Focal
Global
Subclinical
Histopathologic thyroid gland changes (not routinely assessed)
Changes in thyroid hormone levels (TSH and/or T4)
Clinical
1. Generally no clinically overt thyroid changes
2. Thyroid nodularity
3. Goiter
1. Clinical symptoms of hypothyroidism (see text)
2. Clinical symptoms of hyperthyroidism (see text)
As early as the 1920s, Groover and colleagues reported that radiation therapy for hyperthyroidism resulted in hypothyroidism after doses 1500–2000 R (on the order of 15–20 Gy), with the incidence of severe hypothyroidism being 1.3 % of 305 patients (Groover et al. 1929). Some early animal studies had suggested that relatively high doses of radiation did not alter the microscopic appearance of the thyroid in the days and months following radiation, Bower and Clark 1923; Eckert et al. 1937; Warren 1943) but did result in a deficiency of physiologic compensatory hypertrophy when a portion of the thyroid has been resected (Eckert et al. 1937). Prior to 1960, hypothyroidism secondary to high doses from radioactive I131 therapy was well characterized, but hypothyroidism from external beam radiation was thought to be uncommon (Felix et al. 1961; Markson and Flatman 1965). In the 1960s, case studies reported that normal thyroid radiation exposure during therapeutic radiation resulted in clinical hypothyroidism months to years after radiation Felix et al. 1961; Markson and Flatman 1965. In a Swedish study of 41 patients treated with radiation for laryngeal and hypopharyngeal cancer, experimentally measured thyroid iodine uptake was diminished as compared to controls; 4 patients had clinical hypothyroidism, 1 of whom had severe hypothyroidism (Einhorn and Wilkholm 1967). In the 1970s, data began to emerge that thyroid malignancies were a late complication after low-dose radiation, particularly in those irradiated as infants and children (Beahrs 1976; Becker et al. 1975; Favus et al. 1056). In a study of over 1,000 patients treated with radiation to the head and neck, 17 % developed nodular disease and 11 % had nonpalpable lesions detected by nuclear imaging; one-third of those patients undergoing surgery for nodular disease had malignancy (Favus et al. 1056).
2 Anatomy and Histology
The thyroid gland is a bilobed, butterfly shaped endocrine gland, with right and left lobes connected by a narrow isthmus (Fig. 1). In some people, there may be a vestigial pyramidal lobe, which is a remnant of the lower end of the thyroglossal duct. The gland is surrounded by a fibrous capsule which attaches to the pre-tracheal fascia. It is situated in the low anterior neck, partially encircling the larynx and trachea anteriorly, extending superiorly from the thyroid cartilage and inferiorly to the tracheal rings (Hancock et al. 1995).
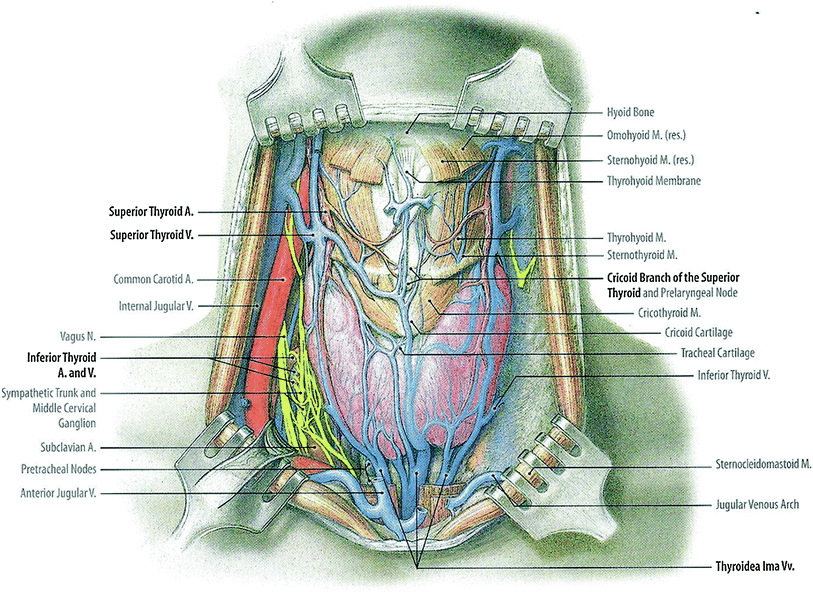
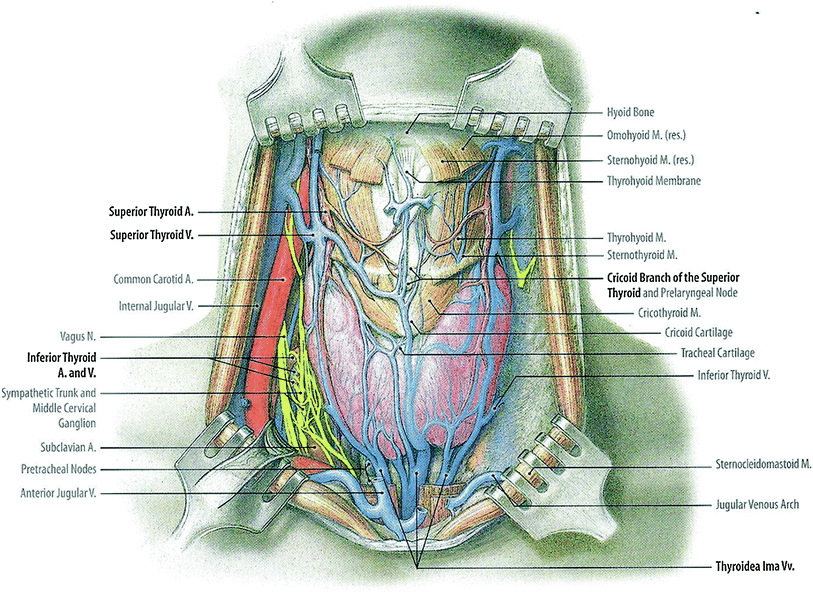
Fig. 1
Gross anatomy of the neck showing the thyroid gland in relation to nerves, vasculature, and muscles (with permission from Tillman 2007)
The thyroid gland consists of follicles, lined with a single layer of epithelial cells that actively transport iodine into the follicle as well as generate and secrete thyroid hormones, T3 and T4 (Fig. 2a, b). The follicles are filled with colloid, rich with the high molecular weight protein thyroglobulin necessary for thyroid hormone synthesis as well as the hormonally inactive monoido- and diiodo-tyrosines (Hancock et al. 1995).
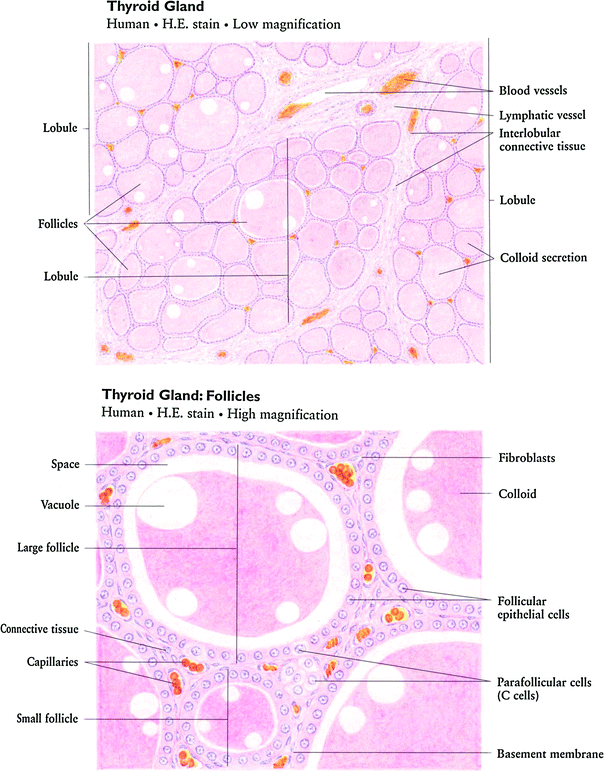
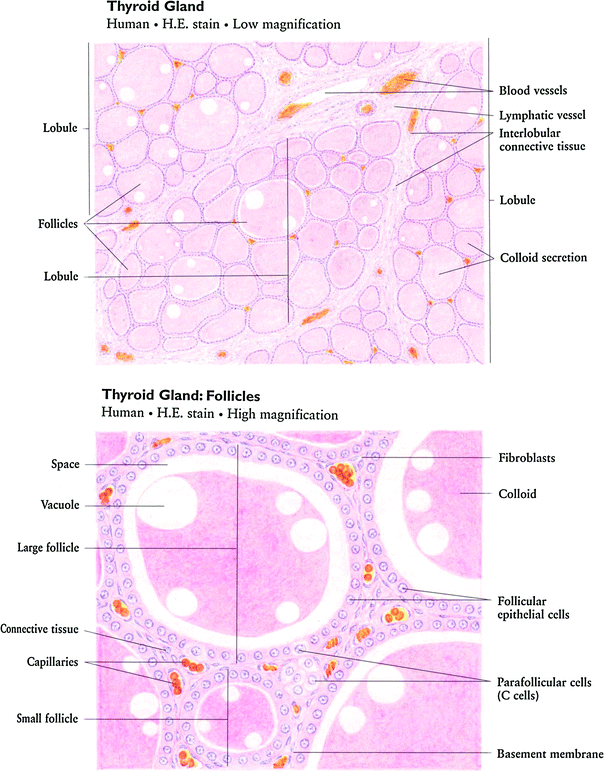
Fig. 2
Histologic appearance of the thyroid gland at low a and high b magnification. At high magnification, the follicular epithelial cells and parafollicular cells are readily apparent (with permission from Zhang 1999)
3 Biology, Physiology, and Pathophysiology
3.1 Physiology and Biology
The follicular epithelial cells synthesize and release triiodothyronine (T3) and thyroxine (T4). Once released into the blood, the active thyroid hormones are bound to and transported via thyroxine-binding globulin, transthyretin, and albumin, with only a small fraction remaining freely unbound (the unbound fraction is hormonally active). T4 is converted to T3 by peripheral organs; T3 is about ten times more active than T4.
The production of T3 and T4 is regulated by thyroid-stimulating hormone (TSH), released by the anterior pituitary gland. TSH production is suppressed by high T4 levels and stimulated when T4 is low. TSH production is modulated by thyrotropin-releasing hormone (TRH), produced by the hypothalamus.
Parafollicular “C-cells,” are sporadically situated between follicles; C-cells secrete calcitonin in response to hypocalcemia, opposing the effects of parathyroid hormone. There does not appear to be any clinically significant consequences from calcitonin deficiency or excess.
3.2 Pathophysiology/Mechanism of Radiation-Induced Injury
Generally, radiation changes in the thyroid result from damage to small vessels (remote branches off the superior and inferior thyroid arteries) and surrounding capsule, and presumably less so from direct damage to the follicular epithelial cells due to their slow turnover (Hancock et al. 1995; Jereczek-Fossa et al. 2004). Rubin and Cassarett postulated that the initial injury was to the endothelium of small vessels, and with additional radiation exposure, the follicular epithelial cells degenerate as a result of further damage to the vasculature (Rubin et al. 1968). Late changes are primarily attributable to vascular damage, while acute and subacute changes may be more affected by repairable damage to the epithelioid cells (Jereczek-Fossa et al. 2004). Acute radiation changes to the thyroid include diminished follicle size with low cuboidal epithelium lining residual follicles, and with higher doses, including therapeutic I131 exposure, follicular necrosis, vasculitis, thrombosis, and hemorrhage followed by vascular sclerosis and lymphocytic infiltration (Hancock et al. 1995). Chronic changes after relatively low-dose exposure include focal and irregular follicular hyperplasia, hyalinization, fibrosis beneath the vascular endothelium, lymphoytic infiltration, thyroiditis, cellular and nuclear atypia, infarction and necrosis (Hancock et al. 1995; Carr and LiVolsi 1989).
In a study of late effects on the thyroid gland in 26 rhesus monkeys undergoing total body irradiation (thus undergoing thyroid as well as hypothalamic-pituitary radiation exposure), increased radiation dose was associated with diminished thyroid gland weight. (Bakker et al. 1999) Half of the thyroid gland specimens (and 6 of 7 in the higher dose group) were noted to have small follicles; one had macrofollicular goiter and papillary hyperplasia. There are scant histopathologic data from humans. In 4 patients from the University of Pennsylvania, undergoing thyroid surgery for palpable nodularity 8–20 years after cervical irradiation, discrete hypercellular adenomatous nodules and hyperplastic nodules (akin to multinodular goiter) were seen in all cases (Carr and LiVolsi 1989). Mild to severe cellular and nuclear atypia was also seen, in the absence of mitoses (Carr and LiVolsi 1989). Thyroid carcinoma can also result from low-dose exposure, though much less commonly. The clinical manifestation of radiation changes includes hypothyroidism, hyperthyroidism, thyroiditis, adenoma formation, and malignancy.
3.3 Cellular Dynamics and Radiation Response
The extent to which there is thyroid organ recovery and/or regeneration is not well documented in the literature. Likewise, the kinetics of the pathophysiologic changes resulting in late thyroid toxicity are not well understood. The latency period between radiation exposure and the clinical manifestation of late thyroid toxicity varies widely. Radiation-induced occult hypothyroidism progresses to clinical hypothyroidism in about a quarter of patients, (Constine et al. 1984) suggesting progression and/or evolution of radiation-induced damage (Hancock et al. 1995). However, rarely hormone levels spontaneously normalize, suggesting a mechanism for repair in some patients (Hancock et al. 1995; Constine et al. 1984). Late transient “silent” thyrotoxicosis has also been observed, albeit uncommonly. This is generally followed by overt hypothyroidism, suggesting that the recovery from thyrotoxicosis leaves a hypo-functioning gland.
4 Clinical Syndromes
To date, there are no compelling data to suggest that susceptibility to radiation-induced thyroid abnormalities has a component of genetic predisposition. The focal and global effects of radiation on the thyroid are briefly summarized in Table 1.
4.1 Hypothyroidism
Hypothyroidism may manifest with classic symptoms, including weight gain, cold intolerance, dry skin, brittle hair, constipation, menstrual irregularities, muscle cramping, and slower mentation; classic signs include periorbital and peripheral edema, hypotension, bradycardia, pericardial effusions, pleural effusions, and prolonged relaxation of deep tendon reflexes. Hypothyroidism is a common condition of the elderly, and thus the contribution of radiation in the development of hypothyroidism in elderly patients may not be readily determined. Hypothyroidism may be occult, detected only by low thyroxine levels and/or elevated TSH (Boomsma et al. 2011). This is often referred to as subclinical or covert hyothyroidism, as opposed to clinical or overt hypothyroidism, in which the hypothyroidism is associated with clinical symptomatology. Compensated hypothyroidism occurs when the pituitary gland releases high levels of TSH, and thus overworks the thyroid gland to maintain adequate levels of circulating thyroid hormones. With uncompensated hypothyroidism, T4 remains low despite elevated TSH. Radiation-induced occult hypothyroidism is likely to progress to clinical hyothyroidism, (Hancock et al. 1995) though TSH levels can spontaneously normalize over long time intervals (Hancock et al. 1995; Constine et al. 1984).
The reported incidence of hypothyroidism after thyroid radiation ranges from >15–50 % (Boomsma et al. 2011). Most studies show the incidence of hypothyroidism progressively rising in the first 3–5 years after radiation, with most patients manifesting clinical signs or symptoms 2–4 years after radiotherapy (Bhandare et al. 2007; Tell et al. 2004; Kim et al. 1980). Some studies demonstrate additional cases of hypothyroidism beyond 5 years, (Garcia-Serra et al. 2005; Mercado et al. 2001; Vrabec and Heffron 1981; Tamura et al. 1981; Hancock et al. 1991) and others show minimal to no appreciable additional risk beyond 5 years (Bhandare et al. 2007; Tell et al. 2004; Kumpulainen et al. 2000).
In a recent study from the University of Florida, in which 312 patients with nasopharynx, nasal cavity, and nasal sinus cancers, the thyroid dose associated with primary hypothyroidism ranged from 36.5 to 65.6 Gy (median 45 Gy); increased radiation dose was significantly correlated with a greater risk of primary hypothyroidism (Bhandare et al. 2007). The median clinical latency was 3.1 years; latency was independent of dose. Ten-year rates of clinical and occult hypothyroidism were 33 and 29 % (of those without clinical hypothyroidism) respectively. These values are similar to those reported by other groups with a large patient population and long follow-up for both head and neck cancer (Tell et al. 2004; Vrabec and Heffron 1981; Kumpulainen et al. 2000) and Hodgkin’s disease (Tamura et al. 1981; Hancock et al. 1991). In an earlier study from the University of Florida, 50 Gy delivered as elective nodal treatment in patients with oropharyngeal cancer resulted in hypothyroidism in 30–50 % of patients at 5 years (Norris et al. 2006). In a Swedish study in 391 patients with head and neck cancer, the 10-year rate of ‘overt’ hypothyroidism (elevated TSH and decreased T4, or requirement of thyroid replacement) was 27 % with a latency of 0.3–8.1 years (median 1.8) (Tell et al. 2004). In a study from Cleveland Clinic, the 8-year actuarial incidence of hypothyroidism was reported to be 67 %, higher than that reported in other series (Mercado et al. 2001). In the Childhood Cancer Survivor Study (CCSS) cooperative group trial, in which 1791 patients with childhood Hodgkin’s disease were studied, hypothyroidism developed 0–27 years (mean 7 years) after diagnosis (Sklar et al. 2000). Significantly increased risk was associated with doses ≥ 45 Gy versus 35–45 Gy vs. <35 Gy versus no radiation.
4.2 Hyperthyroidism
Hyperthyroidism can manifest with classic symptoms including palpitations, heat intolerance, anxiety, fatigue and insomnia, diarrhea, menstrual irregularities, weight loss, weakness. Clinical signs include goiter, tachycardia, tremor, warm moist skin, thinner skin, fine hair and alopecia, infiltrative ophthalmopathy. Grave’s disease is a autoimmune form of hyperthyroidism resulting from the production of antibodies which bind to and chronically stimulate the receptor for thyroid-stimulating hormone (which are expressed in on the follicular cells of the thyroid gland) causing increased production of the T3 and T4 hormones.
Radiation-induced hyperthyroidism is a well-documented complication, albeit much less common than hypothyroidism. In Stanford’s series of 1,787 patients treated with radiation for Hodgkin’s disease, hyperthyroidism was observed in 30 patients (1.7 %) 3 weeks to 18 years (mean 5.3 years) posttreatment (Hancock et al. 1991). Ten of these 30 patients were previously diagnosed and treated for hypothyroidism. An additional 4 patients developed infiltrative ophthalmopathy consistent with Grave’s disease, despite being clinically hypothyroid (n = 3) and euthyroid (n = 1). In a Hungarian study, 2 of 151 patients (1.3 %) treated with radiation for Hodgkin’s disease developed hyperthyroidism, (Illes et al. 2003) and in a French study of 478 patients treated with total body irradiation, one patient developed hyperthyroidism (Thomas et al. 2001). In the CCSS cooperative group trial, including 1,791 patients with childhood Hodgkin’s disease, hyperthyroidism developed in 82 patients (4.5 %) 0–22 years (mean 8 years) after diagnosis (Sklar et al. 2000). Significantly increased risk was associated with doses ≥ 35 Gy.
4.3 Thyroiditis
Thyroiditis is a non-specific condition referring to inflammation of the thyroid gland; thyroiditis can result in hyperthyroidism and/or hypothyroidism. Hashimoto’s thyroiditis is an auto-immune related condition, generally resulting in hypothyroidism. Thyroiditis is an uncommon complication from therapeutic external beam radiation as opposed to the relatively high incidence of thyroiditis resulting from radioactive iodine therapy.
In Stanford’s series of 1,787 patients, 4 patients developed Hashimoto’s thyroiditis and 6 patients developed transient ‘silent’ thyrotoxicosis 10–24 months (except 1 patient at 15 years) after radiation; ‘silent’ thyroiditis was described as elevated thyroid hormone levels, low TSH, and low radio-iodine uptake (Hancock et al. 1991). In addition to Stanford’s series, there have been a few case reports of ‘silent’ thyrotoxicosis several months to years after radiation, generally transient in nature, and usually followed by overt clinical hypothyroidism (Blitzer et al. 1985; Fachnie and Rao 1980; Petersen et al. 1989). In a French study of 478 patients treated with total body irradiation, 6 patients developed thyroiditis, though the clinical presentation and method of diagnosis are not described (Thomas et al. 2001). In one case report, ‘silent’ thyroiditis was noted 2 weeks after radiation (Bryer-Ash et al. 2001).
In the above-mentioned Hungarian study of 151 patients treated with radiation for Hodgkin’s disease, 28 patients were found to have serum anti-thyroglobulin antibodies; 38 patients (mostly those undergoing neck radiation) developed subclinical (n = 26) and clinical (n = 12) hypothyroidism (Illes et al. 2003). Of those with detectable serum anti-thyroglobulin antibodies, 53.6 % had thyroid dysfunction versus 20.3 %, mostly occult hypothyroidism, in those without detectable serum anti-thyroglobulin antibodies. Hypothyroidism was significantly more common in patients undergoing neck radiation (>30 %) versus those receiving chemotherapy alone (5 % among 40 patients); the risk of thyroiditis was roughly twofold higher in patients treated with radiation (26 vs. 13 %), although this difference was not significant. The authors postulate that hypothyroidism may in part result from thyroiditis, which in turn might be result of changes in immune reactivity in patients with Hodgkin’s disease (Illes et al. 2003).
4.4 Nodules: Benign and Malignant
Radiation has also been reported to result in benign adenoma formation as well as thyroid cancer. Generally, radiation-induced thyroid malignancies are localized to the thyroid, and readily curable (Schneider et al. 1986). Historically, low-dose radiation (as low as 0.1 Gy) for benign disease (such as tinea capitis, thymic enlargement, lymphoid hyperplasia, lice, acne) has been a well-established risk factor for thyroid nodules and malignancy, with increasing dose (Favus et al. 1056; Adams et al. 2010; Ron et al. 1995) and decreasing age at exposure increasing the risk (Ron et al. 1995). In the 1970–1980s, case-reports were published suggesting that the higher therapeutic radiation doses used to treat malignancy could also induce thyroid nodules and thyroid cancer (McDougall et al. 1980). The vast majority of radiation-induced solid tumors occur within the radiation field (Bhatia et al. 2003; Garwicz et al. 2000).
In MD Anderson’s series of 145 child and adult patients treated with radiation for Hodgkin’s disease, 7 patients developed palpable thyroid tumors, of which 2 were malignant (Liao et al. 2001). In Roswell Park’s series of 126 adult patients treated for Hodgkin’s disease and non-Hodgkin’s lymphoma, 12 patients (11 of whom were women) developed thyroid nodules 1–6 years after treatment, 5 of whom did not undergo prior neck radiation (Tamura et al. 1981). No patient underwent thyroidectomy and 2 underwent biopsy, which revealed adenoma in one patient and thyroiditis in another.
In Stanford’s series of 1,787 child and adult patients, palpable thyroid nodules were appreciated in 44 patients (2.5 %) from 1.5 to 25 years after radiation (Hancock et al. 1991). Eighteen patients had clinically benign disease, including nodules that concentrate radioiodine (n = 12), cysts (n = 4, based on ultrasound and aspiration), and multinodular goiter (n = 2). Twenty-six had pathologically benign disease (which was clinically worrisome for malignancy given the lowered radioiodine uptake), including adenoma (n = 10), adenomatous nodule (n = 6), and multinodular goiter (n = 4). Six patients had thyroid cancer 9–19 years after radiation therapy, with a relative risk of 15.6 (p < 0.00001) and absolute risk of 33.9 per 100,000 person years. All patients with cancer remained without evidence of disease recurrence at last follow-up.
In the CCSS cooperative group trial, including 1,791 patients with childhood Hodgkin’s disease, thyroid nodules developed in 146 patients (8.2 %) 0–22 years (mean 14 years) after diagnosis, with a relative risk of 27 (Sklar et al. 2000). Significantly increased risk was associated with doses ≥ 25 Gy. Eleven of the 146 patients had thyroid cancer in addition to 9 patient with thyroid cancer not associated with thyroid nodularity.
In a multi-institutional retrospective study of 930 childhood Hodgkin’s disease survivors, 1.5 % developed thyroid cancer, at 8.5–23 (median 14.4) years after Hodgkin’s disease diagnosis, with ~4 times as many females developing thyroid cancer as compared to males (Constine et al. 2008).
5 Radiation Tolerance and Radiation-Induced Injury Risk Factors
5.1 Hypothyroidism
The risk of radiation-induced hypothyroidism is dependent on many factors, including the method of detection (i.e., clinical symptoms versus biochemical hypothyroidism), patient related factors, cancer related factors, treatment related factors, and adequacy and length of follow-up; as a result, the reported risk of hypothyroidism varies widely (Jereczek-Fossa et al. 2004). For example, in one study from the Joint Center of Radiation Therapy, the calculated actuarial incidence of hypothyroidism at 1 year was 27 %, but when the calculation included only patients with ≥5 months follow-up, the 1-year incidence was 49 %, which highlights the importance of adequate follow-up when reporting late toxicity (Colevas et al. 2001).
Factors that have been reported to increase the risk of radiation-induced hypothyroidism include older age, (Colevas et al. 2001; Tell et al. 1997) younger age, (Hancock et al. 1991; Sklar et al. 2000; Glatstein et al. 1971) female gender, (Tell et al. 2004; Alterio et al. 2007; Vrabec and Heffron 1981; Hancock et al. 1991; Wu et al. 2010; Posner et al. 1984) caucasian race, (Mercado et al. 2001; Metzger et al. 2006a) smaller thyroid volume, (Alterio et al. 2007; Diaz et al. 2010) baseline TSH, (Tell et al. 2004) concurrent or adjuvant chemotherapy, (Hancock et al. 1991; Posner et al. 1984) (with one study suggesting chemotherapy lowers the risk of hypothyroidism (Tamura et al. 1981)), resection/hemithyroidectomy, (Tell et al. 2004; Garcia-Serra et al. 2005; Turner et al. 1995; Vrabec and Heffron 1981; Liening et al. 1990; Kumpulainen et al. 2000; Tell et al. 1997; Posner et al. 1984; Leon et al. 2002; Ozawa et al. 2007; Smolarz et al. 2000; Shafer et al. 1975) and comorbid diabetes (Bhandare et al. 2007). Radiation dose is also significant in several studies, as summarized in Table 2 (Bhandare et al. 2007; Constine et al. 1984; Hancock et al. 1991; Sklar et al. 2000; Glatstein et al. 1971; Weissler and Berry 1991; Kaplan et al. 1983; Kuten et al. 1996). A cohort of childhood Hodgkin lymphoma survivors treated between 1970 and 1986 were evaluated for thyroid disease by use of a self-report questionnaire in the CCSS. Among 1,791 survivors, 34 % reported that they had been diagnosed with at least one thyroid abnormality. For hypothyroidism, there was a clear dose response, with a 20-year risk of 20 % for those who received <35 Gy, 30 % for those who received 35–44.9 Gy, and 50 % for those who received >45 Gy to the thyroid gland. The relative risk for hypothyroidism was 17.1. Elapsed time since diagnosis was a risk factor, where the risk increased in the first 3–5 years after diagnosis (Sklar et al. 2000). The cumulative incidence of hypothyroidism among survivors 15 years following leukemia diagnosis has also been evaluated in the CCSS and was 1.6 %. In multivariate analysis, survivors who received ≥ 20 Gy cranial radiotherapy plus any spinal radiotherapy had the highest risk of subsequent hypothyroidism (hazard ratio 8.3) compared with those treated with chemotherapy alone. In radiation dosimetry analysis, pituitary doses >20 Gy combined with thyroid doses ≥10 Gy were associated with hypothyroidism (Chow et al. 2009). Figure 3 shows the dose dependence of hypothyroidism risk from one study of survivors of childhood cancer. In the German “Registry for the Evaluation of Side Effects After Radiotherapy in Childhood and Adolescence” (RiSK) study of 404 patients (with various diagnoses), higher doses to the thyroid were associated with increased risks of TSH elevation (Bolling et al. 2010).
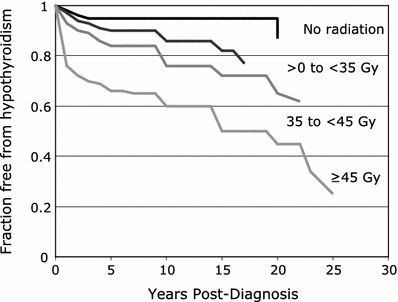
Table 2
Radiation dose to the thyroid gland and the risk of hypothyroidism
Institution | Patient population | # | Risk of hypothyroidism |
---|---|---|---|
Stanford U (Constine et al. 1984) | Children with Hodgkin’s lymphoma | 119 | Thyroid disorders (mostly hypothyroidism): 78 % for >26 Gy vs. 17 % for ≤26 Gy |
Stanford U (Hancock et al. 1991) | Adults and children with Hodgkin’s lymphoma | 1,787 | Clinical hypothyroidism: 20 % for ≥30 Gy vs. 5 % for 7.5–30 Gy at 20 years, p = 0.001 |
Subclinical or clinical hypothyroidism: 44 % for ≥30 Gy vs. 27 % for 7.5–30 Gy at 20 years, p = 0.008 | |||
Childhood cancer Survivor study (Sklar et al. 2000) | Children with Hodgkin’s disease | 1,791 | Patient reports “underactive thyroid” 50 % for ≥45 Gy vs. 30 % for 35–45 Gy vs. ~20 % for <35 Gy at 15 years |
Joint center for Radiation therapy (Kaplan et al. 1983) | Childhood cancer | 95 | Elevated TSH: 68 % for ≥30 Gy vs. 15 % for <30 Gy p = 0.007 |
RiSK Study(Bolling et al. 2010) | Childhood cancer | 404 | Elevated TSH, versus prophylactic cranial RT 15–25 Gy to thyroid gland, RR 3.1 (p = 0.002)>25 Gy to thyroid gland, RR 3.8 (p = 0.009)Cranio-spinal radiation, RR 5.7 (p < 0.001) |
U. Florida (Bhandare et al. 2007) | Adults with head and neck cancer | 197 | Clinical hypothyroidism: 39 % for ≥45 Gy vs. 21 % for <45 Gy at 10 years, p = 0.04 |
Federico II U. (Cella et al. 2011) | Teenagers and adults with Hodgkin’s lymphoma | 53 | Subclinical or clinical hypothyroidism: 11.5 % for thyroid V30 ≤ 62.5 % 70.8 % for thyroid V30 > 62.5 %, p < 0.0001 |
Vanderbilt U. (Diaz et al. 2010) | adults with head and neck cancer | 63 | Not significant: area under the DVH curve, V10, V20, V30, V40, V50, V60, V70, or median, minimum, or maximum thyroid dose |
U. Milan (Alterio et al. 2007) | adults with head and neck cancer | 57 | V10, V30, V50 not significant for clinical or subclinical hypothyroidism |
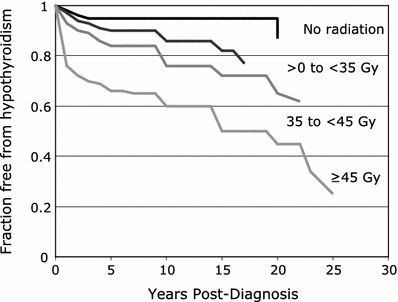
Fig. 3
Dose dependence of hypothyroidism risk from a study of 1,791 survivors of childhood cancer. Data and figure was derived from reference (Sklar et al. 2000), in which actuarial rates of hypothyroidism were calculated among 377 patients who received no radiotherapy, and 1,210 patients for whom sufficient data were available to estimate the dose of radiation to the thyroid gland (median 35 Gy and range 0.004–55 Gy)
Some studies suggest that age, (Bhandare et al. 2007; Constine et al. 1984; Mercado et al. 2001; Tamura et al. 1981; Illes et al. 2003; Kumpulainen et al. 2000; Tami et al. 1992; Kaplan et al. 1983; Smith et al. 1981; Boomsma et al. 2012) gender, (Bhandare et al. 2007; Constine et al. 1984; Colevas et al. 2001; Mercado et al. 2001; Tamura et al. 1981; Liening et al. 1990; Tell et al. 1997; Glatstein et al. 1971; Diaz et al. 2010; Tami et al. 1992; Weissler and Berry 1991; Kaplan et al. 1983; Smith et al. 1981; Bethge et al. 2000) race, (Bhandare et al. 2007; Garcia-Serra et al. 2005) prior neck dissection, (Smith et al. 1981; Garcia-Serra et al. 2005; Colevas et al. 2001; Norris et al. 2006; Sinard et al. 2000; Weissler and Berry 1991; Boomsma et al. 2012) concurrent or adjuvant chemotherapy administration, (Bhandare et al. 2007; Constine et al. 1984; Mercado et al. 2001; Sklar et al. 2000
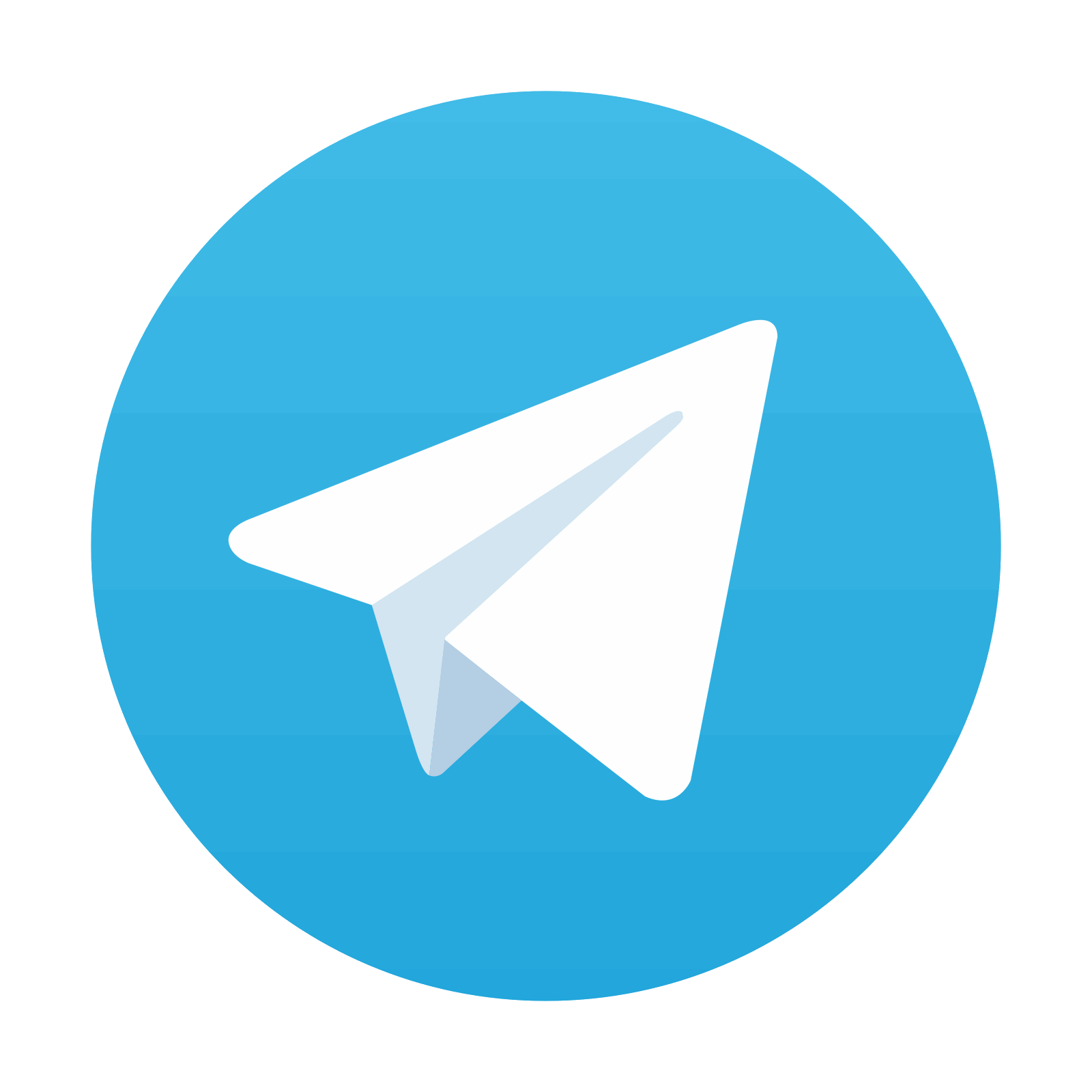
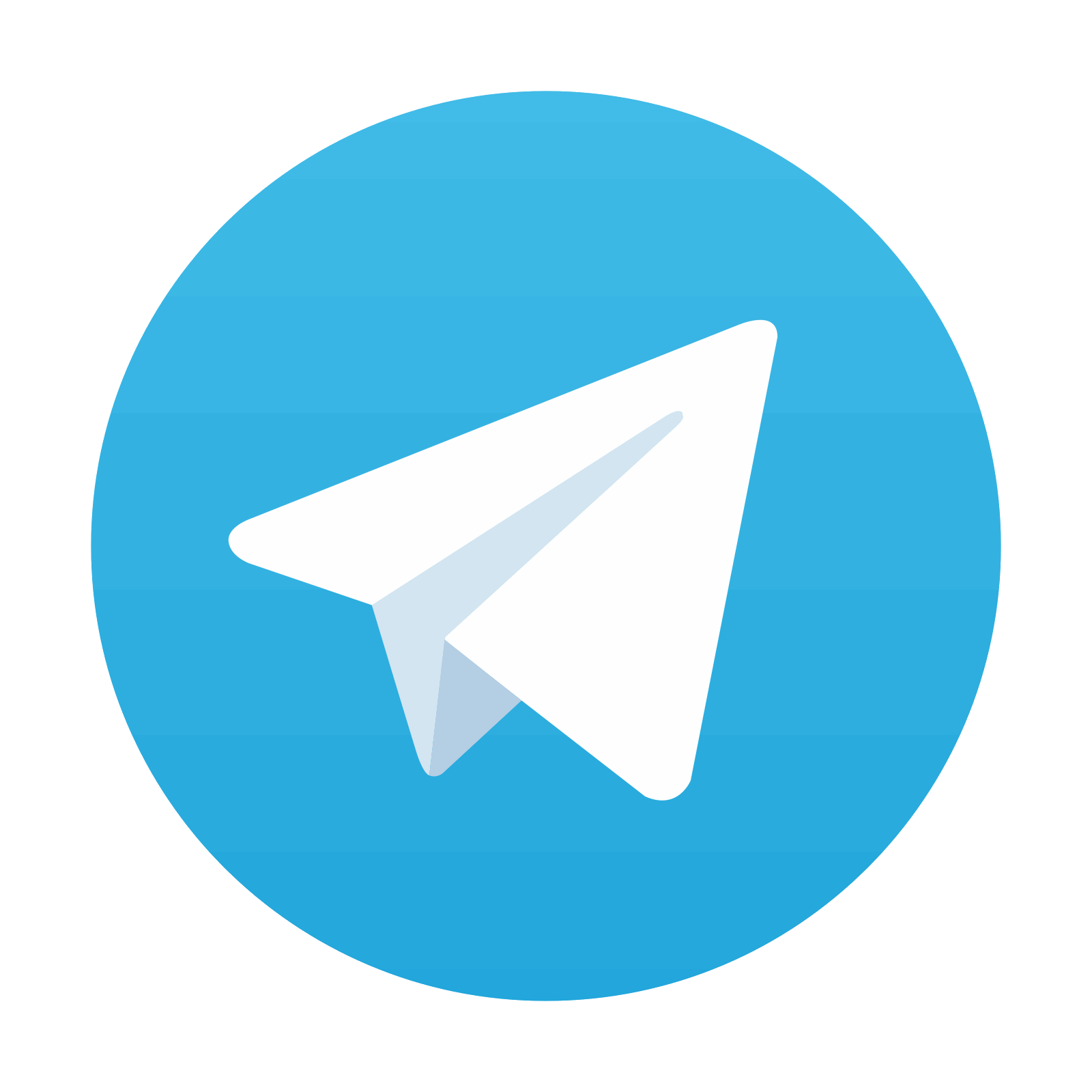
Stay updated, free articles. Join our Telegram channel

Full access? Get Clinical Tree
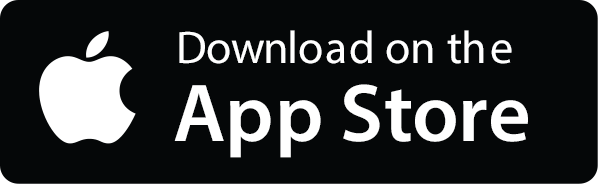
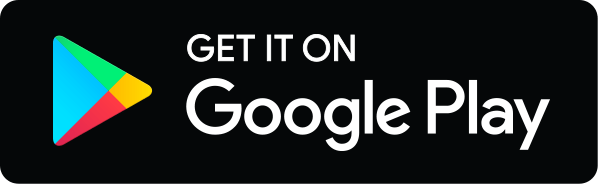