Fig. 21.1
Photo of neurovascular surgery performed in an image-guided therapy radiology suite at the Hospital for Sick Children, Toronto, ON, Canada (With permission, Ellis et al. [9])
Magnetic Resonance Imaging
Rapid progress in MR technology now arms the neurosurgeon and interventional neuroradiologist with exceptional high-resolution multi-planar soft tissue images, three-dimensional (3D) reconstructions, 4D imaging of the cerebral and spinal vasculature (with time resolution), selective imaging of the arterial and venous anatomy (MR angiography, MR venography), dynamic imaging of brain function and blood flow (blood oxygen dependent and arterial spin label fMRI, MR perfusion), and white matter connectivity imaging (diffusion tensor imaging). In addition to fueling parallel advances in frameless stereotaxy and neuronavigation, MR has also become the premier intraoperative imaging modality for image-guided therapy, since the first case performed at the Brigham and Women’s Hospital (Boston, MA) in 1986 (a craniotomy for resection of a cerebral cavernous malformation). Nowhere has this been more evident than in the field of neurooncology, in which intraoperative MR systems permit safer and more complete resection of brain tumors with preservation of adjacent brain structures.
Consideration of HNOR design requires a thorough appreciation of the strengths and benefits of available system configurations. While a complete discussion is beyond the scope of this chapter, current intraoperative MR systems can be broadly divided into closed and open systems. Closed MR systems refer to conventional MR systems similar to those in diagnostic radiology suites, housed in the operating room environment. Such systems are characterized by higher magnetic strength (typically 1.5–3 T), higher image resolution, and the ability to obtain more complex sequences such as diffusion-weighted imaging (DWI), MRA, MRV, and DTI. With earlier versions of closed systems, surgical procedures had to be undertaken outside the 5 G line, requiring procedural disruption and a short transfer distance, of either the patient or the scanner, for image acquisition. However, newer systems allow access to the patient’s head through an opening in the back of the scanner, limiting these disadvantages [12]. Such a configuration has been used at Children’s Hospital Boston, MA, since 2005, where it has facilitated over 500 image-guided neurosurgical procedures in children (Fig. 21.2). The main drawback of the closed system is an inability to perform real-time, MR-guided procedures.
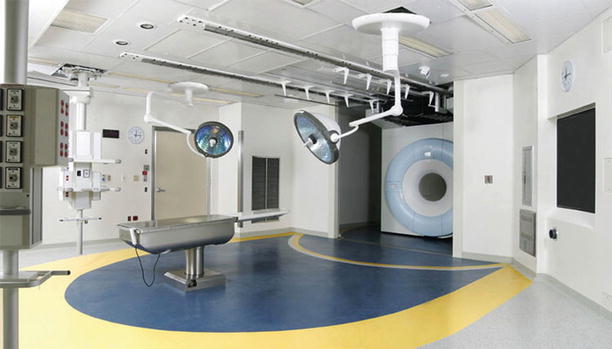
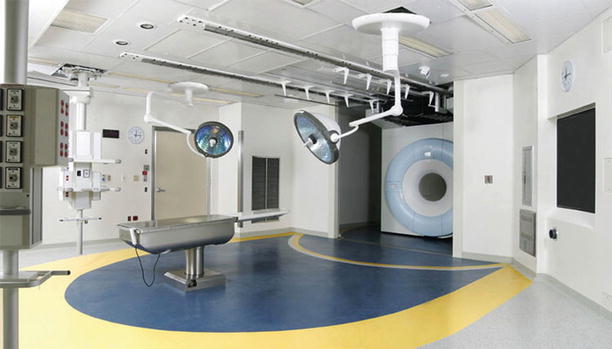
Fig. 21.2
Photo of closed bore intraoperative magnetic resonance imaging facility at Children’s Hospital Boston, Boston, MA, USA
Open MR systems, on the other hand, overcome this barrier with the use of superconducting magnet systems and enhanced data processing systems that permit neurosurgical procedures, such as tumor resection, tumor biopsy, and aspiration procedures, to be completed under live MR-guided neuronavigation within the magnetic field [13]. These units feature either a vertically or horizontally aligned working space, along with specially designed coils positioned around the patient’s head, allowing continuous access to the patient during image acquisition. An open, vertically oriented, 0.5 T system was installed at the Brigham and Women’s Hospital, Boston, MA, in 1986 [14] and has since been used in over 3,000 surgical and interventional procedures (unpublished data, Fig. 21.3). Major drawbacks of the open MR system include limited operating table positioning, the need for strict use of non-ferromagnetic instruments during the procedure, and weaker strength magnets fields, resulting in longer imaging time and inferior image quality compared to closed systems and the inability to obtain complex anatomical and functional images [12]. When selecting an intraoperative MR system, advantages and disadvantages of each configuration must be weighed in light of the target patient population and treating physicians. Ultimately, current configurations require a significant trade-off between patient access and image quality.
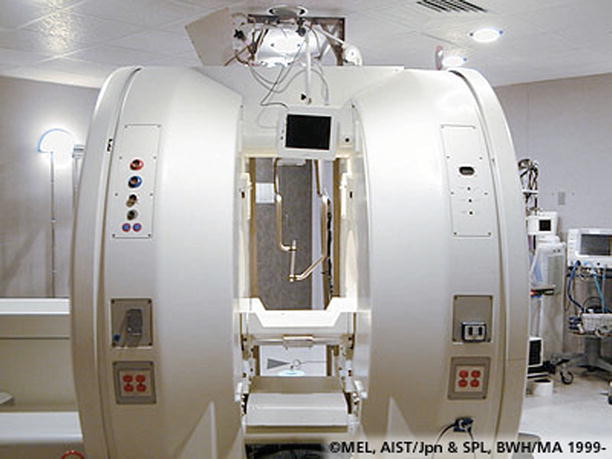
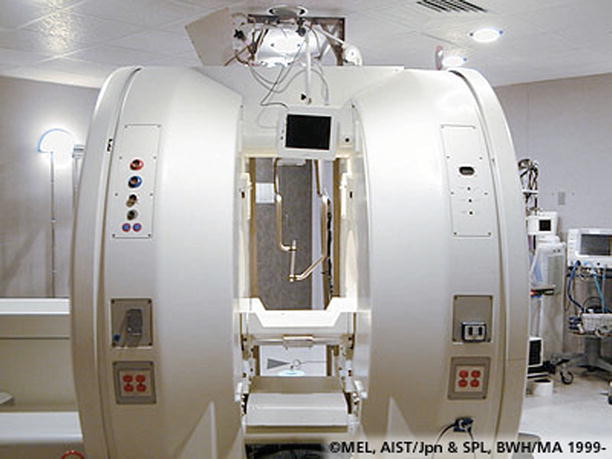
Fig. 21.3
Photo of open-bore intraoperative magnetic resonance imaging facility at Brigham and Women’s Hospital, Boston, MA, USA
Ultrasound
Intraoperative ultrasound has long been a valuable adjunct for a variety of neurosurgical procedures. Major advantages of ultrasound include ease of use, relatively low cost, and the ability to provide rapid, real-time assessment of intracranial and spinal anatomy and providing an important complement to frameless sterotaxy (which relies on preoperative imaging) for surgical procedures. Despite the drawbacks of low signal-to-noise ratios and poor contrast resolution, intraoperative ultrasound can be used to help localize and assess the resection of subcortical vascular lesions such as brain tumors and cavernous malformations, as well as provide real-time guidance for simple procedures such as cyst aspiration or ventriculostomy placement. The addition of Doppler ultrasonography can also be helpful in identifying neurovascular pathology such as AVMs, aneurysms, and dural AV fistulae as well as assessing flow in normal cerebral and spinal vasculature. New advances in 3D ultrasound are now beginning to show promise in integrative neuronavigation platforms, helping to account for challenges such as intraoperative brain shift [15]. Ultrasound is also indispensible for obtaining vascular access, particularly in neonates and infants.
Indocyanine Green Neuroangiography
One of the more recent advances in intraoperative imaging for neurovascular surgery is indocyanine green (ICG) neuroangiography, which can provide non-catheter-based, real-time imaging of the cerebral and spinal vasculature during surgery. This technique involves intravenous bolus administration of ICG, a near-infrared tricarbocyanine dye which fluoresces when the operative field is illuminated by a light source with a wavelength within the ICG absorption band. As the ICG dye travels through the exposed vasculature, fluorescence is induced and recorded in real time by a high-resolution video camera as the dye passes through the arterial, capillary, and venous tributaries. The technique is fully microscope-integrated and provides important intraoperative confirmation of the cure of aneurysms, AVMs, and dural AV fistulae, along with preservation of normal vasculature [16–18]. In one report, ICG neuroangiography helped guide intraoperative management of three paraclinoid aneurysms during endovascular retrograde suction decompression, illustrating its potential use in the HNOR environment [19]. The major drawbacks of ICG neuroangiography is the inability to evaluate flow in vessels that are not within the line-of-sight of the light source and camera, ultimately rendering this technique a useful adjunct, but not a substitute for conventional catheter angiography.
Neuronavigation
Over the past few decades, neurosurgeons have benefitted from frameless neuronavigation platforms to aid in the localization of important neuroanatomical structures in 3D during surgery. Information from preoperative CT, CT angiography, MRI, and MRA can be can imported into the system and fused to generate an integrated 3D working reference model that is co-registered to the patient during surgery. In addition to anatomic information, fMRI, DTI, and magnetoencephalography (MEG) data can also be imported to aid in the delineation of functionally eloquent brain regions and associated white matter tracts and clarify their relation to vascular malformations. Studies have shown that the incorporation of DTI imaging into such platforms is associated with increased resection rates and decreased morbidity in brain tumor surgery [20], but this approach has yet to be widely applied to patients with vascular lesions. The primary limitation of current neuronavigation systems is their inability to account for brain shift and anatomical distortion once the cranial vault is violated and the brain manipulated, leading to diminishing spatial accuracy as time progresses during surgery. Fortunately, intraoperative imaging such as MRI can now provide updated information that can be easily incorporated into the neuronavigation system to help maintain spatial accuracy as the procedure continues.
A second limitation of current neuronavigation systems, particularly as they relate to neurovascular surgery, is the inability to incorporate images acquired from catheter angiography (such as 3D angiography) into neuronavigation platforms. Undoubtedly, future iterations of integrated neurovascular navigation platforms will allow fusion of updated intraoperative angiographic and cross-sectional imaging to help facilitate the localization and resection of vascular lesions such as AVMs.
The Role of the HNOR
Over the past few years, several reports of hybrid or multimodality image-guided neurosurgery operating rooms have begun to explore and expand the potential role of these facilities [21, 22]. In our opinion, the HNOR should provide a complementary venue for the integration of microsurgical and endovascular techniques and the application of up-to-date advances in neuroimaging and image guidance and ultimately overcome the current deficiencies of the separated conventional operating room and angiography suite. Ideally, the HNOR should (1) enable the comprehensive microsurgical and endovascular management of neurovascular disorders in one facility; (2) allow easy and immediate conversion to open microsurgical management under conditions when endovascular therapy is attempted but deemed unfeasible (i.e., uncoilable aneurysms); (3) facilitate combined endovascular/microsurgical approaches to complex conditions (i.e., surgical clipping of a giant paraclinoid aneurysm with proximal control obtained with endovascular balloon inflation); (4) support staged endovascular/microsurgical approaches (i.e., partial presurgical endovascular liquid embolization of an AVM followed by surgical resection); (5) provide access to high-quality, gold standard intraoperative imaging to help document cure or guide additional targeted therapy (i.e., post-AVM resection angiography fused with intraoperative contrast-enhanced MR to guide the resection of residual AVM); (6) expedite the detection and immediate management of unrecognized intraoperative complications (i.e., allowing immediate readjustment of an aneurysm clip that has inadvertently occluded the parent artery, as detected on intraoperative angiography); and (7) offer the ideal venue for incorporation of future image-guided therapeutic techniques (i.e., MR-guided focused ultrasound therapy for AVMs). The facility may also be further incorporated into institutional emergency stroke or subarachnoid hemorrhage/intracranial hemorrhage protocols, providing high-quality diagnostic evaluation followed by immediate therapeutic intervention at the same sitting (i.e., MRI detection of an evolving MCA infarct secondary to proximal M1 occlusion followed by immediate endovascular mechanical thrombectomy).
Designing the HNOR
As mentioned above, HNOR design should be individually tailored to the needs and demands of the target patient population and the treating multidisciplinary neurovascular team. The combined suite should meet the standards of both an operating room and an angiography suite, facilitating microsurgical, endovascular, or combined approaches, as well as seamless transition and conversion between these approaches. Without compromising the facility’s primary purpose, consideration must also be given to other radiological, interventional, or surgical subspecialty groups that may use the facility on a part-time basis. No less importantly, radiology technologists, nursing staff, and other operating personnel should be consulted as well, as the efficiency and efficacy of this hybrid venue will ultimately rest on the coordinated efforts of a well-trained team operating in an environment that is as user-friendly as possible. Additionally, close collaboration and the exchange of ideas between the operating staff and experienced leaders of the neuroimaging and medical device communities is likely to be valuable. Given that neurovascular disease accounts for only a fraction of the neurosurgical and interventional neuroradiology procedures performed in any tertiary hospital, it would be optimal for the HNOR to be suited for more common neurosurgical indications such as brain tumors, epilepsy, spinal disorders, and hydrocephalus as well. Considerations for hybrid neurovascular OR design can be divided into three categories: general considerations, neurovascular-specific considerations, and neuroimaging modality considerations.
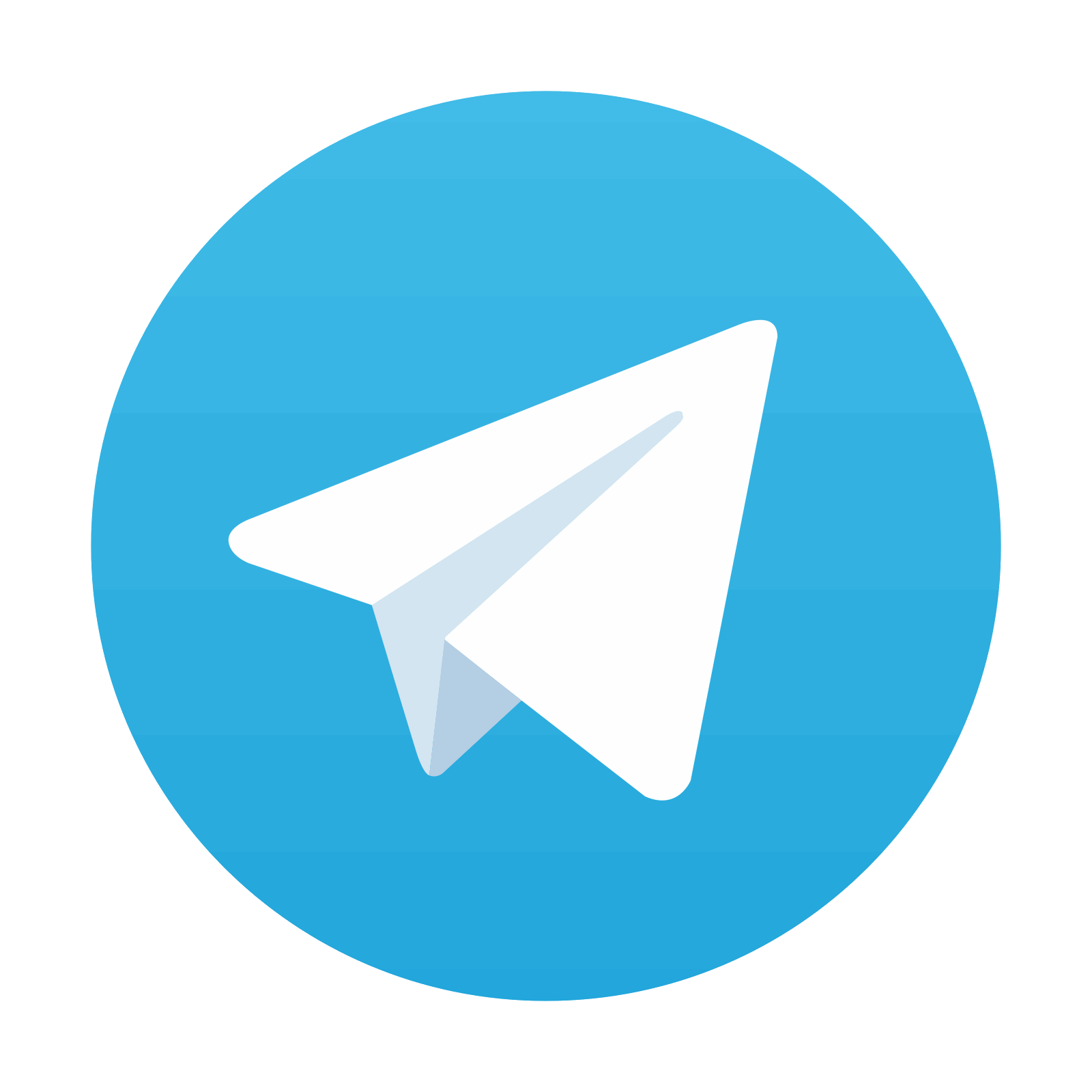
Stay updated, free articles. Join our Telegram channel

Full access? Get Clinical Tree
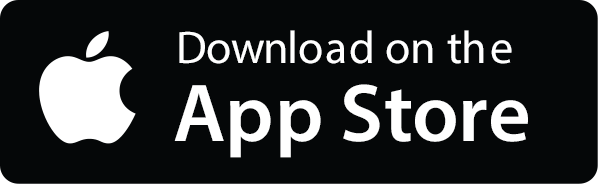
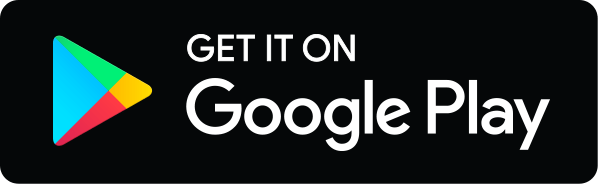