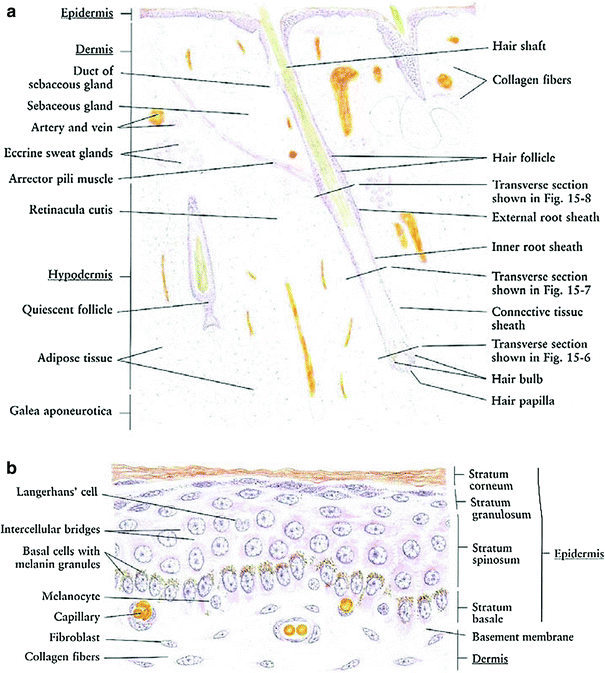
Fig. 3
a Scalp low magnification longitudinal section. b Thin skin: abdomen. High magnification (with permission from Zhang 1999)
The head and neck includes the face and scalp. The vast majority of cancers arise on the skin of the face. Therefore, it is the face and scalp that demand careful attention clinically because of the complex functions, cosmesis, and special senses. All need to be preserved when resecting the cancer. The lymph node drainage of the integumentary surface differs from the upper aerorespiratory and digestive passages.
Anterior chest wall from the clavicles to the navel in males tends to be hirsute. Lesions on the skin of the anterior thoracic wall drain to the anterior axillary nodes.
Posterior chest wall to the same level tends to be less hirsute, is exposed more often to the sun, and subject to forming cancers. The regional nodes are along the posterior wall of the axilla although all axillary nodes are at risk.
Upper extremity is an infrequent sector involved with skin cancer, but can be a site for burn and chronic inflammation. It is notorious for radiation-induced cancer in dentists who finger-held dental films during their practice. Endless resections occur with loss of fingers, then the hand, then the forearm. Involvement of epitrochlear node, then axillary nodes invariably leads to demise from pulmonary metastases.
Anterior abdominal wall drains into the femoral and inguinal nodes but this sector of skin is rarely involved with skin cancers.
Posterior abdominal wall or skin of the lower back is an infrequent site of malignancy and also drains to femoral and inguinal nodes anteriorly.
Lower extremity is not a common site for skin cancers. Burns or chronic inflammation may cause lesions to evolve from hyperplasia to dysplasia and on to neoplasia. Popliteal nodes drain the foot and leg and ultimately drain into superficial femoral lymph nodes, which also drain the thigh.
Skin cancers are predominantly located on and in the face. To fully appreciate the anatomy, it is important to be aware of the surrounding structures and especially underlying muscle and nerves. As the cancer advances and invades, the reconstruction is more than cosmesis. A particularly troublesome area is over the parotid gland because perineural invasion of the widely branching facial nerve is a major concern.
N-oncoanatomy of the skin surfaces emphasizes the anterior location for most if not all lymph node stations.
The skin lymphatics of the face are different from head and neck cancers, since the drain to a ring of nodes that hang like a necklace from the occiput to below the ear, the parotid and anteriorly to the submaxillary and submandibular region. Once the cancer invades and advances involving deeper underlying structures then the deeper cervical nodes can become at risk for involvement.
There is a rich network of venous channels beneath all skin surfaces that allows for venous hematogenous spread once the dermal and hypodermal layers are penetrated by invading cancers (Fig. 2). These venous collateral channels and plexus are rich and appear once obstruction occurs. Metastatic spread via superficial and deep jugular vein is to lung predominantly. With extensive recurrent and destructive basal cancers which seldom metastasize early, aspiration into lung has been postulated but is an unlikely mechanism. Squamous cell cancers as they invade lymph nodes disseminate via focal veins. Merkel cell cancers are more virulent and are more prone to become metastatic (Rubin and Hansen 2008).
2.1.1 Skin Functional Unit
The smallest unit of skin that retains all the characteristics of skin (a unit of skin structure) consists of a microvessel with associated epidermis and dermis. This element of skin is referred to as a “skin functional unit” (FUs). The FUs is a core about 30 μm in diameter and 350 μm in length. There are an estimated 30,000 FUss per cm2 in swine, with an associated 135 basal cells overlying a microvessel tuft 80–150 μm long. The skin configuration model emphasizes that the dose response of the FUs defines the dose response of the skin.
2.2 Histology
The skin is a multifunctional organ composed of three layers: the epidermis, dermis, and underlying hypodermis (Fig. 3a). The skin provides protective barrier function, and serves as an important role in hydration, temperature balance, and immunity (Fig. 3a).
The epidermis is the outer layer of the skin, composed of stratified squamous epithelium (Fig. 3b). It varies in thickness from 0.05 (on the eyelids) to 1.5 mm (on the soles of the feet). There are five cell layers in the epidermis: from deep to superficial these are the strata basale, spinosum, granulosum, lucidum, and corneum. The stratum basale, or basal layers, contain mitotically active basal cells, which migrate superficially to replace the nondividing outer layers. As the cells migrate away from the vascular supply in the dermis, they lose their cytoplasm, change shape and composition, and begin to accumulate keratin. The outer stratum corneum is composed of layers of flattened dead skin cells that continuously shed. This keratinized layer of the skin is responsible for barrier function, retaining water and shedding chemical irritants and pathogens. In addition to keratinocytes, the epidermis contains specialized elements such as melanocytes, Langerhans cells, and Merkel cells.
The dermis is composed of connective tissue and is connected to the epidermis by a basement membrane. The dermal layer contains blood and lymphatic vessels, sensory receptors, and skin adnexa (hair follicles, sweat and sebaceous glands, and apocrine glands) (Fig. 3a). The microvasculature in the dermal layer regulates body temperature by dilation and constriction. The papillary region of the dermis is more superficial, and is composed of looser connective tissue arranged in projections or papillae. The underlying reticular dermis is thicker and composed of denser connective tissue. The reticular dermis contains the largest concentrations of extracellular matrix proteins, as well as the adnexal structures.
2.2.1 Hair
Each hair arises in a tubular invagination of the epidermis into the dermis. The epithelium of the hair is arranged in three concentric layers: the medulla, the cortex, and cuticle. The hair follicle is tubular sheath composed of an inner epithelial sheath continuous with the epidermis and an outer connective tissue sheath. At the lower end, the root and follicle form a hair bulb connected to a papilloma consisting of fibroblasts, collagen, and rich in capillaries. Normal hair is divided into three parts: the infundibulum, the isthmus, and the inferior segment described above.
Hair growth is cyclical and is divided into three separate and distinct phases: anagen, telogen, and catagen.
Anagen Phase is mitotically active and there is rapid growth i.e., scalp hair.
Telogen Phase is a dormant and mitosis is arrested i.e., eyebrows, pubic hair, and axillary hair. This phase lasts for months to years.
Catagen Phase occurs when the root is separated from the hair bulb, pigment is terminated and the hair root is separated from the bulb.
Radiation in modest does to scalp (10–20 Gy) can initiate hair loss within 2–4 weeks and if the total dose is less than TD50; hair will regenerate. It is important to note that telogen hairs in eyebrows are often spared when scalp hair is shed.
2.2.2 Sebaceous Glands
Sebaceous glands are lined by actively proliferating stratified epithelium continuous with the germinal layer of skin. Mitoses are frequent in cells close to walls of excretory duct; newly produced cells move into secretory regions. Modest low doses of radiation have utilized to treat skin acne on face and chest when sebaceous gland activity is stimulated in adolescents.
2.2.3 Sweat Glands
Sweat Glands are simple coiled tubular glands deep in the dermis with its secretory merocrine myoepithelial cells which are specialized post mitotic cells. Sweat glands are more radioresistant and require large doses comparable to producing acute moist dermatitis to ablate. This can occur when the axilla is in the field when irradiating breast cancers.
The hypodermis or subcutis connects the dermal layer to the underlying muscle, bone, and fascia and is, by volume, composed primarily of fat. This layer serves as one of the major sites of fat storage by the body, and also contains macrophages, fibroblasts, and larger caliber blood vessels. The subcutaneous layer normally provides a loose layer of connective tissue to allow for cushioning and articulation during body movement. It is the primary site of fibrotic replacement following high-dose radiation.
3 Physiology and Biology
Skin has been utilized for numerous radiobiologic studies to characterize its radioresponsiveness (Table 1). Pig skin was initially favored because of its histologic characteristics being similar to humans however, more recently mouse leg has been adopted more widely. Radiation reactions have reproducible grading scales and dose time fractionation has been well studied. The clinical course of radiation skin murine reactions is similar to humans and useful for studying the genetic and molecular basis for radiosensitive versus radioresistant strains (Hall and Okunieff).
Table 1
Cytokines and growth factors implicated as mediators for the development of late radiation effects on skin
Cytokines, growth factors, and other proteins | Potential mechanisms of actions |
---|---|
IL-1 beta | Stimulates proliferation of keratinocytes and fibroblasts (Shenkier and Gelmon 1994; Yeo et al. 1997) Increases dermal angiogenesis (Schwartz et al. 2003) |
IL-6 | |
IL-8 | Chemokine (Saif et al. 2008) |
Eotaxin | Chemokine (Saif et al. 2008) |
TGF-B1 | Enhances collagen production in response to radiation (Denham and Hauer-Jensen 2002; Cassady et al. 1975; Khanfir and Anchisi 2008) Accelerates the terminal differentiation of progenitor fibroblast to postmitotic functional fibrocytes Stimulates the synthesis of extracellular matrix proteins and MMPs (Bostrom et al. 1999) |
PDGF | Induces fibroblast differentiation (Hird et al. 2008) |
CCN2 | Involved with TGF-B1 in stimulating fibrosis (Hird et al. 2008) |
TNF-α | |
CTGF | Promotes fibrosis and secreted by fibroblasts and endothelial cells (Greco et al. 1976) |
Smad3 | Transduces signaling effects through TGF-B1 (increases chemoattraction and elaboration of extracellular matrix by fibroblasts, inhibitory effects of keratinocyte proliferation, and migration) (Cassady et al. 1975) |
The pathophysiologic mechanism of late changes, particularly fibrosis, in response to radiation is incompletely understood. Transforming Growth Factor Beta (TGF-β) is a secreted protein that serves a regulatory role in normal tissue inflammation and remodeling by controlling proliferation, differentiation, and secretory functions. TGF-β is expressed in irradiated tissue within hours of exposure (Rodemann and Bamberg 1995; Rubin et al. 1992; Rodemann et al. 1991), and has been correlated with late fibrotic changes in several tissue types (Anscher et al. 1998, 2003), including skin (Kumar et al. 2008). Abrogation of downstream mediator Smad3, a pro-inflammatory signaling molecule induced in response to TGF-β, appears to protect tissue from late fibrotic changes after radiation exposure (Arany et al. 2007; Flanders et al. 2008, 2002; Martin et al. 2000).
TGF-β serves a complex regulatory role in wound healing and tissue remodeling. It is synthesized and secreted by several cell types including tissue macrophages, epithelial and endothelial cells, and fibroblasts. The most prominent isoform (TGF-β1) is synthesized and secreted in an inactive form, and activated by various proteases in the extracellular matrix. It then binds to serine/threonine kinase receptors on the cell surface of mature and immature fibroblasts, endothelial cells, and hematopoietic cells, among others (Rodemann and Blaese 2007).
TGF-β regulates extracellular matrix remodeling by increasing fibroblast proliferation, differentiation, and activation (Rodemann et al. 1991, 1996; Lara et al. 1996; Burger et al. 1998; Herskind et al. 1998; Hakenjos et al. 2000), and thereby increasing secretion of extracellular matrix component proteins (Canney and Dean 1990; Vozenin-Brotons et al. 1999; Schultze-Mosgau et al. 2002). TGF-β promotes its own secretion by fibroblasts in a self-amplifying cascade (Burger et al. 1998). In response, the secretion of most matrix proteins, including collagen, is increased, and matrix proteinases are decreased (Mayer 1990; Hageman et al. 2005; Zhao et al. 2001). Epithelial cell proliferation is diminished, and there is chemotaxis of mast cells and macrophages. The result is increasing production, processing, and deposition of collagen (fibrosis), and loss of epithelial reconstitution of normal tissue structure.
The initiating event in TGF-β activation in response to radiation is poorly understood. Latent TGF-β in the extracellular matrix may be activated by exposure to ionizing radiation (Rodemann and Blaese 2007; Barcellos-Hoff et al. 1994; Barcellos-Hoff 1998). This may involve proteolytic enzymes which act in the presence of radiation-induced reactive oxygen species. This is supported by experimental evidence that free radical scavengers may prevent TGF-β increases as well as fibrosis in irradiated lung (Vujaskovic et al. 2002; Epperly et al. 1999). Other potential sources of TGF-β include endothelial cells, which may release TGF-β in direct response to radiation, or from normal dermal components such as fibroblasts, epithelial cells, macrophages, or endothelial cells as a response to tissue damage. The latter may be the predominant mechanism of consequential late fibrosis following severe acute skin injury, as the severe acute epidermal and dermal tissue injury activates a generalized fibrotic response.
Endothelial cell damage may be a direct result of radiation exposure (endothelial apoptosis or necrosis) or indirectly thorough loss of epithelial barrier function or adjacent tissue necrosis. Damaged endothelial cells secrete TGF-β, which then promotes fibrotic replacement of the damaged dermis. It also increases endothelial permeability, which results in inflammation, and activates the coagulation cascade (Denham and Hauer-Jensen 2002). The inflammatory response includes the chemotaxis and proliferation of macrophages and mast cells which is then direct neovascularization. The histologic result is hypovascular, fibrotic dermis, with a network of tortuous vessels, and telangiectasia. Cytokines that have been implicated in late skin effects from RT are summarized in Table 1.
4 Pathophysiology
Following a large single or fractionated dose there is a linear loss of basal cells, reaching a nadir at ~21 days, followed by exponential reepithelialization to control levels and above by 28–32 days (Fig. 4). The mitotic index and labeling index are increased during this period of regeneration. The generation time for this period is estimated to be 15 h. Complete regeneration of the epidermis is produced at all dose fractions up to 45 Gy. Reepithelialization at 32–36 days can then be followed by a second ulceration and necrosis. During the period of basal cell degeneration and regeneration the endothelial population parameters do not change.
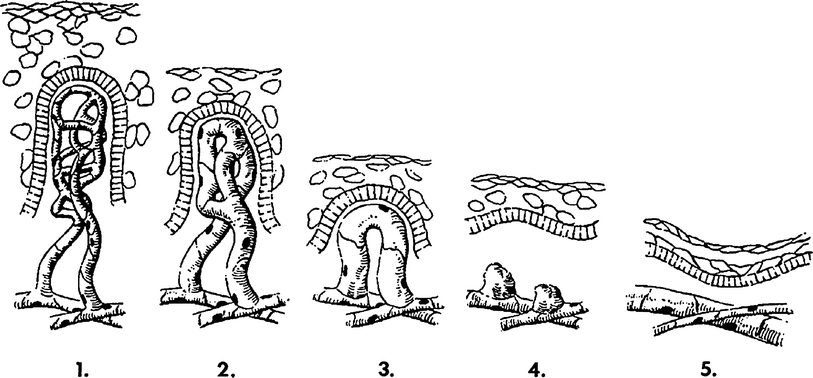
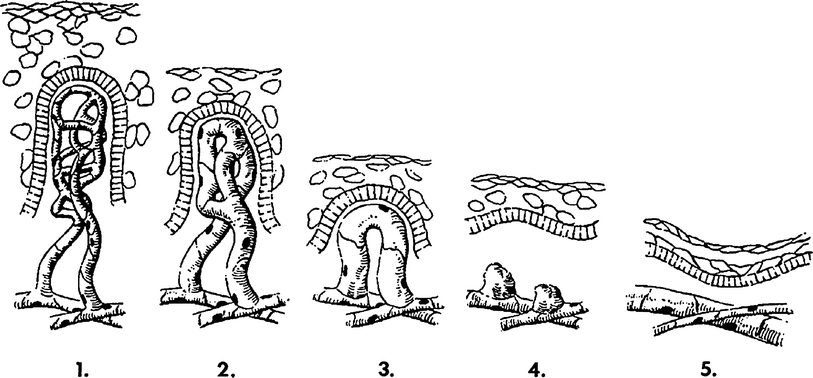
Fig. 4
A diagram of the sequence of microvessel changes in the skin functional unit in time following irradiation. The microvessel tuft is shown as a folded manifold. This model incorporates the loss of cells with vessel shortening and the loss of cells with loss of the short branch of the manifold. A time scale is ignored but must be represented by months and years with the development of telangiectasia. The telangiectasia produced is represented as formed in the vessels of the rete plexus that supplied the tuft. (From Archambeau et al. 1995, IJROBP LENT SOMA)
Change produced by irradiation in the microvessel endothelium, which exits as a line of 10–20 single cells without supporting adventitia, is not well documented. Endothelial proliferation has not been observed; therefore, the principle change is one of cell loss without replacement. The changes documented histologically are cell loss, a decreased in the number of vessel lumens seen on microscopic section (representing vessel shortening), decrease in tuft density and dilatation. The turnover time or replacement time of endothelial cells is not known, but is estimated to take months of years as evidenced by the time required for late effects to be produced.
A diagram of the sequence of microvessel changes in the skin functional unit in time following irradiation. The microvessel tuft is shown as a folded manifold. This model incorporates the loss of cells with vessel shortening and the loss of cells with loss of the short branch of the manifold (Fig. 3b). A time scale is ignored but must be represented by months and years with the development of the telangiectasia. The telangiectasia produced is represented as formed in the vessels of the rete plexus that supplied the tuft.
5 Clinical Syndromes
5.1 Acute Erythema Phase
Hyper-acute reactions (within hours of RT): Skin changes after radiation exposure follow a predictable course dictated by radiation dose, timing, and the biology of the human inflammatory reaction (Tables 2, 3). The earliest clinically evident reaction is erythema that may occur and resolve within hours, and is normally only evident after relatively high-dose exposure. The threshold dose is 2 Gy or greater, and this effect is noted in therapeutic courses aimed at cutaneous targets, lower energy treatment courses (kilovoltage), or hypofractionated treatment regimens. Histologically, there is a vasodilation and a transient permeability increase in capillaries that results in mild erythema and edema at 2–24 h following exposure (Hall and Giaccia 2005).
Table 2
LENT SOMA Skin/Subcutaneous tissue
Grade 1 | Grade 2 | Grade 3 | Grade 4 | |
---|---|---|---|---|
Subjective | ||||
Scaling/roughness sensation | Present/asymptomatic Hypersensitivity, pruritus | Symptomatic Intermittent pain | Require constant attention Persistent pain | Debilitating dysfunction |
Objective | ||||
Edema Alopecia(scalp) Pigmentation change Ulcer/Necrosis Telangiectasia Fibrosis/Scar Atrophy/Contraction (depression) | Present/asymptomatic Thinning Transitory, slight Epidermal only Minor Present/asymptomatic Present/asymptomatic | Symptomatic Patchy, permanent Permanent, marked Dermal Moderate < 50 % Symptomatic Symptomatic/ < 10% | Secondary dysfunction complete, permanent Subcutaneous Gross ≥ 50 % Secondary dysfunction Secondary dysfunction/10–30 % | Total dysfunction Bone exposed Total dysfunction Total dysfunction/ > 30 % |
Management | ||||
Dryness Sensation Ulcer Edema Fibrosis/Scar | Intermittent medical intervention | Medical intervention Continuous medical intervention Medical intervention Medical intervention Medical intervention | Surgical intervention/amputaion Surgical intervention/amputaion Surgical intervention/amputaion | |
Analytic | ||||
Color photographs | Assessment of changes in appearance |
Table 3
Late skin changes may be broadly, and somewhat arbitrarily, segregated into categories and associated examples as shown
Focal | Global | |
---|---|---|
Subclinical | 1. Latent reduced capacity to tolerate future insults (e.g., difficulty with wound healing) 2. Imaging abnormalities | 1. Reduced tolerance to alterations in temperature |
Clinical | 1. Ulceration 2. Fibrosis/retraction 3. Hair loss 4. Atrophy and skin thinning | 1. Cosmetic changes and secondary challenges with socialization 2. Reduced mobility of joints underlying fibrotic areas of skin 3. Abnormalities in thermoregulation |
Prior to adoption of the Roentgen (R), and later the Gray (Gy), as a measure of radiation dose, skin erythema dose (SED) was used as a crude clinical measure of patient radiation exposure (Khan 2003). For lower energy radiation, this was a reasonable measure of the total dose deposited because the maximum dose was deposited at the skin surface. This transient acute reaction is no longer commonly noted due to the increased use of multiple or rotational fields and megavoltage therapy, high-energy, relatively skin sparing, radiation treatment beams, and the use of fraction size of ≤ 2 Gy per day. Acute, transient skin erythema is still commonly reported following interventional diagnostic and therapeutic procedures with prolonged fluoroscopy screening times, due to less penetrating kilo-voltage beams. The SOMA LENT system provides a systematic manner to assess, categorize and grade acute reactions to the skin (Table 2), and to specific organs where the skin reaction is a prominent component (e.g., the Breast, see “Breast Cancer“). Skin changes may also be broadly, and somewhat arbitrarily, segregated into focal versus global, and clinical versus subclinical (Table 3).
5.2 Detection and Diagnosis
Since the integument is readily visible on physical examination, the gross skin changes produced by irradiation are summarized in Table 4.
Table 4
Gross skin changes produced by irradiation
Acute changes | Late changes |
---|---|
Erythema | Atrophy |
Pigmentation | Scaling |
Dry desquamation | Pigmentation |
Moist reaction | Atrophy |
That heals | Fibrosis |
Heals partially | Telangiectasia |
Does not heal | Necrosis |
5.2.1 Acute Moist Dermatitis
The more sustained, common, and relevant reactions take place over a matter of weeks following initial exposure (Fig. 5a, b, c, d, e). Acute radiation dermatitis progresses through stages of severity based on the accumulation of radiation-induced changes to dermal vascular and appendageal structures, epidermal stem cells, and activation of inflammatory pathways. The severity of dermatitis is a function of accumulated damage and therefore related to radiation dose. Dermatitis may be graded by the National Cancer Institute Common Terminology Criteria for Adverse Events (CTCAE). This scale is symptom-based, and does not inherently distinguish between acute and late reactions based on the timing of the symptom in relation to radiation exposure. Common acute and late dermatologic symptoms not included as radiation dermatitis by the CTCAE.
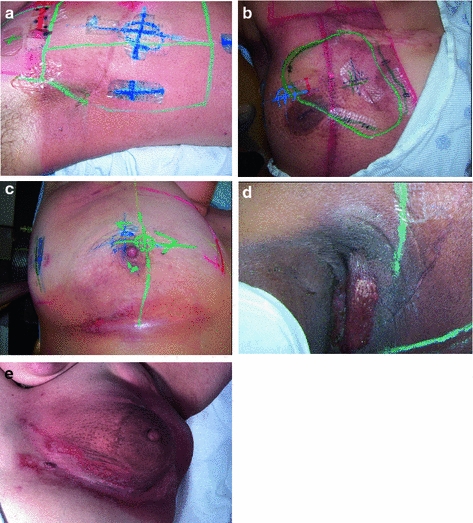
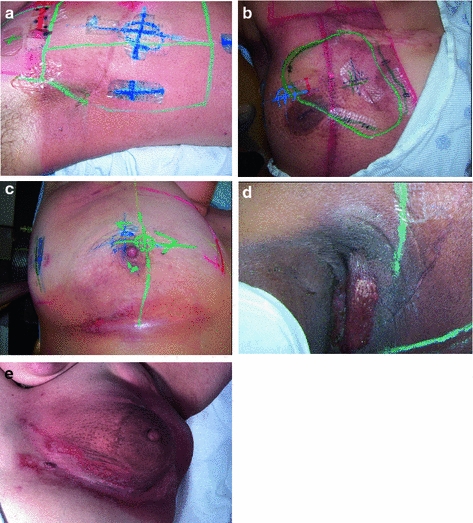
Fig. 5
Acute Dermatitis. a Early erythema during radiation therapy for breast cancer. b Hyperpigmentation as an early manifestation of radiation dermatitis. Note that the scar tissue in the upper inner quadrant is spared. c Dry desquamation of the inframammary fold. d Patchy moist desquamation in an axillary skin fold. e Confluent moist desquamation
Grade 1 dermatitis manifests as faint skin erythema within the treatment area, or dry desquamation. Erythema is seen in two contexts: first, as discussed above, there may be a transient vasodilation in the hours after a single fraction skin exposure of 2 Gy or higher. More commonly, erythema develops over the first two to three weeks of fractionated radiation with accumulated exposure (Fig. 5a). In some patients, this manifests as hyperpigmentation (Fig. 5b). With continuing or higher dose radiation exposure, damage to the basal cells in the epidermis may progress until this stem cell population is lost in localized areas, resulting in dry desquamation (Fig. 5c).
Further and more widespread damage to the basal layer leads to further desquamation, and the production of a fibrinous exudate due to increased arteriole permeability and edema in the underlying dermis. This is moist desquamation. The CTC differentiates moist desquamation based on whether it is patchy and localized to skin folds (grade 2, Fig. 5d), or confluent affecting a more widespread area (grade 3, Fig. 4e). Skin folds and creases are particularly susceptible to such reactions since the local dose may be increased due to a “local loss of skin sparing”. Additionally, these areas may be subject to additional trauma associated with friction between the two “opposing sides” of the skin fold during normal movement, and/or overlying clothing (e.g., waist-bands of pants or a women’s brassiere). More widespread moist desquamation in areas less prone to mechanical trauma is indicative of additional accumulated damage.
The outer strata of the epidermis are composed of fully differentiated and nondividing epithelial cells, which are continuously renewed from proliferating cells in the basal layer of the epidermis. Newly formed daughter cells in the basal layer migrate outward as they differentiate, over approximately 14 days, to reconstitute the outer strata. The turnover time is a function of the local thickness of the epidermis, and can vary from 10 to 40 days. Desquamation occurs due to radiation-induced loss of this basal layer, and manifests in the second or third week of fractionated radiation, as the loss of basal stem cells becomes clinically evident. Moist desquamation is an indication of more complete loss of the basal layer; the fibrinous exudate is a result of increased permeability in the dermal vasculature, along with loss of normal epidermal basement membrane integrity.
5.2.2 Dermis
The underlying dermis is composed of connective tissue and houses the vascular and lymphatic network, as well skin adnexa including hair follicles, sweat and sebaceous glands, and sensineural structures. Acutely, most clinically evident changes in the dermis occur due to vascular changes. Vasodilation and increasing vascular permeability occur early (Fajardo and Berthrong 1988), and the resulting perivascular inflammation results in clinically characteristic erythema and edema. Skin adnexal cells are relatively radiosensitive as well, and may not regenerate following exposure. The process of epilation begins within days of radiation exposure (Sieber et al. 1993). Sebaceous glands have similar sensitivity, and eccrine sweat glands become dysfunctional shortly afterwards in a fractionated radiation treatment course. Histologically, these glandular structures demonstrate apoptosis, necrosis, and loss of normal mitotic activity (Malkinson and Keane 1981). Clinically, this leads to either acute and chronic hypohydrosis or anhidrosis.
5.2.3 Regeneration
Regeneration of areas of moist desquamation occurs primarily through replacement of epidermal basal cells either from islands of intact cells within the epidermis or by the migration of such cells from adjacent, uninvolved areas. Normal healing of the radiation wound becomes clinically evident approximately 2 weeks after exposure, consistent with the basal cell turnover time. Widespread confluent mucositis (CTCAE grade 3), or more severe toxicity such as necrosis of the epidermis or underlying dermis, may not undergo complete regeneration of the structural and adnexal elements. Instead, there is prolonged inflammation and progression to early fibrosis. Histologically, the normal epidermis is replaced by fibroblasts and a collagen scar. This is in contrast to the more common late fibrosis, which arises following the regeneration of relatively normal-appearing skin. “Consequential late effects” (Dorr and Hendry 2001) are those directly related to the severity and extent of acute events, and result from the failure of normal healing of the radiation wound, rather than the more commonly noted chronic toxicity, the severity of which is not always predicted by the extent and severity of acute events.
5.2.4 Late Changes of Atrophy and Fibrosis
Late radiation toxicity occurs months to years following exposure, following a period during which the skin may not exhibit significant abnormalities (Figs. 6a, b, c, d, e, f, and 7a, b, c, d, e). Unlike consequential late effects, the risk and severity of true late skin changes are not thought to be associated with the risk and severity of acute dermatitis, except that both occur as a function of radiation dose. The clinical hallmarks of late radiation dermatitis are fibrosis, atrophy, and telangiectasia.
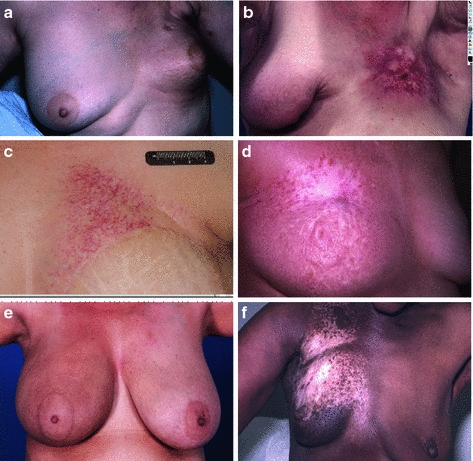
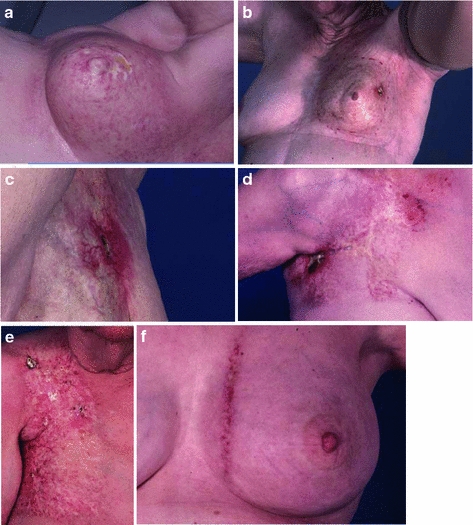
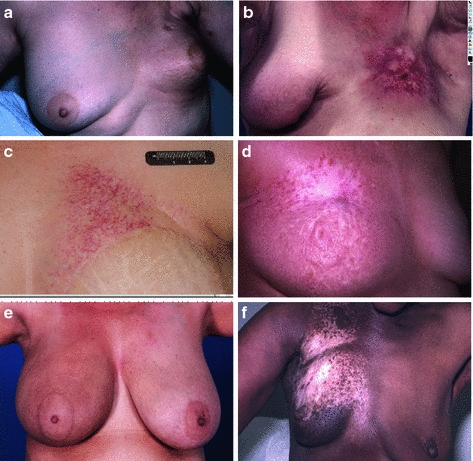
Fig. 6
Chronic Fibrosis. In panel a, there is retraction of the treated left breast, with mild to moderate overlying fibrosis. b More significant and localized fibrosis with loss of normal skin markings, pigmentation changes, skin retraction, and early ulcerative changes. c Telangiectasia which have developed years after radiation. d Pigmentation changes -mixed hypo- and hyperpigmented areas. e Hyperpigmentation one year after completion of radiation. f Hypopigmentation
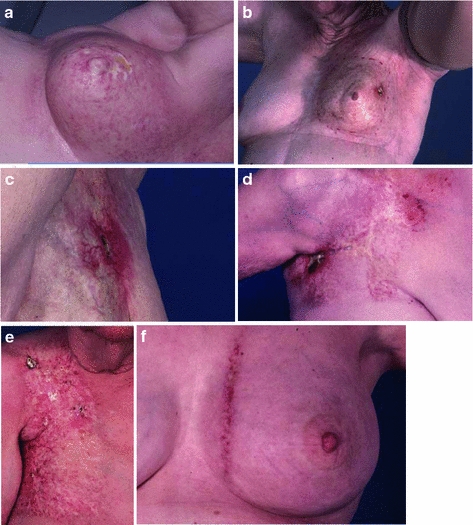
Fig. 7
Late radiation necrosis. a A superficial area of necrosis. b A necrotic ulceration in the background of marked fibrosis. c Necrosis with superficial erosion into the subcutaneous layer. d Advanced necrosis. e A large area of severe fibrosis. f Match line fibrosis at the junction of a breast tangent field with an internal mammary field. The most significant skin changes are limited to the area of overlap, which received radiation from both fields
The late skin toxicity with the most functional consequence is subcutaneous fibrosis. Activation of growth factors induces replacement of the subcutaneous adipose tissue with fibrous tissue, leading to limitations in range of motion, contraction, pain, and poor cosmesis (Fig. 6a, b). Fibrosis of hair follicles may lead to permanent alopecia. Even in cases where dermal and subcutaneous fibrosis is not clinically evident, there may be atrophy of the skin adnexa. Hair follicles, sebaceous, and sweat glands may be absent in previously irradiation skin because these are not regenerated during normal radiation wound repair.
Loss of glandular elements leads to anhidrosis when extensive skin areas are irradiated, such as in total skin electron therapy. The microvasculature of the dermis and subcutis may develop excess myointimal proliferation, leading to a functional hypovascularity. Tortuosity within small vessels, and microthrombi, results in visible telangiectasia (Fig. 6c). Irregular regeneration of the basal layer of the epidermis may be evident as dyspigmentation (Fig. 6d, e, f).
Paradoxically, there may be a decrease in the population of resident skin fibroblasts in atrophic skin, with loss of the normal collagen structure leading to increased skin fragility and poor wound healing. Necrosis can occur in response to minor trauma or spontaneously, as a consequence of poor tissue perfusion, because of impaired normal tissue repair (Fig. 7a, b, c, d). Care should therefore be taken when considering a biopsy or other procedure involving irradiated skin.
The pathologic severity of late fibrosis is dependent on the radiation dose and volume, and may be modified by underlying comorbid illness and the genetic background. The clinical severity may range from poor cosmesis to significant loss of function, and additionally depends on the anatomic restrictions to motion that result from the underlying fibrosis (Fig. 7e).
6 Radiation Tolerance
6.1 Dose Time Fractionation
One of the earliest reports to systematically document time dose fractionation, was by Strandquist (1944
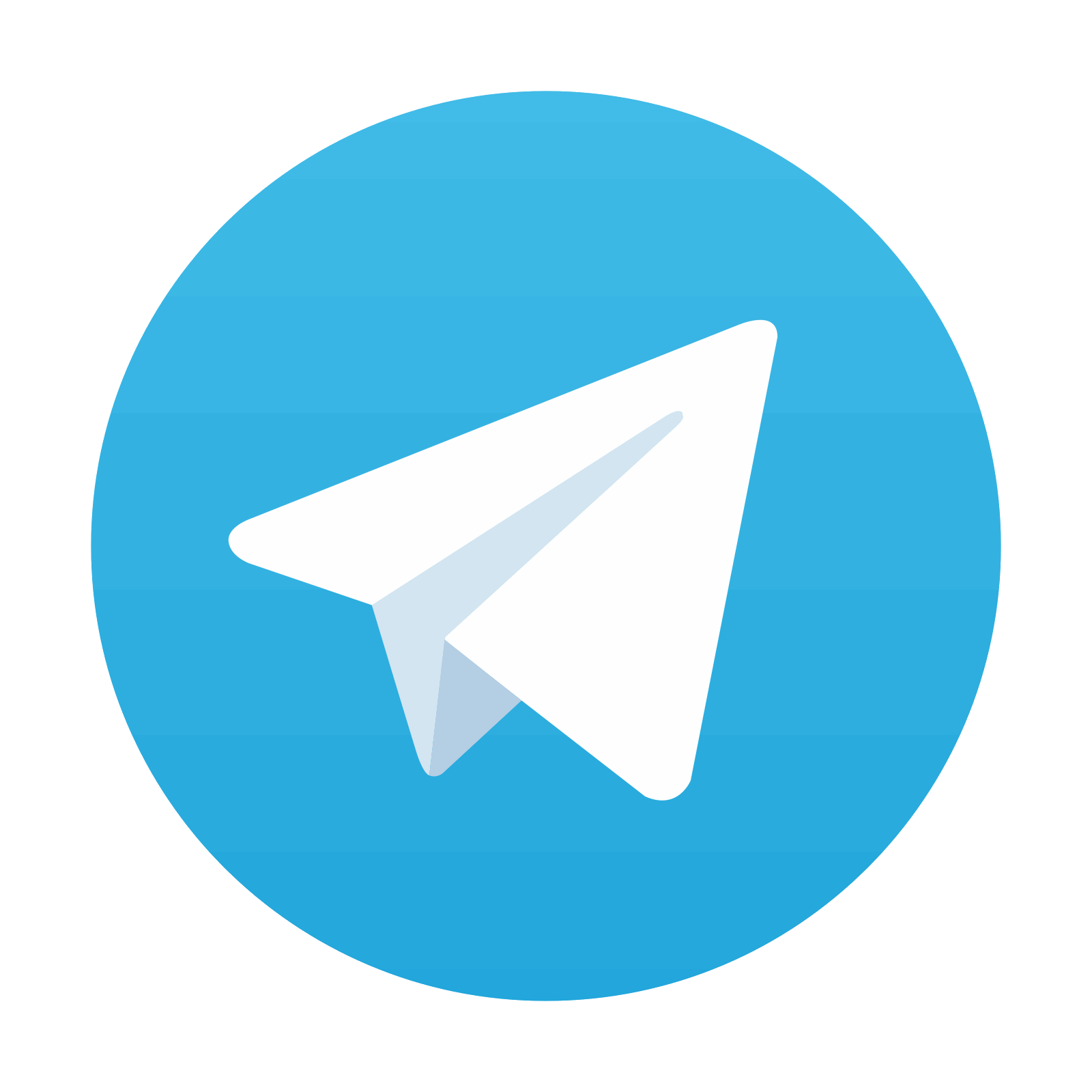
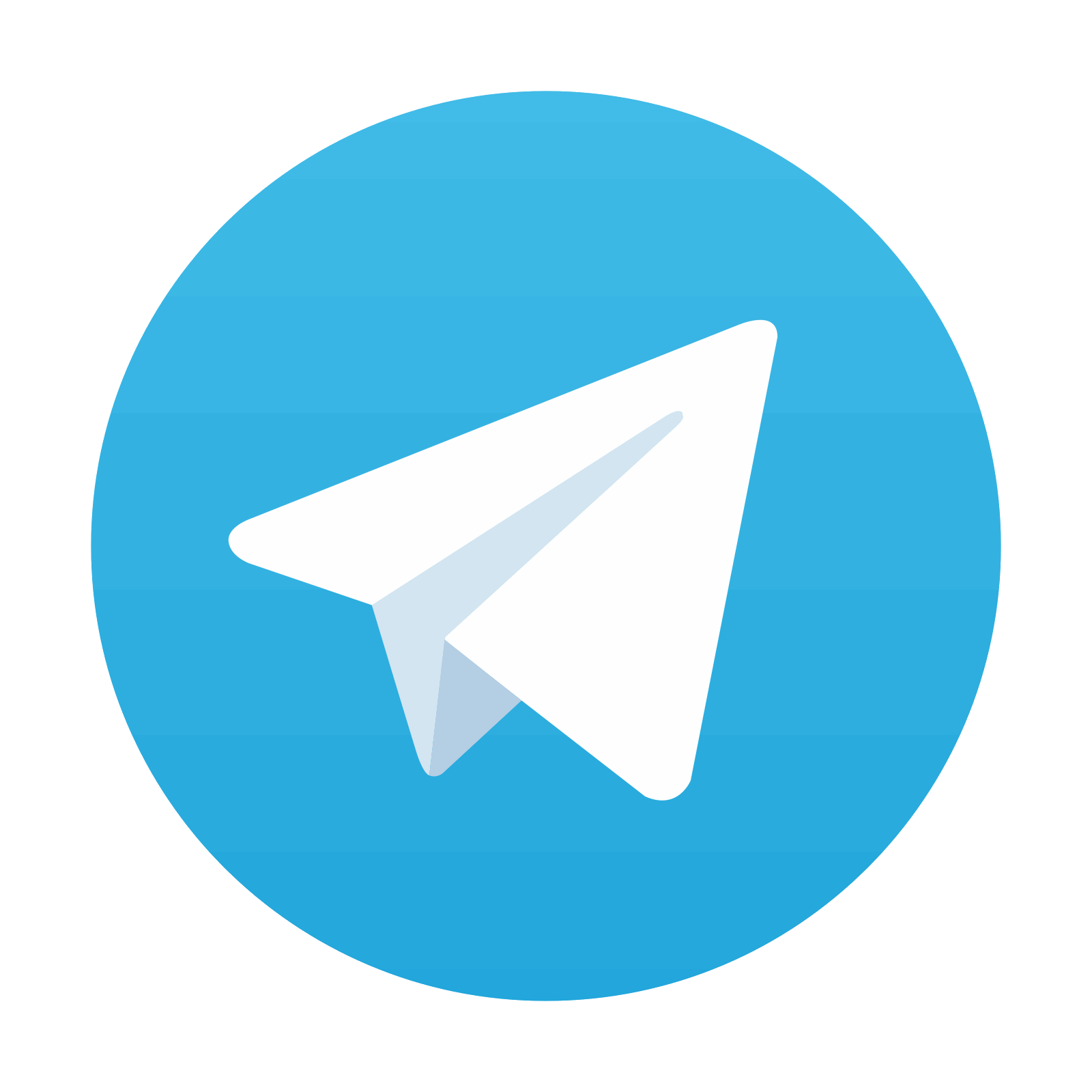
Stay updated, free articles. Join our Telegram channel

Full access? Get Clinical Tree
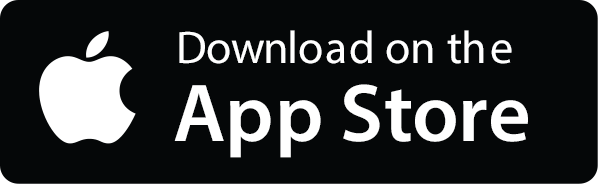
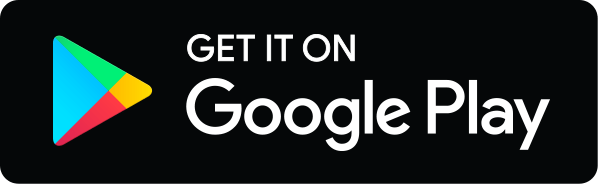