Fig. 16.1
The relative distribution of radiation dose in the treatment of esophageal cancer with 3-dimensional conformal radiation therapy (3D CRT) versus intensity-modulated radiation therapy (IMRT)
Thus, when optimal beam arrangements are used, IMRT can substantially improve sparing of organs at risk (OARs) relative to conformal techniques. Moreover, the technology used to deliver IMRT can produce further improvements in conformality and dose delivery. In one treatment planning comparison study of 10 patients with upper thoracic esophageal tumors, the use of “micro” 4-mm-wide multileaf collimators (MLCs) was compared to the use of standard 10-mm-wide MLCs. The 4-mm MLCs were more efficient, using fewer monitor units (MUs) than did the standard 10-mm MLCs (mean 703 vs. 833 MUs). Dose coverage was also improved, as was sparing of the spinal cord and lung [17]. Other studies have explored the use of volumetric modulated arc therapy (VMAT, also known as RapidArc), a specialized IMRT delivery system in which the radiation is delivered while the gantry of the linear accelerator is being rotated through one or more arcs. This application can be used to further improve OAR sparing over that which is possible with 3D CRT or fixed-field IMRT and significantly reduces the “beam-on” time [1, 19, 31, 36, 49, 57]. One treatment planning study that compared single-arc and double-arc VMAT plans to those for 4-field IMRT or 3D CRT showed that the IMRT and VMAT plans improved both OAR sparing and dose conformality over that possible with the 3D CRT plans; also, the double-arm VMAT plan further enhanced OAR sparing while also reducing beam-on time compared with the single-arc VMAT plan [49]. These improvements may be further enhanced with the use of flattening filter-free RapidArc plans. In a study comparing this technique with standard RapidArc therapy, IMRT, and 3D CRT, all of the IMRT plans showed better sparing of the lung compared with 3D CRT, but the flattening filter-free RapidArc therapy showed a 20 % increase in MU/Gy, a 90 % increase in average dose rate, and a 20 % reduction in beam-on time [36]. The use of IMRT delivered by helical tomotherapy has also been reported in several treatment planning studies, and the dose distributions are quite similar to those of the double-arc RapidArc plans [6, 33, 35].
16.4 Clinical Evidence in Support of IMRT
As alluded to in the previous section, many treatment planning studies have demonstrated potential dosimetric advantages from IMRT in terms of improving avoidance of normal tissues and the efficiency of radiation treatment; however, clinical evidence of such benefits is only recently emerging from a few single-institution reports. No large randomized trials have been undertaken to directly compare IMRT with 3D CRT, and indeed, such studies are unlikely. Although 3D CRT remains the standard of care in many parts of the world, IMRT is increasingly being used at large academic centers and in many community practices. Several small studies have shown that IMRT for esophageal tumors is feasible and has promising long-term outcomes and good tolerability [27, 52]. A small randomized trial was conducted in China comparing IMRT versus 3D CRT in 60 patients [29]; the radiation dose was 64 Gy in 30 fractions and was given with concurrent cisplatin and docetaxel. Although the 1- to 3-year survival rates seemed to favor IMRT, the numbers were too small to reach statistical significance. MD Anderson Cancer Center reported a large retrospective analysis that spanned 1998–2010; 3D CRT was more common in 1998–2002, and IMRT was more common in 2002–2010 [30]. Because of the substantial potential for bias in this nonrandomized comparison, propensity-matched analysis was used with an inverse probability weight approach to balance the two treatment era groups for 13 potential confounding patient- and treatment-related characteristics. Imbalances noted were that patients given IMRT were less likely to have received induction chemotherapy (35.7 % in the IMRT group vs. 46.7 % in the 3D CRT group), had worse performance status scores (≤80 in 66 % of IMRT vs. 50 % of 3D CRT), were more likely to have undergone positron emission tomography (PET) with fluorodeoxyglucose (FDG) as part of the initial disease-staging workup (95 % vs. 55 %), and had higher levels of predicted forced expiratory volume in 1 second (FEV1) (96 % vs. 90 %). Clinical outcomes, evaluated after inverse probability weight adjustment and Cox logistic regression, showed that IMRT produced significantly better overall survival and locoregional control but not cancer-specific survival or distant metastasis-free survival. To determine why overall survival but not cancer-specific survival was better in the IMRT group, cause-specific survival was compared between groups, specifically evaluating deaths from cancer, pulmonary or cardiac disease, or other causes (defined as deaths that were not related to cancer but were unknown). Cancer-related deaths accounted for most of the deaths in these patients, accounting for >40 % of deaths during the long-term follow-up period (median 40.3 months for the IMRT group and 82.4 months for the 3D CRT group). No differences were found in cancer-specific death or pulmonary death between groups, but the cumulative risks of cardiac-related mortality and other death were strikingly higher in the 3D CRT group than in the IMRT group, with nearly three times the risk of dying from causes not related to cancer (Fig. 16.2). To determine if this observation could be generalized to other populations, these investigators evaluated two large populational databases, the United States Surveillance, Epidemiology, and End Results (SEER) and the Texas Cancer Registry-Medicare databases. Inclusion criteria included having nonmetastatic esophageal cancer, being older than 65 years, and having been treated in 2002–2009 with either conformal radiation or IMRT. A total of 2,578 patients were identified who met these criteria, 2,265 who received conformal radiation and 313 IMRT. The two groups were well balanced in age, sex, race, disease stage (localized or regional), tumor grade, practice location, Charlson comorbidity index (CCI), use of chemotherapy, use of surgery, and various underlying cardiac and pulmonary diseases; the only differences were in marital status and SEER geographic region. On average, 85 % of patients had had chemotherapy with radiation, and 18 % had surgical resection. The rate of IMRT use increased over time from 2.6 % in 2002 to 30 % in 2009, and the use of 2D or 3D radiation therapy decreased from 97.4 % in 2002 to 69 % in 2009. All-cause mortality was significantly lower in the IMRT group (52.4 % vs. 74.5 %, p < 0.0001). Cause-specific mortality also revealed that IMRT led to lower rates of esophageal cancer-specific death (40.3 % vs. 55.6 %, p < 0.0001) and cardiac-related mortality (1.6 % vs. 5.3 %, p = 0.0043), but not pulmonary-specific death (0.96 % vs. 1.55 %, p = 0.419) or all other non-cancer deaths (9.6 % vs. 12.1 %, p = 0.204). On multivariable analysis, not being married (HR 1.17, p = 0.02), having regional vs. localized disease (HR 1.29, p < 0.0001), having a Charlson comorbidity index of 2+ (HR 1.21, p = 0.01), receiving surgery (HR 0.52, p < 0.0001), receiving chemotherapy (HR 0.57, p < 0.0001), and living in the New Jersey region (HR 0.73, p = 0.002) predicted cancer-specific mortality, but receipt of IMRT (rather than 3D CRT) did not. However, other multivariable analyses showed that IMRT (HR 0.39, p = 0.047) and comorbidity index 2+ (HR 3.9, p < 0.0001) were the only significant predictors of for cardiac-related mortality [32].
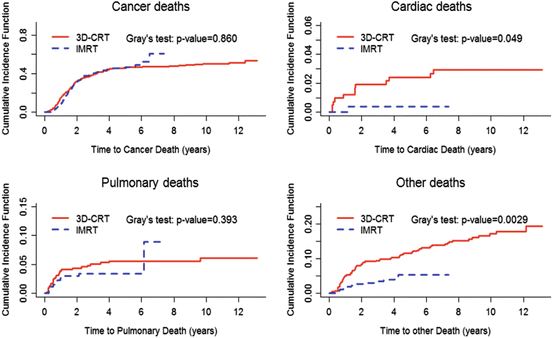
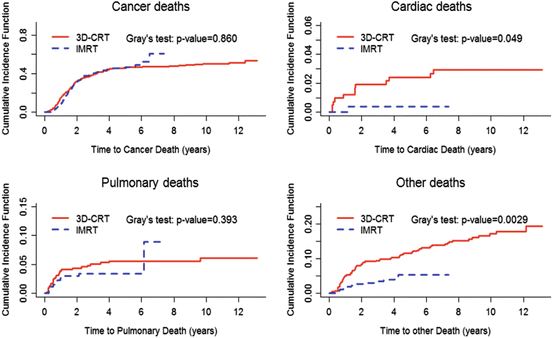
Fig. 16.2
The cumulative risk of cause-specific death after 3D CRT versus IMRT for esophageal cancer. Cancer and pulmonary deaths were not significantly different between IMRT and 3D CRT; however, for cardiac and other deaths (undefined deaths), rates of deaths were significantly higher in patients treated with 3D CRT (Reprinted with permission from [30])
Because postoperative complications are well known to be related to radiation exposure of OARs, MD Anderson investigators further studied whether postoperative complications could be improved with the use of IMRT relative to 3D CRT. In the subgroup of 444 patients who received preoperative chemoradiation followed by surgical resection between 1998 and 2011, 208 had received 3D CRT, 164 patients IMRT, and 72 patients proton beam therapy (PBT; use of PBT began at MD Anderson in 2006). Postoperative complications evaluated were pulmonary, gastrointestinal, cardiac, and wound-healing complications [53]. Although radiation was not associated with cardiac or wound-healing complications, significant differences were seen in pulmonary and gastrointestinal complications: IMRT was associated with a substantial reduction in risk of both gastrointestinal (odds ratio [OR] 0.57, 95 % CI [confidence interval] 0.34–0.93) and pulmonary (OR 0.50, 95 % CI 0.27–0.91) complications compared with 3D CRT, but no differences were found between IMRT and PBT (with the possible exception of IMRT being more likely to produce pulmonary complications [OR 1.56, 95 % CI 0.68–3.60]). Actuarial rates of pulmonary complications were 34 % for the 3D CRT group, 23 % for the IMRT group, and 14 % for the PBT group. The Lyman-Kutcher-Burman modeling showed that the MLD was the strongest predictor of pulmonary toxicity (Fig. 16.3a); indeed, after MLD was added to the multivariate model, the link between radiation modality and pulmonary toxicity disappeared. Superimposing the MLD of each patients treated with each modalities revealed that pulmonary complications were driven not by the type of radiation used but by the ability of each of the modalities to achieve lower MLD (Fig. 16.3b). PBT produced the lowest MLD and IMRT a low-to-intermediate range MLD, and 3D CRT was associated with intermediate-to-high MLD. These findings strongly link MLD with the percent probability of pulmonary complications [53]. The rates of pulmonary and gastrointestinal complications associated with 3D CRT in this study were similar to those reported in the literature, but were much lower among patients treated with either IMRT or PBT (Table 16.1).
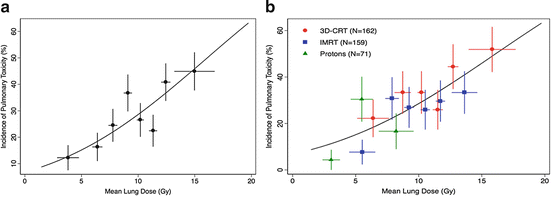
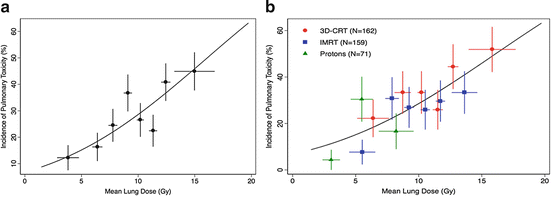
Fig. 16.3
LKB modeling of pulmonary toxicity in relation to mean lung dose and radiation modality. (a) The probability of developing postoperative pulmonary toxicity is related to the mean lung dose (MLD). (b) The relationship between incidence of postoperative pulmonary toxicities and MLD in the context of radiation modality (Reprinted with permission from [53])
Table 16.1
Studies of pulmonary and gastrointestinal toxicity after surgery for esophageal cancer
Study | Pulmonary | Gastrointestinal |
---|---|---|
[50] | 46.40 % | 3.5 % † |
[8] | 13.6 %* | 24.40 % |
[46] | Not reported | 14.9 % † |
[44] | 33.30 % | 20.80 % |
[9] | 45.00 % | 14.0 %† |
[47] | 46.00 % | 22.3 %† |
[53] | 25.2 % (overall) | 23.0 % (overall) |
30.3 % (3D CRT) | 28.4 % (3D CRT) | |
23.8 % (IMRT) | 18.3 % (IMRT) | |
13.9 % (PBT) | 18.1 % (PBT) |
16.5 Treatment Planning for Chemoradiation
Investigators at MD Anderson take a multidisciplinary approach to treating esophageal cancer. Each case is discussed at multidisciplinary tumor board meetings, and eligibility for various ongoing clinical trials is considered. After a complete staging workup that includes FDG-PET/CT and esophagoduodenoscopy and endoscopic ultrasonography with biopsy, all patients with nonmetastatic disease are treated with chemoradiation, to be followed by an evaluation to determine surgical resectability. That workup also includes FDG-PET to rule out metastatic disease and endoscopy and biopsy to identify any local residual disease.
For treatment planning, 4D CT-based treatment simulation is used for all patients. This technique assesses diaphragmatic motion of the gastroesophageal junction, where most tumors are located, and that motion is accounted for in the treatment planning, along with findings from pretreatment PET scans and ultrasonography. Outlines of the gross tumor volume (GTV) and the path of its motion (iGTV) are contoured on the axial slices of maximum intensity projection (MIP) images, with the margin expanded by 4 cm superiorly and by 3 cm inferiorly, into the lesser curvature of the stomach. If nodal disease is present in the left gastric nodes along the lesser curvature of the stomach, generally, those nodes are included in the iGTV, but the internal clinical target volume (iCTV) will extend down to encompass the celiac axis nodes because of the risk of regional recurrence in those nodes. Laterally, the iCTV encompasses all of the soft tissues surrounding the esophagus up to the parietal pleural surface and avoids all anatomic boundaries (e.g., aorta, vertebral body, and the inferior border of the heart). The planning target volume (PTV) is a uniform expansion of 0.5 cm around the iCTV to account for setup errors and is used only if daily kV or MV projection imaging is used for daily image-guided setup. If daily imaging is not performed, the PTV should be a 1-cm expansion from the iCTV. The standard radiation dose used is 50.4 Gy in 28 fractions, although other regimens (e.g., 40 Gy in 20 fractions, 41.4 Gy in 23 fractions, or 45 Gy in 25 fractions) are also acceptable. The most common field arrangement is a 5-field IMRT approach, with 5 posteriorly placed beams without any beams directly entering anteriorly through the heart. In light of the potential advantages of VMAT or RapidArc IMRT in terms of workflow efficiency, these techniques are also being implemented. In terms of dosimetric (dose-volume histogram or DVH) limits, the total lung V10 is kept at <40 %, lung V 15 < 30 %, and lung V 20 < 20 %, particularly for patients who will go on to undergo surgery, to avoid postoperative pulmonary complications. The heart V 40 is generally kept at <40 %, but considerably lower doses can generally be achieved. The most common chemotherapy regimen used concurrently with IMRT is cisplatin with fluorouracil, although other regimens can also be used, as none have been shown to have particular advantages. Per the CROSS trial, carboplatin (AUC 2.0) and paclitaxel (50 mg/m2) can also be considered a standard chemotherapy regimen.
16.6 Conclusions
Radiation is a crucial component of the management of esophageal cancer. Based on findings from recent randomized trials and meta-analyses, trimodality therapy (chemotherapy, radiation, and surgery) can be considered the standard of care. For patients who cannot tolerate surgery, chemoradiation is the definitive treatment, with long-term cure rates of around 20 %. Treatment of esophageal tumors, particularly those that arise in the mid- to distal locations, can cause significant morbidity and postoperative complications such as pleural and pericardial effusions owing to the proximity of the heart and lung. The use of IMRT can improve dose conformality to the target and also reduce the dose to the OARs. The clinical experience at MD Anderson, in which large numbers of patients have been treated with IMRT or 3D CRT, has demonstrated significant improvements for IMRT in overall survival and reduced cardiac mortality as well as in postoperative pulmonary and gastrointestinal complications. A recent population-based analysis of SEER-Medicare data further supports a benefit from IMRT in terms of improved survival, not from a reduction in cancer-specific death but rather from a reduction in risk of cardiac mortality compared with 3D CRT. Given the unlikeliness of a large randomized trial that directly compares IMRT with 3D CRT, the findings reviewed in this chapter should provide sufficient evidence to support the use of IMRT for esophageal cancer.
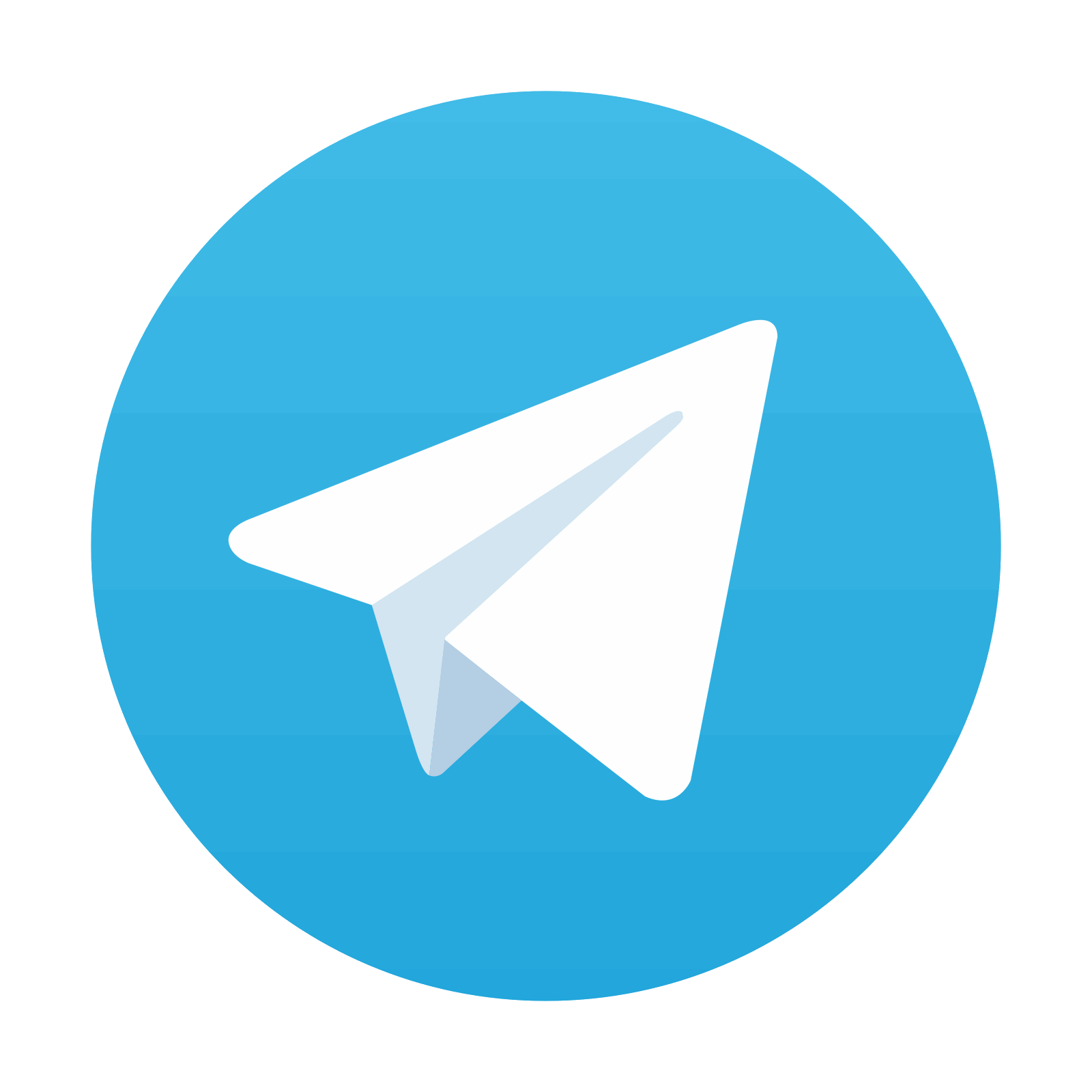
Stay updated, free articles. Join our Telegram channel

Full access? Get Clinical Tree
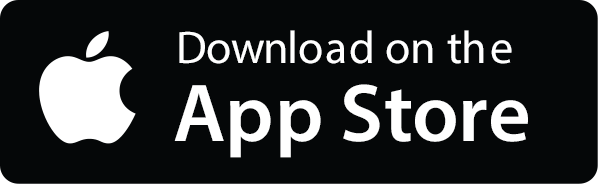
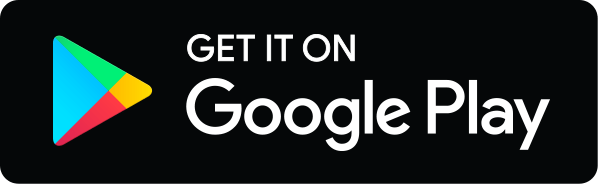