Organ at risk
JCOG 0403 (4 fractions)
RTOG 0618 (3 fractions)
JROSG10-1 (8 fractions)
RTOG 0813 (5 fractions)
Spinal cord
≤25 Gy
≤18 Gy
≤35.5 Gy
≤30 Gy
(22.5 Gy < 0.25 cc)
(13.5 Gy < 0.5 cc)
Esophagus
40 Gy ≤ 1 cc
≤27 Gy
40 Gy < 5 cc
≤105 % of PTV prescription
35 Gy ≤ 10 cc
(27.5 Gy < 5 cc)
Pulmonary artery
40 Gy ≤ 1 cc
–
54.5 Gy < 1 cc
<105 % of PTV prescription
47.5 Gy < 10 cc
35 Gy ≤ 10 cc
(47 Gy < 10 cc)
Aorta
–
–
58 Gy < 10 cc
<105 % of PTV prescription
(47 Gy < 10 cc)
SVC/Pulmonary vein
–
–
48 Gy < 1 cc
<105 % of PTV prescription
(47 Gy < 10 cc)
Heart/Pericardium
–
≤30 Gy
40 Gy < 15 cc
<105 % of PTV prescription
(32 Gy < 15 cc)
Trachea/Bronchus
40 Gy ≤ 10 cc
≤30 Gy
54.5 Gy < 10 cc
<105 % of PTV prescription
(18 Gy < 4 cc)
Brachial plexus
–
≤24 Gy
≤40 Gy
≤32 Gy
(30 Gy < 3 cc)
Skin
–
≤24 Gy
≤40 Gy
≤32 Gy
(30 Gy < 10 cc)
Stomach/Intestine
36 Gy < 10 cc
–
–
–
30 Gy < 100 cc
Lung (right & left)
40 Gy < 100 cc
V20 ≤ 10 %
V20 < 20 %
V20 < 10 %
V20 ≤ 20 %
(12.5 Gy < 1,500 cc)
V15 ≤ 25 %
(13.5 Gy < 1,000 cc)
MLD ≤ 18 Gy
Other organs
48 Gy ≤ 1 cc
–
65.5 Gy < 1 cc
–
40 Gy ≤ 10 cc
54.5 Gy < 10 cc
13.1.1 Radiation Pneumonitis
Radiation pneumonitis (acute and late) is most common toxicity after SBRT in the treatment for lung cancers. Most of radiation pneumonitis observed after SBRT is grade 1–2, and grade 3 or greater radiation pneumonitis is reported less than 10 % [2, 3]. Grade 5 pulmonary complications including radiation pneumonitis has been reported 0.6 % in multi-institutional survey in Japan [4]. Most cases of Grade 5 pneumonitis is reported to be accompanied with interstitial lung disease [5–7].
Generally, radiation pneumonitis develops from 2 to 6 months after SBRT [8]. Symptomatic pneumonitis tends to appear earlier than asymptomatic pneumonitis [9]. Acute radiological changes are typically categorized to five patterns as follows; (1) diffuse consolidation, (2) patchy consolidation, (3) diffuse ground glass opacities, (4) patchy ground glass opacities, and (5) no evidence of increasing density [10.11]. Among them, diffuse consolidation is most common and the frequency is approximately 20–30 % [12]. These acute radiological changes typically correspond to the lung volume exposed to 20 Gy or over doses of radiation (V20). Radiation pneumonitis gradually proceeds to the process of fibrosis as late radiological changes. Radiation fibrosis is categorized into modified conventional pattern, mass-like pattern, and scar-like pattern, and no evidence of increasing density [10–12]. The most predominant pattern is modified conventional pattern [10–12]. Figures 13.1 and 13.2 show the cases the radiation fibrosis of modified conventional pattern and scar-like pattern, respectively. Among them, radiation fibrosis showing mass-like pattern, also called mass-like consolidation (fibrosis), sometimes is difficult to be distinguished from local recurrence. These radiological changes are usually fixed within 24 months after SBRT [10, 13], and tend to be mild in the patients with pulmonary emphysema [14].
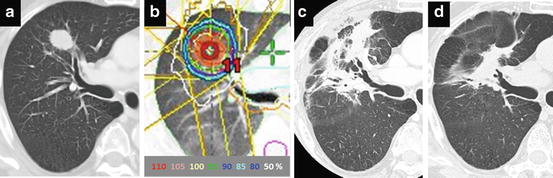
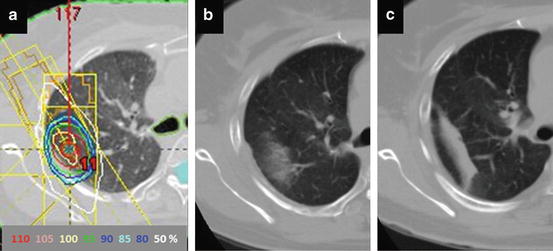
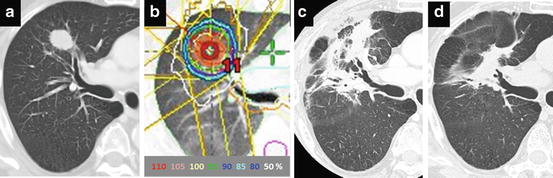
Fig. 13.1
Post-SBRT radiation fibrosis considered modified conventional pattern. (a) CT image before SBRT, (b) Isodose distribution prescribed with 48 Gy in 4 fractions at isocenter, (c) CT image at 5 months after SBRT, (d) CT image at 24 months after SBRT
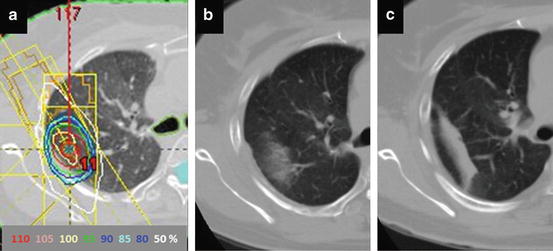
Fig. 13.2
Post-SBRT radiation fibrosis considered scar-like pattern. (a) Isodose distribution prescribed with 48 Gy in 4 fractions at isocenter, (b) CT image at 3 months after SBRT, (c) CT image at 24 months after SBRT
Dosimetric factors including V5, V20, and MLD have been well documented in conventional radiotherapy [15, 16]. Also in SBRT, dose-volume metrics such as V20, V25 and MLD are considered to be correlates with symptomatic radiation pneumonitis after SBRT [17–20]. Barriger et al. documents that V20 and MLD are significant factors correlated with grade 2 or greater radiation pneumonitis after SBRT with 60 Gy in 3 fractions. In their results, grade 2–4 pneumonitis developed in 16.4 % and 17.6 % of patients with V20 > 4 % and those with MLD > 4 Gy, respectively. In contrast, the incidence of grade 2 or greater pneumonitis was reported 4 % in the patients with V20 < 4 % and MLD < 4 Gy [18]. Matsuo et al. report that PTV and V25 is significant dose-volume metric associated with grade 2 or greater pneumonitis in their series of SBRT with 48 Gy in 4 fractions [19]. Takeda et al. report that grade 2 pneumonitis is well correlated with dose-volume metrics including V5-V30 and MLD, but grade 3 or higher pneumonitis tend to be associated with variables of the host rather than those of dose-volume metrics [20]. In addition to these dose-volume metrics, patient factors including gender, age, pulmonary function (FEV1.0) and smoking history are suggested to be associated with the risk of radiation pneumonitis.
Bronchiolitis obliterans organizing pneumonia (BOOP) is well known as a particular from of pneumonitis developing beyond the radiation field, which is observed in 2–3 % of the breast cancer patients who received postoperative irradiation. Radiation pneumonitis similar to BOOP is reported to be found in 4.8 % of patients with stage I NSCLC and metastatic lung tumor [21]. Also, radiation recall pneumonitis induced by chemotherapy is reported [22]. The risk of recall pneumonitis may be considered in the setting of adjuvant chemotherapy after SBRT.
13.1.2 Pulmonary Function
In the treatment for small lung tumors, typically in stage I NSCLC, SBRT does not significantly affect pulmonary function. Although the clinical evidence regarding pulmonary function changes is still limited, most of previous retrospective studies have shown that impairment of pulmonary function is generally little and transient. Ohashi et al. report that there is no significant change in total lung capacity (TLC), vital capacity (VC) and forced expiratory volume in 1 s (FEV1.0) 1 year after SBRT. Conversely, diffusing capacity of lung for carbon monoxide (DLCO) is found to be improved in patients who had been heavy smokers before SBRT [23]. Stephans et al. also report that pulmonary function is not substantially altered by SBRT, although individual patients may exhibit increased or decreased FEV1.0 and DLCO values after treatment [24]. In contrast, there are several studies showing significant decrease of FEV1.0 or DLCO. Henderson et al. show that DLCO decrease by 1.11 ml/min/mmHg while FEV1.0 does not change over time [25]. Mclnerney et al. show that FEV1.0 and DLCO were reduced 6–7 % and 16–17 %, respectively, and that DLCO decrease correlated with MLD and V10–20 [26]. However, it is generally difficult to show the correlation of dose-volume parameters and impairment of pulmonary function because many of patients treated with SBRT have pre-existing chronic obstructive disease which affects worsening of pulmonary function rather than radiation toxicity.
13.1.3 Skin and Chest Wall Toxicity
Toxicities of the skin and chest wall are common in the treatment for periphery located lung tumors. The risk factors predicting grade 2 or greater skin toxicity are shown a limited number of beams, distance from the tumor to the chest wall, and a maximum skin dose of exceed 50 % of the prescribed dose [27]. In Radiation Therapy Oncology Group (RTOG) 0618 trial using 3 fractions for stage I NSCLC, dose constraint (≤24 Gy) is determined for the skin as one of the organ-at-risk. On the other hand, in the Japan Clinical Oncology Group (JCOG) 0403 trial using 4 fractions, the skin is not included as an organ-at-risk because the risk of severe skin toxicity is very low in total dose of 48 Gy.
Toxicities of the chest wall include chest wall pain, fibrosis of soft tissue, and rib fracture [28]. Rib fracture can be asymptomatic and is found incidentally on follow-up CT examination. Approximately 50 % of patients with rib fracture are reported asymptomatic [29]. Therefore, the incidence of rib fracture might be underestimated in early retrospective series. Including asymptomatic rib fracture, the incidence is reported 30–50 % in the patients after SBRT in recent literatures [29, 30]. Figure 13.3 shows a case with asymptomatic rib fracture after SBRT. The chest wall toxicity is more likely to be observed in patients with periphery located lung tumors close to the chest wall (≤1.8 or 2.0 cm) [29, 31]. Dunlap et al. report that the chest wall volume receiving more than 30 Gy predicts risk of severe pain and/or rib fracture [30]. In studies focusing on the rib dose, high dose volume including maximum dose and D2cc is well correlated with the risk of rib fracture [29, 32].
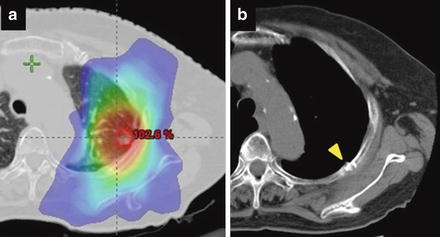
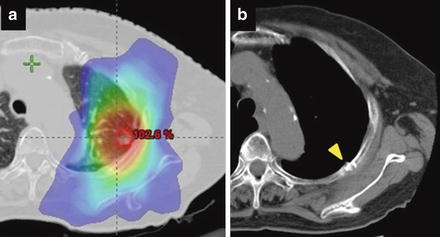
Fig. 13.3
Asymptomatic rib fracture developed at 24 months after SBRT. (a) Isodose distribution prescribed with 48 Gy in 4 fractions at isocenter, (b) CT image 18 months after SBRT. A yellow arrow shows the SBRT-induced rib fracture with a linear sclerotic change and discontinuity of the cortex
Regarding chest wall pain, Welsh et al. report that 25 % of patients treated with SBRT experienced chest wall pain, and many of them had no rib fractures. They also found that body mass index (BMI) and diabetes were strong predictors for the development of chest pain [33]. By contrast, the incidence of grade 2 or higher chest wall pain is reported very low in Japanese SBRT series [29, 34]. Difference in BMI or diabetes rate between nationalities might be a cause of the difference of symptomatic presentation.
13.1.4 Brachial Plexus Toxicity
Brachial plexus is an organ-at –risk in patients with lung tumors at apical locations. Tolerance dose of the brachial plexus in SBRT using high fractional doses is not well known. Recent investigation from Indiana University reported that 7 of 36 patients (19 %) with apical lung tumors who were treated with SBRT using median dose of 57 Gy in 3–4 fractions experienced Grade 2–4 brachial plexopathy after the treatment (range 6–23 months). The 2-year rate of brachial plexopathy is documented 46 % for maximum dose > 26 Gy versus 8 % for ≤ 26 Gy [35]. In the RTOG trial (0618), dose constraint for brachial plexus is defined ≤ 24 Gy (8 Gy/fraction).
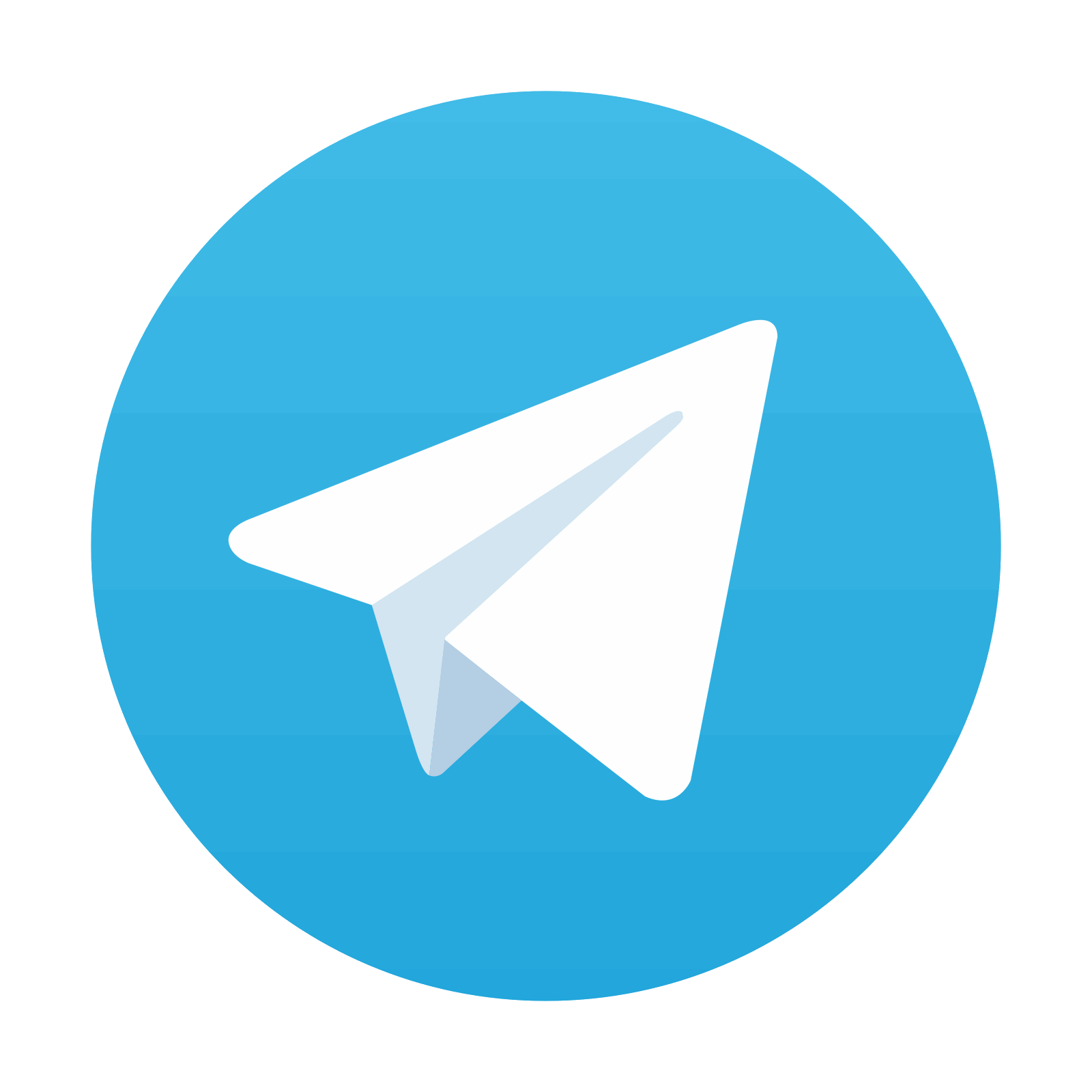
Stay updated, free articles. Join our Telegram channel

Full access? Get Clinical Tree
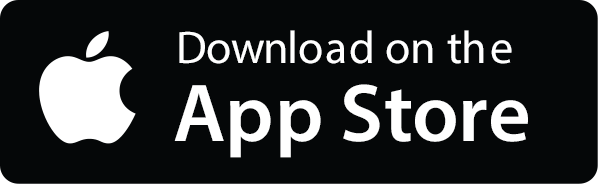
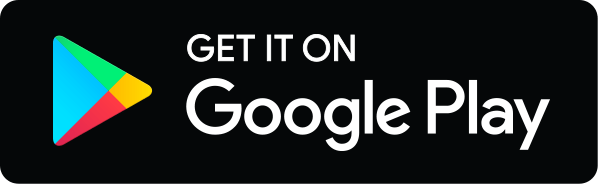