Three-dimensional Conformal Brachytherapy: Current Trends and Future Promise
Jeffrey F. Williamson
Robert A. Cormack
The growing utilization of three-dimensional (3D) imaging in planning, evaluation, and delivery of brachytherapy has had substantial impact in clinical practice, perhaps more than any other technical development since the introduction of artificial radionuclides.1 Using x-ray computed tomography (CT) and other 3D imaging modalities to delineate clinical target volumes (CTV) and organs at risk (OAR) relative to the implanted sources and anatomy-based coverage indices to evaluate dose distributions has the potential to significantly improve treatment outcomes. The image-based brachytherapy approach (Fig. 7.1) is a radical departure from the time-honored surgical paradigm in which applicators and sources are guided into position through direct surgical exposure and visualization or palpation of the target tissue. Treatment plans for conventional surgically guided implants are based on reference points and other dose-specification quantities defined relative to the implanted sources.
Early reports of 3D imaging in brachytherapy planning include analogue transverse axial tomography for interstitial implants,2 B-mode ultrasound (US) for intracavitary implant dosimetry,3,4 and soon after its introduction in 1974, application of x-ray transmission CT to both interstitial5,6 and intracavitary brachytherapy planning.7 Stereotactically guided interstitial implants for malignant gliomas were introduced in the early 1980s.8,9 They were one of the first (and to date, one of the most sophisticated and accurate) approaches using a combination of two-dimensional (2D) and 3D image guidance to accurately position radioactive sources at preplanned locations relative to a CTV delineated from a preoperative 3D imaging study. Some of the earliest 3D image-based planning software was developed for this clinical application.10
The largest scale application of image-guided brachytherapy, transrectal US (TRUS)-guided brachytherapy, was introduced in the early 1980s by Holm et al.11 (Fig. 7.1B). TRUS-guided perineal implant techniques were pioneered by Blasko et al.12 for permanent implantation and by Mate et al.13 and Martinez et al.14 for high dose rate (HDR) delivery. One of the remarkable trends of the last decade is the growing dominance of TRUS-guided brachytherapy for low- and intermediate-risk prostate cancer. Ten-year survival data15,16 demonstrate that brachytherapy, as sole treatment for low-risk patients or in combination with external-beam radiation for higher risk disease, results in biochemical relapse-free survival rates comparable to or superior to radical prostatectomy and definitive external-beam therapy. For low-activity palladium-103 (103Pd) or iodine-125 (125I) permanent implantation, the attraction of a 1-day procedure along with a favorable profile of normal tissue complications has resulted in exponential growth of this modality. The number of procedures has grown from less than 5,000 in 1995 to 40,000 to 60,000 in 2002,17 approximately 30% to 40% of all eligible patients diagnosed annually in the United States, challenging radical prostatectomy as the standard of treatment.
The goal of this chapter is to provide a snapshot of the rapidly evolving field of 3D conformal brachytherapy (3DCBT), a broad category that we define to include both image-based planning and image-guided insertion techniques. In addition, this chapter defines basic terminology and outlines a taxonomy for classifying the broad spectrum of 3DCBT processes. The state of 3DCBT for prostate cancer is also reviewed. Finally, the chapter concludes with brief discussions of 3DCBT techniques in cervical cancer treatment and the scientific and clinical challenges confronting 3DCBT.
VARIETIES OF THREE-DIMENSIONAL CONFORMAL BRACHYTHERAPY
We define 3DCBT as any brachytherapy procedure in which 3D imaging is used to visualize source/applicator locations in relation to the patient’s 3D anatomy. Using 2D x-ray imaging techniques is not 3DCBT unless a rigorous method exists for inferring source positions in the 3D image frame of reference from 2D projections, as in the case of stereotactic brain implants. The term 3DCBT indicates that its goal is to improve conformality of the dose distribution with respect to
the target volume. 3DCBT can involve a single modality (e.g., CT, which is used both to localize the sources and visualize anatomy) or the fusion of two or more forms of imaging (e.g., x-ray fluoroscopy to evaluate source positions fused with 3D TRUS images to visualize soft tissue).18 Treatment position (lithotomy insertion position, supine treatment position, or preoperative volume-study position) is also an important variable. As Figure 7.2 indicates, 3DCBT can take the form of image-based brachytherapy planning (IBBP), in which the 3D dose distribution or source positions are in registration with 3D segmented structures, or image-guided brachytherapy (IGBT), in which intraoperative 3D images provide feedback to the brachytherapist during the source or applicator insertion process. IBBP can be separated from the insertion procedure (e.g., preplanning or postoperative dose evaluation), or it can be performed intraoperatively and even used as a form of image guidance, which the American Brachytherapy Society (ABS) guidelines19 refer to as “dynamic dose calculation.” One variable that distinguishes different forms of IGRT is the feedback speed, which is the frequency with which imaging is repeated, allowing the operator to update or correct applicator trajectories or positions during the insertion process. For example, modern 3D TRUS is able to acquire partial volume rotated sagittal images in real time as the operator inserts each needle. One reported20 magnetic resonance imaging (MRI)-guided HDR needle insertion procedure acquires updated MRI images after inserting each group of four to five needles. All IGRT feedback is inherently 3D involving either informally observing or quantitatively measuring proximity (either in geometric space or dose
space) of the applicator trajectory relative to organ boundaries. This excludes purely 2D localization techniques, although sub-3D imaging that supports indirect tracking of applicators through the 3D image space (e.g., stereotactic brain implantation with intraoperative 2D fluoroscopic verification9) satisfies this definition of IGRT.
the target volume. 3DCBT can involve a single modality (e.g., CT, which is used both to localize the sources and visualize anatomy) or the fusion of two or more forms of imaging (e.g., x-ray fluoroscopy to evaluate source positions fused with 3D TRUS images to visualize soft tissue).18 Treatment position (lithotomy insertion position, supine treatment position, or preoperative volume-study position) is also an important variable. As Figure 7.2 indicates, 3DCBT can take the form of image-based brachytherapy planning (IBBP), in which the 3D dose distribution or source positions are in registration with 3D segmented structures, or image-guided brachytherapy (IGBT), in which intraoperative 3D images provide feedback to the brachytherapist during the source or applicator insertion process. IBBP can be separated from the insertion procedure (e.g., preplanning or postoperative dose evaluation), or it can be performed intraoperatively and even used as a form of image guidance, which the American Brachytherapy Society (ABS) guidelines19 refer to as “dynamic dose calculation.” One variable that distinguishes different forms of IGRT is the feedback speed, which is the frequency with which imaging is repeated, allowing the operator to update or correct applicator trajectories or positions during the insertion process. For example, modern 3D TRUS is able to acquire partial volume rotated sagittal images in real time as the operator inserts each needle. One reported20 magnetic resonance imaging (MRI)-guided HDR needle insertion procedure acquires updated MRI images after inserting each group of four to five needles. All IGRT feedback is inherently 3D involving either informally observing or quantitatively measuring proximity (either in geometric space or dose
space) of the applicator trajectory relative to organ boundaries. This excludes purely 2D localization techniques, although sub-3D imaging that supports indirect tracking of applicators through the 3D image space (e.g., stereotactic brain implantation with intraoperative 2D fluoroscopic verification9) satisfies this definition of IGRT.
DIVERSITY OF PROSTATE THREE-DIMENSIONAL CONFORMAL BRACHYTHERAPY TECHNIQUES
Interstitial brachytherapy, either as the sole or boost modality for definitive radiotherapy of prostate cancer, is currently the most frequently practiced indication for brachytherapy in the United States. It is currently the most intensively investigated and developed form of 3DCBT and illustrates the rich diversity of image-based planning and guidance techniques available. Regardless of whether permanent seed implantation or HDR temporary implantation is used, IBBP and image-guided seed and/or applicator positioning has become the standard of practice.
SELECTION OF IMAGING MODALITY
The variety of brachytherapy procedures that can be classified as 3DCBT indicates that imaging may be used in many different ways. Even for a single site such as prostate cancer, imaging is used to support preimplant volume assessment, operative guidance of applicator placement, 3D treatment planning, and postimplant dose evaluation.
Imaging Uses
PREPROCEDURE PREPARATION.
In selecting patients for permanent prostate implantation, imaging is used to assess disease stage, to determine whether disease is confined to the gland,21 and to rule out contraindications such as pubic arch interference via TRUS22 or other volumetric imaging modalities.23 Having elected to perform brachytherapy, a major goal is to predict the quantity of radioactive material necessary to deliver the prescribed dose. The volume study and preplan in current standard of practice (see Conventional Preplanned, Transrectal Ultrasound-guided Prostate Brachytherapy section) allow seeds to be ordered based on the patient anatomy and eliminate the need to estimate seed number and activity from volume estimates and nomograms.24
TREATMENT PLANNING.
All image-based planning, regardless of its purpose, involves two major components: 3D anatomy modeling, using segmented images, and source localization measuring the source positions in the same coordinate system as the anatomic model. Imaging used for anatomic modeling must allow visualization of a volume either by acquiring stacks of planar images by moving the imaging device or patient, as in TRUS or CT, or by sampling the space, as in MRI. Treatment planning systems have been developed for each of these modalities.25, 26, 27 For adaptive intraoperative planning, the selected imaging modality (or combination thereof) must be able to visualize the needles or sources not only relative to relevant anatomy, but also relative to the intended or planned source locations whenever geometric (vs. purely dosimetric) feedback is used.
IMAGE-GUIDED APPLICATOR/SOURCE PLACEMENT.
TRUS-guided permanent prostate implants use TRUS for both image-based planning and image-guided source or needle positioning. TRUS imaging may be coupled with stepper devices to acquire a stack of US images that may be used to create a 3D model for planning purposes. TRUS imaging is also used during the implant to verify that the needles are deployed in a manner consistent with the treatment plan.29
Although TRUS-guided prostate implants may be considered the first IGBT procedure to be widely adopted, brachytherapy has always made use of x-ray imaging to view applicator or needle placement. X-rays are well suited to the visualization of needles or sources in prostate implants during an implant. Although not revealing much about the details of soft tissue anatomy, fluoroscopic images can be used for an independent check of relative placement of needles placed under US guidance or for confirming that sources have been deployed from the needles in the case of permanent implants.29 Because TRUS imaging is able to visualize the
prostate boundary but has limited seed localization accuracy, several groups have proposed combining it with fluoroscopic/radiographic imaging.30
prostate boundary but has limited seed localization accuracy, several groups have proposed combining it with fluoroscopic/radiographic imaging.30
CONFIRMATION/EVALUATION AND POSTIMPLANT DOSE EVALUATION.
Although TRUS imaging can provide the clinician with subjective but near real-time visual feedback that needles are being placed appropriately, it has a number of limitations, including limited seed localization accuracy and an anatomy distorted by the lithotomy position and intrarectal imaging probe. Thus, postprocedure imaging is needed to dosimetrically or geometrically evaluate the deployment of the sources in the patient. Historically, postprocedure imaging focused primarily on the source configuration and, more recently, on computer analysis of CT images, in which case both seed position localization and soft tissue anatomy visualization are needed. However, x-ray CT has limited ability to discern soft tissue structures,31,32 which limits the reliability of summary dose statistics used to ascertain the implant quality and the delivered dose.
Imaging Modalities for Prostate Cancer
In the preplanned TRUS-guided permanent implant paradigm, 3D images derived from stacks or fans33 of TRUS images are used for image-based planning, 2D TRUS images are used intraoperatively for needle guidance, and x-rays or CT scans are used for postimplant evaluation. However, other imaging approaches may be incorporated in each phase, so a review of the imaging options in the pelvis is in order in terms of anatomy visualization, device visualization, and logistical limitations.
FLUOROSCOPY.
Planar x-ray images have played a role in verifying source placement since the birth of brachytherapy. Low-energy x-ray imaging is well suited to differentiate soft tissue from high-density structures such as bone or metal applicators. Although geometric distortions in fluoroscopic images generally make them inappropriate for image-based treatment planning, fluoroscopy is well suited for visualizing needle positions relative to one another and for confirming that seeds have been successfully deployed from the needles. The advent of digital units and image processing tools, such as subtraction of successive images, may offer some advantages. Fluoroscopy units are generally available in a hospital setting and provide a useful modality for an image-guided implant.
TRANSRECTAL ULTRASOUND.
TRUS is the workhorse modality for prostate brachytherapy. TRUS images are used to both plan and guide the implant. Planar US images are well suited for delineating soft tissue interfaces and provide highly interactive near real-time imaging. This allows the clinician to detect dynamic structures, such as a pulsing blood vessel, that might hide in a static grey scale image. With the calibrated overlay of the implant template, TRUS allows verification of needle placement relative to the planned position. US does have a limited depth of view, and TRUS transducers have a fixed size and field of view, which may limit gland sizes that can be visualized. US is not well suited to visualizing metallic structures such as implant needles or sources. Needles are often treated to increase echogenicity; visibility may vary significantly between manufacturers. Although US is generally available in a hospital, TRUS imaging as used in brachytherapy is a specialized modality not generally used in diagnostic imaging.
3D image sets can be formed from digitally captured planar TRUS images, formed either using steppers (giving stacks of transverse images) or computer control of the US device (usually fans of tilted sagittal images).33 It should be noted that using mechanical steppers changes the depth of probe insertion from one image to the next, thereby stabilizing patient anatomy over the course of image acquisition. In contrast, series of sagittal-like images can be acquired without changing the depth of insertion. By uploading 3D TRUS images to the treatment planning system, image-based planning similar to CT-based planning can be achieved. The same concerns about field of view that are associated with planar TRUS images remain, whereas the dynamic nature of the images is lost. However, some planning systems allow simultaneous overlay of the 3D model on a live planar image, allowing the clinician some additional feedback about the accuracy of contouring. TRUS imaging requires good acoustic coupling between the transducer and rectal wall, which generally results in a distortion of the anatomy from the rest geometry. Placement of the probe requires the patient to be in a lithotomy position, which also changes the relative position of anatomy from that seen in other imaging studies with the patient typically supine on an imaging table.
X-RAY COMPUTED TOMOGRAPHY.
Because of the limited soft tissue contrast of x-ray CT imaging, physician-to-physician variability in segmenting prostate, rectum, and other soft tissue structures is not insignificant.32,34,35 Radioactive sources containing high-density materials cause streaking artifacts on axial CT images, which further reduces anatomic structure visualization. CT is well suited for determining the distribution of sources36,37 and is widely used for postimplant evaluation. Although intraoperative CT imaging has been reported,38 often the scanners are located outside the operating room (OR). The growing availability of dedicated outpatient brachytherapy procedure rooms equipped with cone beam CT systems39 may increase use of intraoperative CT imaging. CT-based postimplant evaluation falls in the realm of image-based planning even though the anatomy definition is not optimal.
MAGNETIC RESONANCE IMAGING.
MRI is widely viewed as the imaging modality of choice for the pelvis and should be included in any discussion of pelvic imaging. MRI supports 3D imaging without moving either the device (TRUS) or patient (CT) and provides excellent soft tissue visualization of the prostate and surrounding structures. Although there are pulse sequences that are well suited for soft tissue visualization (T2 weighted), these sequences are not appropriate for source or needle localization. T1-weighted sequences are more appropriate for object localization but do not provide the same level of soft tissue detail. Because of its relatively slow image acquisition process and limited intraoperative availability, MRI is generally used in conjunction with other modalities to improve the tissue contouring and target definition in the post- and preimplant settings.40
MULTIMODALITY IMAGING.
CT, MRI, and TRUS imaging have complementary strengths and drawbacks (Fig. 7.3). Thus, there is considerable effort to develop multimodality image registration algorithms that allow the simultaneous use of these modalities. Figure 7.4 shows TRUS and 1.5-T MRI imaging of a prostate. Endorectal coil MRI is widely perceived to be the modality of choice for pelvic imaging and contains much more information than TRUS or CT. Using registered images is challenging due to the time pressures during the implant procedure and soft tissue deformation that make simple rigid alignment algorithms inaccurate.
PERMANENT SEED PROSTATE BRACHYTHERAPY
Permanent implantation, using low-intensity 125I, 103Pd, or cesium-131 (131Cs) seeds, is the most frequently used brachytherapy modality, especially for low-risk presentations. Although its characteristic ultra low-dose rates provide maximum opportunity for repair of late-responding normal tissues, even the most sophisticated image-guided radiation therapy (IGRT)/adaptive replanning technique cannot guarantee optimal dose delivery to the target due to the unpredictable influence of prostate edema resolution, seed migration, and other forms of organ deformation and tissue motion. Because HDR brachytherapy (HDRBT) delivers the dose over a very short interval, there is greater potential for reproducing the planning anatomy during treatment delivery. Also, anatomy-based dwell weight optimization may be able to compensate for needle insertion spatial errors. HDRBT is discussed in the next subsection.
Conventional Preplanned, Transrectal Ultrasound-guided Prostate Brachytherapy
The current “standard of practice” for permanent seed prostate brachytherapy (Fig. 7.5) consists of three temporally separated imaging procedures.41,42 Several weeks prior to the procedure, preplanning begins with acquisition of a TRUS image set (called “volume study”) to identify the idealized seed arrangement and source strength needed to realize the desired dose distribution (Fig. 7.6A). Radioactive seeds are ordered and prepared in the form of preloaded needles, stranded seed arrays of appropriate length, or single-seed insertion devices. After positioning of the patient and duplication (as judged subjectively) of the TRUS probe alignment relative to the prostate and pubic arch, needles are inserted (Fig. 7.6B) under TRUS guidance. Needle tips cast a strong and relatively localized reflection (Fig. 7.6C), which allows the brachytherapist to control needle positioning relative to the prostate boundary. Most brachytherapists use transverse B-mode imaging, which requires varying the probe insertion depth in the rectum to select the imaged plane. In addition to insertion depth-dependent tissue deformation, seed visualization is poor on transverse TRUS images43 (Fig. 7.7), so that displacements of seed from the needle retraction trajectory are not easily appreciated. An important advance is 3D TRUS imaging using a side-firing rotating transducer, which numerically reconstructs a 3D image set from a fan of tilted parasagittal images,33 eliminating the impact of intrarectal probe repositioning over the axial field of view of the image. Phantom data indicate that 3D rotating fan TRUS imaging improves seed detectability and visualization.44 After completion of the procedure, final implant evaluation is performed using x-ray CT imaging performed the same day or 30 days later. Postprocedure is the most definitive estimate of the dose actually delivered by the procedure.
Although there is no question that the current TRUS-guided transperineal approach is a major advance over direct implantation via suprapubic surgical exposure of the prostate, many sources of error remain. Prostate shrinkage and TRUS probe position differences between volume study and implantation can significantly alter the 3D anatomy. Intraoperative guidance is based on subjective observation; deviations between actual and intended needle insertion paths are quantified neither geometrically nor dosimetrically. Moreover, TRUS can only clearly visualize needle tips rather than deposited seeds, which may deviate 4 to 5 mm from the needle paths. Finally, prostate edema secondary to needle-induced trauma is an important effect. It achieves its maximum just after procedure completion (increasing preimplant CT prostate volume by a factor of 1.53 ± 0.2),45 has a variable half-life (4 to 23 days),46 and can result in day 0 postplanning dose underestimation errors as large as 30%. Finally, as noted earlier, there are large intermodality errors between x-ray CT and TRUS; the former has much larger prostate delineation errors and overestimates prostate volume by 20% to 50%.32
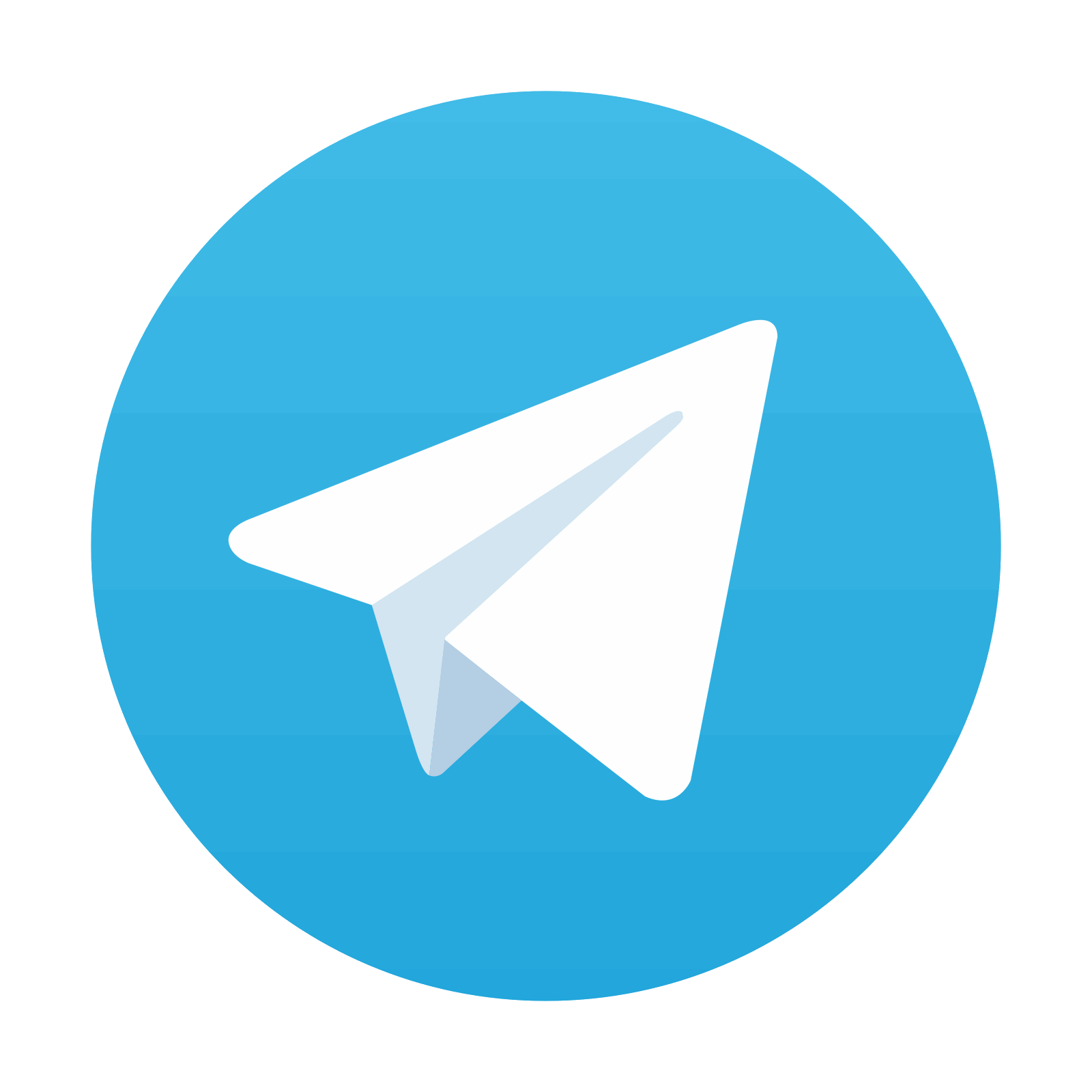
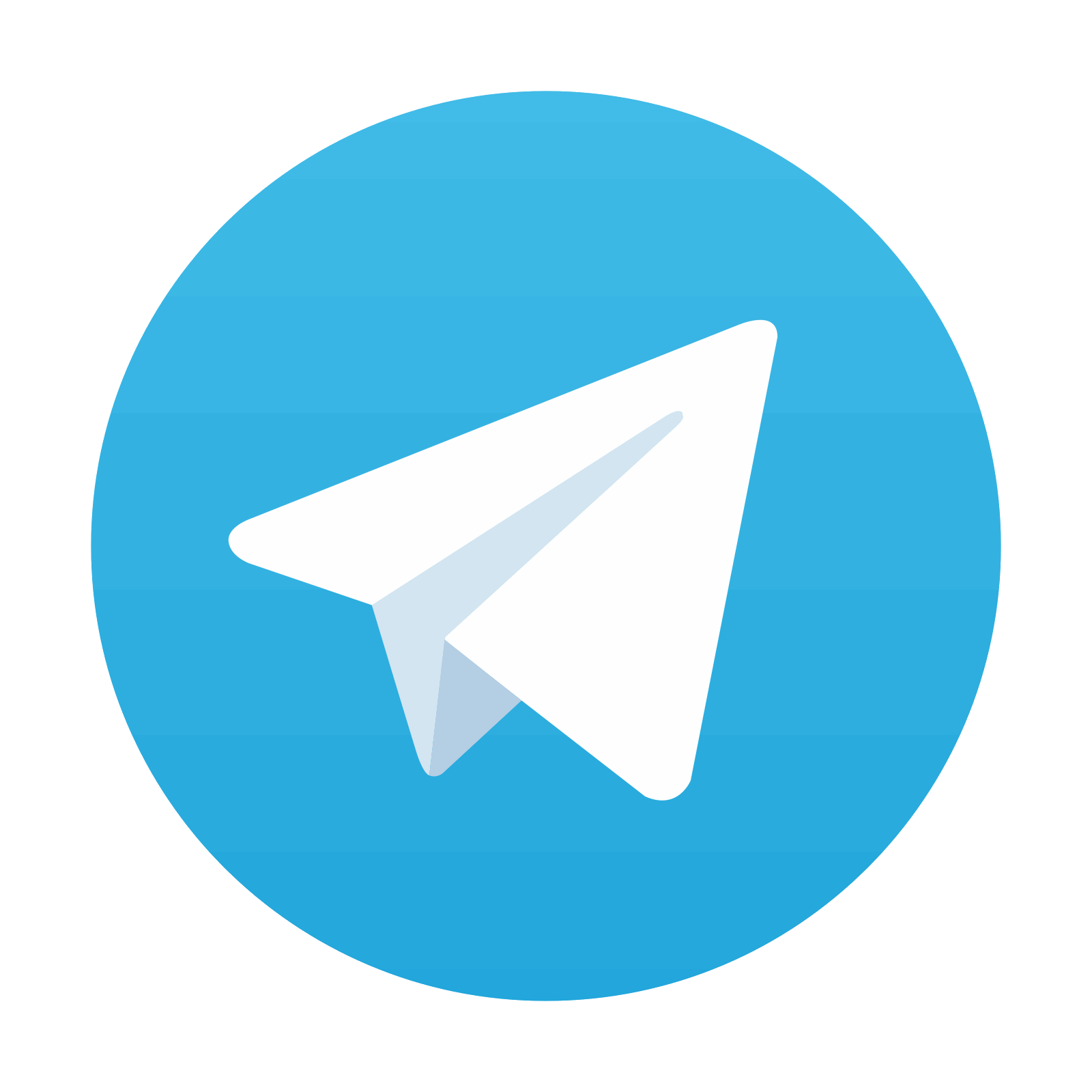
Stay updated, free articles. Join our Telegram channel

Full access? Get Clinical Tree
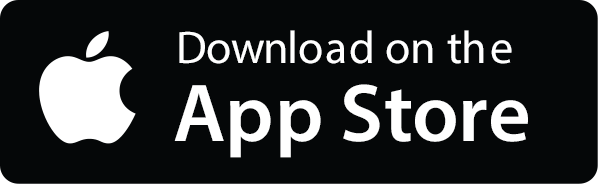
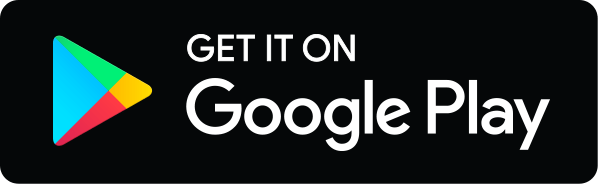
