The Biology and Exploitation of Tumor Hypoxia
Oxygen homeostasis is deregulated in several pathologic settings. Hypoxia, or low oxygen conditions, exists in virtually all solid tumors. As tumor cells rapidly expand, their growth outpaces the ability of the existing vasculature to supply both nutrients and oxygen, resulting in tumor hypoxia. Cells experiencing hypoxia can cope with the low oxygen tension by either increasing delivery of oxygen or adapting to the low oxygen level.
▪ HYPOXIA-INDUCIBLE FACTOR
Hypoxia-inducible factors (HIFs) are transcription factors that facilitate both oxygen delivery and adaptation to oxygen deprivation by regulating the expression of genes that are involved in many cellular processes including glucose uptake and metabolism, angiogenesis, erythropoiesis, cell proliferation, and apoptosis. HIFs bind to DNA as heterodimers composed of an oxygen-sensitive α-subunit and a constitutively expressed β-subunit, also known as the aryl hydrocarbon receptor nuclear translocator (ARNT). To date, three HIFs (HIF-1, -2, and -3) have been identified that regulate transcriptional programs in response to low oxygen levels.
HIF-1 was the first HIF family member to be characterized. Using DNA affinity purification, HIF-1 was identified as a hypoxic-induced factor that bound an 18-nucleotide fragment of the erythropoietin enhancer required for the hypoxic activation of erythropoietin in Hep3B cells. Structural analysis of the HIF-1α protein revealed four distinct domains, including an oxygendependent degradation domain (ODD) that regulated HIF-1α degradation by the ubiquitinproteasome pathway. HIF-1 has been designated the global regulator of hypoxia-inducible gene expression because it is ubiquitously expressed and induces the expression of most hypoxiainducible genes.
HIF-2 was the second HIF family member identified and shares approximately 50% sequence homology with HIF-1. HIF-2α is also sensitive to changes in oxygenation and possesses an ODD. Although HIF-2α heterodimerizes with ARNT like HIF-1α, the expression of HIF-2α is restricted to endothelial cells, glial cells, pneumocytes type II, cardiomyocytes, fibroblasts of the kidney, interstitial cells of the pancreas and duodenum, and hepatocytes.
The third HIF family member, HIF-3α, also possesses an ODD, can dimerize with ARNT and bind to hypoxia response elements in vitro. The role of HIF-3 in the hypoxic regulation of target gene expression in vivo is not well understood.
Oxygen-Dependent Regulation of Hypoxia-Inducible Factor
The transcriptional activity of HIF is regulated through changes in protein stability and through the recruitment of transactivation coactivators (Fig. 26.1). In the presence of oxygen, HIF-1α is hydroxylated on two highly conserved proline residues: prolines 402 and 564, both found within the ODD. A family of 4-prolyl hydroxylases (PHDs), distinct from the structurally important collagen-4-prolyl hydroxylases, modifies these prolines. These enzymes catalyze a hydroxylation reaction, which uses molecular oxygen and 2-oxoglutarate as substrates and iron and ascorbate as cofactors. Hydroxylation of HIF-α is necessary for binding to the von Hippel-Lindau (VHL) tumor suppressor protein. Under normoxic conditions, HIF-α protein is rapidly turned over because of hydroxylation, binding to VHL, and destruction by the proteasome. Under hypoxic conditions, the 4-prolyl hydroxylases have reduced activity as oxygen is a requisite substrate of the hydroxylation reaction. Decreased hydroxylation of HIF-α allows it to escape recognition by VHL, resulting in protein stabilization. Once stabilized, HIF-α dimerizes with ARNT and binds to a core sequence of 5′-RCGTG-3′ in the enhancer elements of target genes to initiate gene transcription.
An additional layer of HIF regulation occurs at the level of recruitment of coactivators. A third hydroxylation of HIF-α takes place on the C-terminal transactivation domain on asparagine residue 803. This hydroxylation reaction is catalyzed by a separate asparaginyl hydroxylase, factor inhibiting HIF (FIH). Hydroxylation of
this residue under normoxic conditions prevents interaction with p300, which, in turn, prevents gene transcription. These modifications of HIF demonstrate the very stringent regulation of HIF under normoxic conditions.
this residue under normoxic conditions prevents interaction with p300, which, in turn, prevents gene transcription. These modifications of HIF demonstrate the very stringent regulation of HIF under normoxic conditions.
Cancer Mutations that Activate Hypoxia-Inducible Factor
Tumor hypoxia is only one mechanism of HIF activation in tumors. HIF can also be activated under normoxic conditions through mutations in VHL, which plays a central role in regulating HIF protein stability. Inactivation of VHL results in HIF stabilization and increased target expression irrespective of oxygen concentrations. Germ line mutations in VHL result in a familial tumor syndrome that predisposes patients to the development of highly vascularized neoplasms including hemangioblastomas of the retina and central nervous system, renal cell carcinomas (RCCs), endocrine and exocrine pancreatic tumors, as well as pheochromocytomas. VHL is also found to be inactivated in most sporadic RCC and hemangioblastomas, reinforcing the important role of VHL tumor suppressor activity in HIF regulation and tumor progression. In addition to mutations in VHL, inactivation of the PTEN tumor suppressor gene can also result in increased HIF activity in tumors through the mammalian target of rapamycin (mTOR) signaling pathway.
Important Roles of Hypoxia-Inducible Factor in Tumors
The ability of tumor cells to adapt and survive their growth inhibitory microenvironment is accomplished in part by HIF activation. HIFs have been found to promote key steps in tumor progression, including angiogenesis, metabolism, and metastasis.
Tumor Angiogenesis
The formation of new blood vessels is critical for tumor expansion because the supply of oxygen and nutrients becomes limited in tumor cells that are located more than 100 µm away from a blood vessel. Unlike normal tissues where there is a balance between proangiogenic and antiangiogenic factors, tumors tip the balance toward proangiogenic factors. Of all the proangiogenic factors induced by HIF, vascular endothelial growth factor-A (VEGF-A) appears to be the most critical because it has potent proangiogenic properties and is expressed in a large number of human tumors.
Tumor Metabolism
More than 70 years ago, cancer cells were found to shift glucose metabolism from an oxidative to glycolytic pathway. This shift has become known as the Warburg effect, and is characterized by decreased mitochondrial respiration and increased lactate production even with oxygen. Numerous studies have established that HIF-1 regulates the expression of genes involved in glycolytic metabolism including glucose transporters, glycolytic enzymes, lactate production, and pyruvate metabolism.
Tumor Metastasis
Metastasis is the cause of most human cancer deaths. It occurs in a series of distinct steps that include tumor cell invasion, intravasation, extravasation, and proliferation. HIF activation correlates with metastasis in multiple tumors and promotes metastasis through the transcriptional regulation of key factors such as E-cadherin, lysyl oxidase, and CXCR4 that govern cell adhesion, extracellular matrix formation, and cell migration.
Hypoxia-Inducible Factor and Radiotherapy
It has recently been proposed that HIF-1 plays an important role in determining tumor response to radiotherapy and tumor regrowth. Radiation results in a reoxygenation-dependent increase in HIF-1 activity by two distinct mechanisms. Tumor reoxygenation results in both HIF-1 stabilization and enhanced translation of HIF targets through the release of reactive oxygen species. The effects of HIF on tumor radiosensitivity are twofold. On one hand, HIF-1 stabilization promotes tumor vasculature radioresistance through the release of proangiogenic cytokines such as VEGF. Paradoxically, HIF-1 can also induce tumor radiosensitivity through the induction of apoptosis. Overall, HIF-1 stabilization promotes radioresistance because HIF-1-deficient tumors are more sensitive to radiation compared to wildtype tumors.
Studies by Ahn and Brown’s laboratory have identified an important role for bone marrow (BM)-derived cells in regenerating tumor vasculature following radiotherapy. Tumors grown
in previously irradiated tissues exhibit decreased growth caused by insufficient neovascularization that results from radiation-induced injury to the host vasculature and connective tissue. They hypothesized that the vasculature of tumors grown in previously irradiated tissues is derived from cells from the BM. In particular, they demonstrated an important role for tissue MMP-9 and CD11b+ myelomonocytic cells from the BM to restore tumor growth in these preirradiated sites. HIF plays an important role in this recruitment of BM-derived cells by regulating several factors such as CXCR4 and SDF-1. Inhibition of HIF results in an inhibition of tumor vascularization. This study implicates vasculogenesis as an important target for adjunct therapy to radiotherapy.
in previously irradiated tissues exhibit decreased growth caused by insufficient neovascularization that results from radiation-induced injury to the host vasculature and connective tissue. They hypothesized that the vasculature of tumors grown in previously irradiated tissues is derived from cells from the BM. In particular, they demonstrated an important role for tissue MMP-9 and CD11b+ myelomonocytic cells from the BM to restore tumor growth in these preirradiated sites. HIF plays an important role in this recruitment of BM-derived cells by regulating several factors such as CXCR4 and SDF-1. Inhibition of HIF results in an inhibition of tumor vascularization. This study implicates vasculogenesis as an important target for adjunct therapy to radiotherapy.
▪ UNFOLDED PROTEIN RESPONSE
Prolonged periods of hypoxia can activate non-HIF signaling pathways such as the unfolded protein response (UPR). The UPR is a cellular stress response that is induced by the accumulation of unfolded proteins in the endoplasmic reticulum (ER) in order to deal with the problems associated with the accumulation of misfolded proteins. Activation of the UPR results in a transient inhibition of protein translation to prevent further misfolded proteins from accumulating and through the induction of chaperone proteins that function in the ER to assist in folding, trafficking, and secretion of proteins (Fig. 26.2). The UPR has been well characterized in yeast and, more recently, a greater understanding has been elucidated in humans. The ER stress sensor inositol-requiring kinase 1 (IRE1) is found both in yeast and in humans. IRE1 becomes activated through homodimerization and trans-autophosphorylation. Biochemically, the endoribonuclease activity of IRE1 splices the pre-messenger ribonucleic acid (mRNA) of the X-box binding protein (XBP1) transcription factor that results in translation of XBP1. The XBP1 transcription factor has many target genes involved in ER protein maturation. Koong et al. have shown that XBP1 could be inhibited by small molecules and that this
inhibition resulted in a significant inhibition of tumor growth.
inhibition resulted in a significant inhibition of tumor growth.
A second ER stress sensor is the protein kinase-like endoplasmic reticulum kinase (PERK). Activation of PERK prevents further translation of proteins. PERK activation occurs rapidly under severe hypoxia on the order of minutes, whereas under milder hypoxic conditions, PERK activation occurs on the order of hours. Through a combination of oligomerization and autophosphorylation, PERK becomes activated and inhibits translation by directly phosphorylating serine 51 of eIF2α, a key initiator of the mRNA translation machinery. Koumenis and colleagues have shown that activation of PERK is important to survive under hypoxic conditions as PERK-deficient cells undergo apoptosis following hypoxia, resulting in a lower survival rate compared to genetically matched cells that have the PERK pathway intact. These PERK-deficient cells are less tumorigenic, likely in part because of higher apoptosis in the hypoxic regions of the tumor. Thus, one cellular response to chronic conditions of hypoxic stress is to limit the load of misfolded proteins.
▪ RADIOSENSITIZING HYPOXIC CELLS
As described in Chapter 21, a great deal of experimental work through the years had established that at least in transplanted tumors in animals, tumor control by x-rays frequently is limited by the foci of hypoxic cells that are intransigent to killing by x-rays, which may result in tumor regrowth. Among the methods suggested to overcome this problem are treatment in hyperbaric oxygen chambers and the introduction of highlinear energy transfer (LET) radiations, such as neutrons and heavy ions. Chemical sensitizers address the same problem. High-LET radiations are discussed in Chapter 7. This chapter addresses hyperbaric oxygen, chemical radiosensitizers, and the latest approach, namely, hypoxic cytotoxins.
Hyperbaric Oxygen
Following the identification of hypoxia as a possible source of tumor resistance, a major effort was made to solve the problem by the use of hyperbaric oxygen. Patients were sealed in chambers filled with pure oxygen raised to a pressure of three atmospheres. Churchill Davidson at St. Thomas’ Hospital in London pioneered this work, but it was taken up by researchers on both sides of the Atlantic. The clinical trials that were performed involved small numbers of patients and were difficult to interpret because unconventional fractionation schemes were used; that is, a few large fractions were used because of the time and effort involved in the technical procedures. Patient compliance was also a problem because of the feeling of claustrophobia from being sealed in a narrow tube. There was also the serious risk of fire, because tissue is highly flammable in pure oxygen (as evidenced by the accident when the crew of a space capsule died in an oxygen fire on the ground), although in practice, an accident of this nature never happened during the treatment of several thousand patients. The largest multicenter trials performed by the Medical Research Council in the United Kingdom showed a significant benefit both in local control and in survival for patients with carcinoma of the uterine cervix and advanced head and neck cancer, but not for patients with bladder cancer. The data generated a great deal of debate. An overview of the trials showed a 6.6% improvement in local control, with a suggestion, too, of an increase in late normal tissue damage. Hyperbaric oxygen fell into disuse, partly because it is cumbersome and difficult in practice, and partly because of the promise of drugs that would achieve the same result by simpler means.
The notion of improving tumor oxygenation by breathing 100% oxygen rather than air has been revived in recent years by experiments involving carbogen. If pure oxygen is breathed, it tends to lead to vasoconstriction, a closing down of some blood vessels, which, of course, defeats the object of the exercise. This is avoided if 5% carbon dioxide is added to the oxygen, a mixture called carbogen. Breathing carbogen at atmospheric pressure, then, is a relatively simple attempt to overcome chronic hypoxia, that is, diffusion-limited hypoxia. The use of carbogen in combination with nicotinamide is described subsequently in this chapter.
Improving the Oxygen Supply to Tumors
A group at the Princess Margaret Hospital in Toronto showed convincingly that a blood transfusion prior to radiotherapy led to a significant
improvement in local tumor control probability in patients with carcinoma of the uterine cervix. Several other studies have shown that hemoglobin levels can influence the success of radiation therapy.
improvement in local tumor control probability in patients with carcinoma of the uterine cervix. Several other studies have shown that hemoglobin levels can influence the success of radiation therapy.
Tumor oxygenation also can be improved by the use of artificial blood substances such as perfluorocarbons. Because smoking can decrease tumor oxygenation, it is clearly advisable for patients to give up smoking, at least during radiotherapy.
Hypoxic Cell Radiosensitizers
Spurred largely by the efforts of radiation chemists (most notably, Adams), a search was under way in the early 1960s for compounds that mimic oxygen in their ability to sensitize biologic materials to the effects of x-rays. Instead of trying to “force” oxygen into tissues by the use of high-pressure tanks, the emphasis shifted to oxygen substitutes that diffuse into poorly vascularized areas of tumors and achieve the desired effect by chemical means. The vital difference between these drugs and oxygen, on which their success depends, is that the sensitizers are not rapidly metabolized by the cells in the tumor through which they diffuse. Because of this, they can penetrate further than oxygen and reach all of the hypoxic cells in the tumor, including those most remote from a blood supply. In the early 1960s, many simple chemical compounds were found to have the ability to sensitize hypoxic microorganisms. These studies were guided by the hypothesis, now known to be correct, that sensitizing efficiency is related directly to the electron affinity of the compounds.
Adams and his colleagues listed properties that would be essential for a clinically useful hypoxic cell sensitizer. First, it has to selectively sensitize hypoxic cells at a concentration that would result in acceptable toxicity to normal tissues. Second, it should be chemically stable and not subject to rapid metabolic breakdown. Third, it must be highly soluble in water or lipids and must be capable of diffusing a considerable distance through a non-vascularized cell mass to reach the hypoxic cells, which in a tumor may be located as far as 200 µm from the nearest capillary. Fourth, it should be effective at the relatively low daily doses of a few grays used in conventional fractionated radiotherapy. The first candidate compound that appeared to satisfy these criteria was misonidazole.
Misonidazole
Figure 26.3 illustrates the numbering of the basic ring structure of the nitroimidazoles. The side chain determines position 1, and the position of the nitro group (NO2) leads to the classification of the drug as a 2-nitroimidazole, 4-nitroimidazole, and so on. In general, 2-nitroimidazoles have a higher electron affinity than 5-nitroimidazoles, the class that includes metronidazole, which was briefly tried as a radiosensitizer. Misonidazole is a 2-nitroimidazole; its structure is shown in Figure 26.4.
Misonidazole produces appreciable sensitization with cells in culture (Fig. 26.5). Hypoxic cells in 10 mM of misonidazole have a radiosensitivity approaching that of aerated cells. Misonidazole also has a dramatic effect on tumors in experimental animals. This is illustrated in Figure 26.6, which shows the proportion of mouse mammary tumors controlled as a function of x-ray dose delivered in a single fraction. If x-rays are used alone, the dose required to control half of the tumors is 43.8 Gy. This falls to 24.1 Gy if the radiation is delivered 30 minutes after the administration of misonidazole (1 mg/g body weight). This corresponds to an enhancement ratio of 1.8. Dramatic results, such as those shown in Figure 26.6 in which an enhancement ratio of 1.8 was obtained, are rather misleading; they represent single-dose treatments, in contrast to the multifraction regimens common in conventional radiotherapy. Most animal tumors reoxygenate to some extent between irradiations, so in a multifraction regimen, the enhancement ratio for a hypoxic cell sensitizer is usually much less than for a single-dose treatment.
After encouraging results in laboratory studies, misonidazole was introduced into a large number of clinical trials, involving many different types of human tumors, in Europe and the United States. In general, the results have been disappointing. Of the 20 or so randomized prospective controlled clinical trials performed in the United States by the Radiation Therapy Oncology Group (RTOG), none yielded a statistically significant advantage for misonidazole, although a number indicated a slight benefit. The only trial that shows a clear advantage for misonidazole was the head and neck cancer trial performed in Denmark, the largest single trial performed
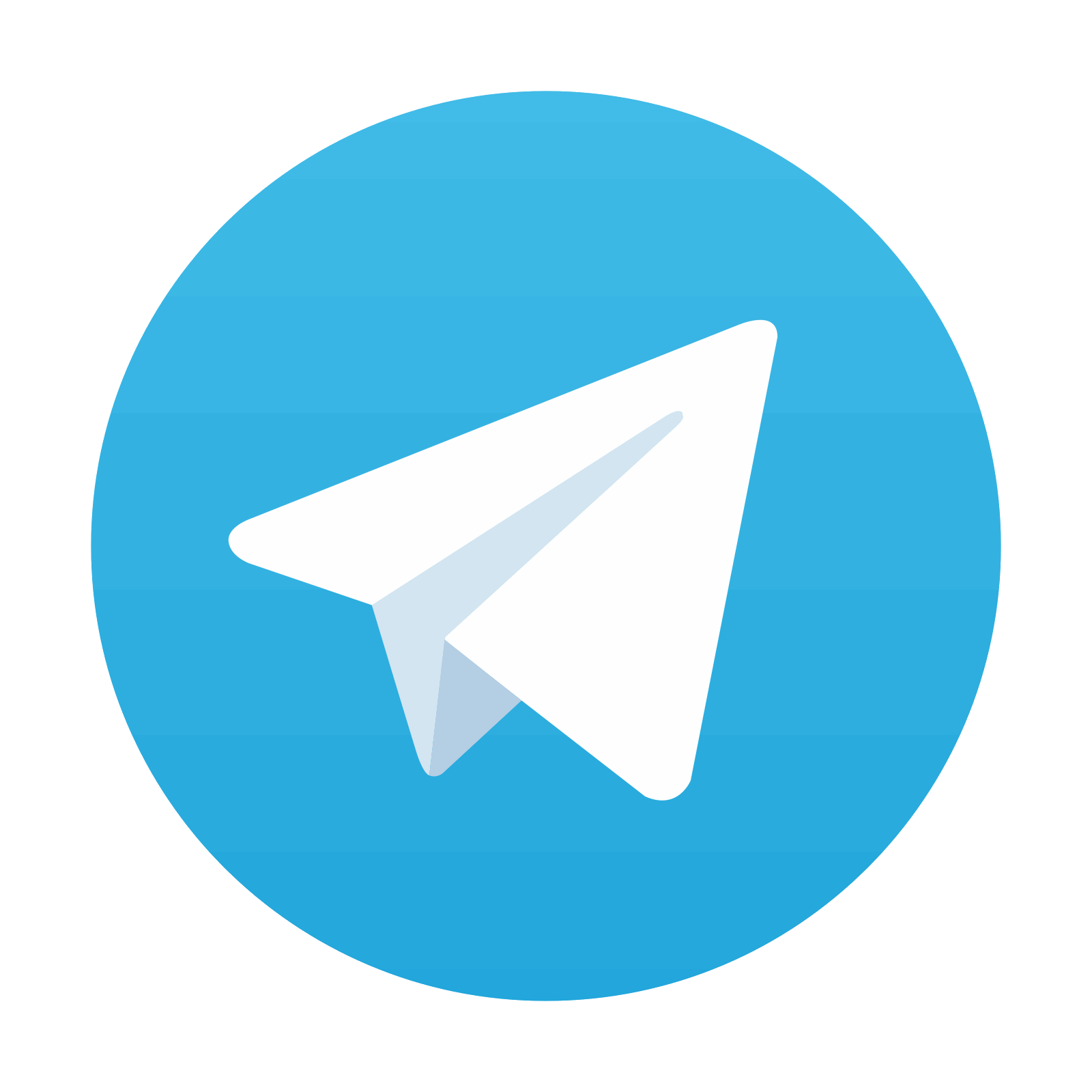
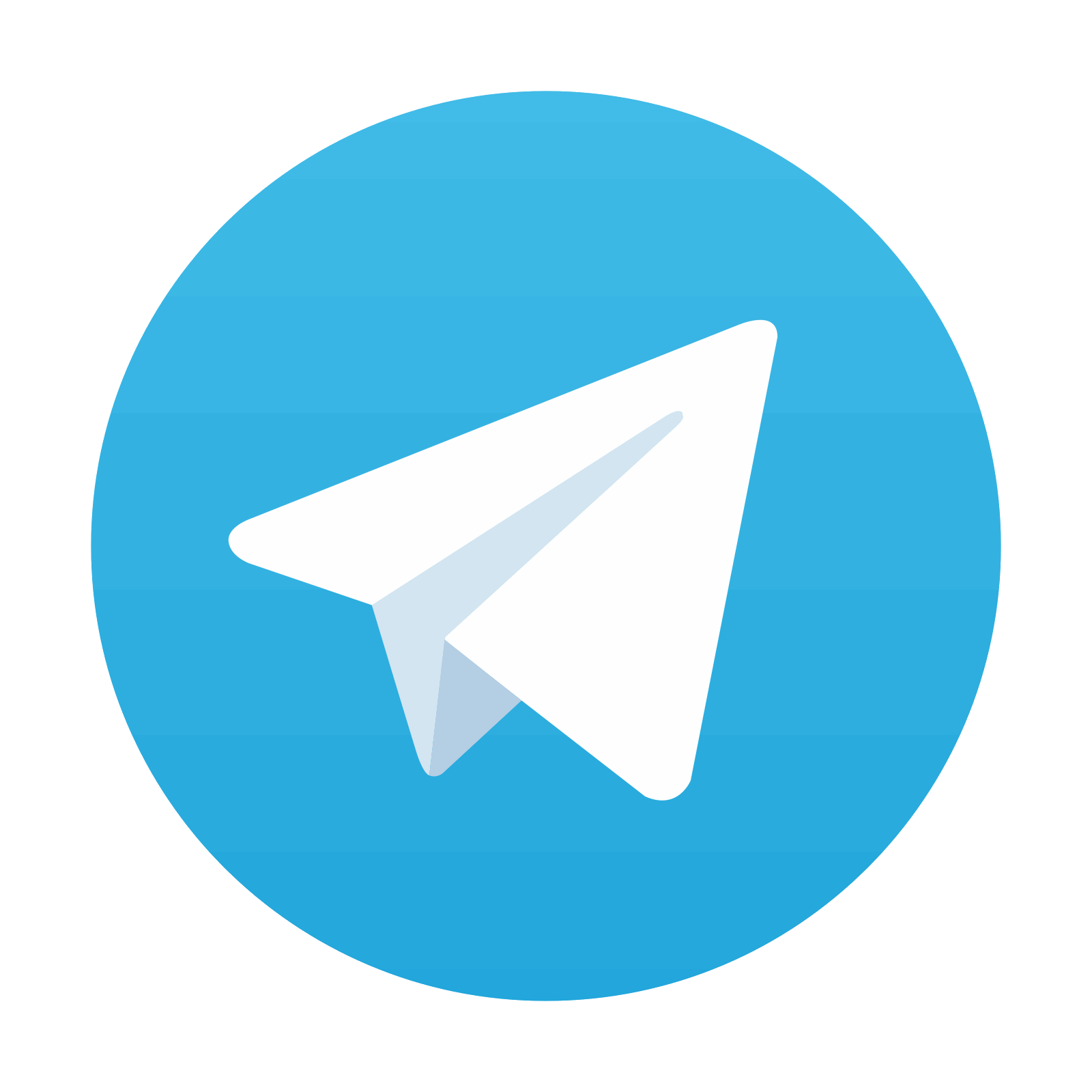
Stay updated, free articles. Join our Telegram channel

Full access? Get Clinical Tree
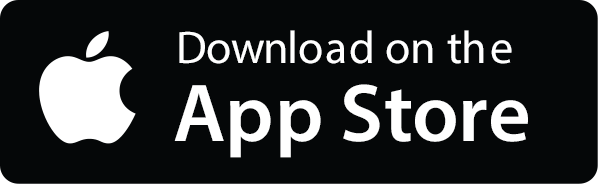
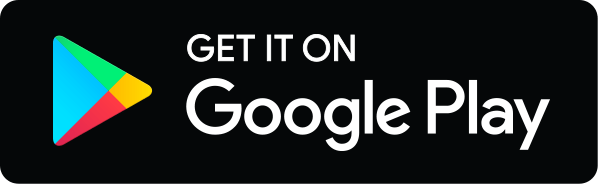
