Transesophageal Echocardiography for Congenital Heart Disease in the Adult
INTRODUCTION
The spectrum of congenital heart disease (CHD) seen in the adult varies widely. Malformations range from mild anomalies requiring no intervention to extremely complex pathologies characterized by the presence of multiple coexistent defects. Echocardiography represents the primary noninvasive imaging modality in the assessment of these lesions. The transesophageal approach expands the applications of echocardiography by allowing the acquisition of anatomic and functional information that may not be obtainable by transthoracic imaging. This is of particular benefit to the adult individual with suboptimal transthoracic windows as transesophageal echocardiography (TEE) significantly enhances the characterization of structural defects and evaluation of hemodynamics. Additional major contributions of TEE in CHD include the intraoperative assessment of the surgical repair, detection of residual pathology, and guidance of the intervention if an immediate revision is necessary. TEE also plays an important role in the cardiac catheterization laboratory as an adjunct to therapeutic interventions in patients with CHD.
Until the last several years most of the TEE experience was limited to two-dimensional (2D) imaging and complementary modalities; however, recent advances have resulted in three-dimensional TEE (3D-TEE) being increasingly applied to all forms of heart disease. This includes the evaluation of congenital lesions. The high-resolution spatial anatomic information provided, together with the demonstration of salient features of the pathology from unique views, exceeds the capabilities of 2D-TEE imaging. This represents a distinct advantage in CHD. As the experience increases, 3D-TEE is likely to further facilitate diagnostic and therapeutic strategies in patients affected by structural cardiovascular malformations.
This chapter focuses on selected anomalies in the adult with CHD and addresses corresponding applications of TEE. The role of this imaging modality in the intraoperative and cardiac catheterization settings is highlighted. Although many of the defects can be fully characterized using the standard TEE views recommended by the Society of Cardiovascular Anesthesiologists and American Society of Echocardiography in the comprehensive guidelines, in some cases the detailed examination of the abnormalities, particularly in the case of complex disease, requires modified planes of interrogation. Another important aspect of TEE in CHD is the need not only for specific views but also sweeps that display the anatomic and spatial relationship among structures. As relevant to the pathology being considered, these modified cross sections/sweeps will be described. The initial 3D-TEE experience in the adult with CHD is also briefly reviewed.
CONGENITAL HEART DISEASE IN THE ADULT: INCIDENCE, PREVALENCE, AND SURVIVAL
The incidence of CHD in the United States is estimated to be 6.2 per 1,000 live births. This figure does not include the bicuspid aortic valve (Bic AV), regarded as the most common form of CHD occurring in 2% to 3% of the general population. At birth, the most common cardiac structural abnormality is that of a ventricular septal defect (VSD). Other relatively common congenital lesions include atrial septal defects (ASDs), pulmonary stenosis (PS), and patent ductus arteriosus (PDA). Beyond these defects, other less prevalent pathologies include tetralogy of Fallot (TOF), aortic stenosis (AS), coarctation of the aorta (CoA), atrioventricular septal defects (AVSDs), and transposition of the great arteries (TGA).
Not surprisingly, the highest survival rate in CHD occurs among infants with milder forms of disease. However, overall outcome in those with complex pathology has improved dramatically over the years. This is attributed to factors such as prenatal diagnosis, advances in medical and surgical strategies, definitive surgical repair at an earlier age, and improvements in intraoperative/postoperative care. The prevalence of CHD is approximately 4 per 1,000 living adults, of which nearly 10% have complex CHD. Currently, there are more adults than children with CHD in the United States accounting for an estimated population of nearly 2 million adults. This group of patients is also referred to as the “Grown-Up with CHD (GUCH).” It is anticipated that the number of adults with congenital cardiovascular malformations will continue to increase worldwide, as well as the complexity of this patient group.
CARDIAC EMBRYOLOGY AND DEFECTS RESULTING FROM ABNORMAL CARDIAC DEVELOPMENT
Familiarity with cardiac embryology and normal development facilitates the understanding of abnormal cardiovascular anatomy and resultant pathology in CHD. Thus, a brief review is relevant to the discussion of these lesions that follows.
The earliest developmental stage of the heart and vascular system is seen following the second week of gestation. By the middle of the third week clusters of angiogenic cells develop and give rise to vascular structures in the human embryo. Over time, these cells form two endothelial tubes that fuse to form a single heart tube. This eventually differentiates into components that include the sinus venosus, atrium, primitive ventricle, and bulbus cordis (Fig. 19.1A).
FIGURE 19.1 Heart tube. A: Left panel: The figure illustrates in graphical form the various components of the single heart tube that include the sinus venosus, atrium, primitive ventricle, and bulbus cordis regions. Right panel: The early stages of bending or looping of the heart tube within the pericardial sac are shown. The arrows note the usual direction of looping. B: Graphic representation following completion of looping of the heart tube. Note the cephalad migration of the atria, unseptated common outflow tract, and orientation of the convex surface of the heart toward the right.
Initially a short and straight structure (single midline heart tube) undergoes a process of coordinated rapid growth within the pericardial sac resulting in bending, rotation, and torsion, otherwise known as looping. This leads to migration of the atria in a cephalad direction and orientation of the convex surface of the heart to the right (Fig. 19.1B). The term “D-looping” is used to define this aspect of normal cardiac development.
Following looping of the heart tube, the sinus venosus region undergoes many changes while developing into the venous system of the heart. It begins as a paired structure and initially fuses to form a transverse sinus with right and left “horns” (Fig. 19.2A). As development continues, the right horn enlarges due to progressive shift of venous blood drainage to right-sided structures and the left horn becomes atretic. Ultimately, the right sinus horn becomes incorporated into the right atrium (RA) as the vena cava and the left sinus horn becomes the coronary sinus (Fig. 19.2B). The lack of regression of the left horn contributes to the persistence of a left superior vena cava (L-SVC).
FIGURE 19.2 Development of systemic veins. A: Posterior view of the primitive heart depicting the prominent right and left sinus horns and the transverse sinus. B: Posterior view of the developed heart. Note the coronary sinus, a remnant of the left sinus horn. The enlarged right sinus horn is now incorporated into the right atrium as the superior and inferior vena cavae.
The primitive atrium is undivided and communicates with the primitive ventricle that connects to the outlet bulbus cordis. Around the fourth week of human embryonic development, the process of cardiac septation begins involving the atrium, ventricle, atrioventricular junction and valves, outflow tracts, and semilunar valves. During atrial septation, the septum primum, formed by tissue ingrowth along the superior aspect of the primitive atria, extends inferiorly toward mesenchymal tissue swellings known as endocardial cushions (future atrioventricular orifice) in a curtain-like fashion. Initially, the septum primum leaves an inferior opening, the ostium primum, below its free edge. Subsequently, a second opening develops in the superior aspect of the septum primum referred to as ostium secundum. This provides for flow of highly saturated placental blood across the interatrial septum during fetal life, from the RA to the left atrium (LA). The septum primum then extends inferiorly, becoming continuous with developing endocardial cushions of the atrioventricular junction and obliterates the ostium primum (Fig. 19.3A). Around the fifth to sixth week of gestation, a second partition, the septum secundum, develops parallel and to the right of the septum primum and similarly extends inferiorly (Fig. 19.3B). The septum secundum covers the ostium secundum but forms an incomplete atrial partition. The formation of this incomplete atrial partition again ensures blood flow across the interatrial septum during fetal life. The remaining opening of the septum secundum is the foramen ovale (Fig. 19.3C). Tissue from the septum primum overlies the foramen ovale and forms a flap that closes when the left atrial pressure increases normally at birth. A patent foramen ovale (PFO) does not represent a defect of atrial septal tissue as such but rather an incompetent flap valve of the fossa, occurring in an estimated 25% of adults. The presence of a PFO has been implicated in the etiology of migraines and strokes. ASDs can be the result of excessive resorption of septal tissue leading to deficient septum primum (secundum defects), lack of fusion of the septum primum with absent endocardial cushion (primum defects), or abnormal development of the sinus venosus portion of the atrial septum (sinus venosus defects).
FIGURE 19.3 Stages of atrial septation. A: As the septum primum grows inferiorly toward the endocardial cushions, the ostium secundum, labeled as perforations in upper septum primum, forms in the posterior portion of the septum primum. Once the ostium secundum is formed it ensures the flow of blood across the atrial septum. Thereafter, the septum primum completes its growth and becomes continuous with the developing endocardial cushions of the atrioventricular junction (see arrows). B: The septum secundum develops parallel and to the right of the septum primum. It forms an incomplete partition. C: The remaining opening in the septum secundum is known as the foramen ovale. It is covered by a flap valve formed from tissue from the septum primum. Normally this flap valve closes when pressure in the left atrium increases, exceeding right atrial pressure, following birth.
Septation of the atrioventricular canal begins as the endocardial cushions enlarge and fuse. This occurs concurrently with completion of the septum primum and expansion of the atrioventricular orifice. The cushions, initially muscular in nature, perform a valve-like function and through a process of cellular differentiation they become thin and membranous resulting in the formation of separate right and left atrioventricular valves. Altered development during this process is thought to lead to persistence of a common atrioventricular junction and contribute to atrioventricular valve abnormalities. Failure of normal fusion of the endocardial cushions results in canal-type defects.
Ventricular septation begins in the fifth week of gestation and is derived from a primordial muscular interventricular septum, outgrowths of endocardial tissue, as well as tissue originating from conus and truncal swellings (Fig. 19.4). Following fusion of these components, the ventricular septum is comprised of a small membranous portion and a large muscular component that is divided into inlet, trabecular, and outlet regions. Persistence of a small interventricular communication or incomplete formation of the septum can lead to VSDs.
FIGURE 19.4 Ventricular septation. The muscular interventricular septum grows in a dorsal direction toward the endocardial cushions. Subsequently, the membranous interventricular septum occurs as an outgrowth of endocardial tissue and portions derived from conus and truncal swellings.
The outflow tracts of the left ventricle (LV) and right ventricle (RV) are formed following septation or partitioning of the bulbus cordis and single truncus arteriosus. This process includes the formation of bulbar and truncal ridges, spiraling, and resultant creation of a spiral aortopulmonary septum that separates the future aorta (Ao) from the pulmonary artery (PA). The semilunar valves are derived from subendocardial tissue swellings in the arterial trunks. Defects of conotruncal development, semilunar valve formation, and aortopulmonary septation account for a large number of congenital defects (TOF, TGA, truncus arteriosus, and aortopulmonary window).
The coronary arteries appear relatively late during cardiac development. Subepicardial vascular networks during the fifth week of development are thought to give rise to the distal coronary vessels. The origin of the proximal coronary vasculature is more controversial. The aortic sac, aortic arches, and dorsal Ao contribute to the development of the mature aortic arch through an orchestrated series of events that if altered can result in vascular anomalies.
CLASSIFICATION OF CONGENITAL HEART DISEASE
In view of the variable spectrum of pathology, several classification schemes have been proposed to facilitate the understanding of CHD and the physiologic impact of these defects. Lesions can be characterized, among many ways, according to severity of disease, presence or absence of cyanosis, or primary physiologic alteration.
Based on Severity of Disease
Defects have been stratified according to their severity or level of complexity into simple, moderate severity, or complex defects. This classification scheme has been utilized for recommendations regarding patient care, anticipation of long-term problems, and expectation of potential outcomes. Intracardiac communications in their isolated forms represent in most cases simple defects. Complex pathology includes all forms of cyanotic CHD, lesions associated with multiple concomitant defects, and malpositions of the heart/viscera (heterotaxy syndromes).
Based on the Presence or Absence of Cyanosis
In this scheme, congenital cardiac malformations are divided into acyanotic or cyanotic lesions based on whether the primary functional disorder results in cyanosis. Conditions associated with cyanosis are characterized by restrictive pulmonary blood flow (in the presence of intracardiac shunting) or complete arterial and venous admixture. Cyanosis is less likely to occur in individuals with pulmonary overcirculation secondary to isolated intracardiac communications.
Based on Physiology of the Defect
The classification algorithm based on the physiologic spectrum of CHD comprises four major categories: Shunts, obstructions to either pulmonary or systemic blood flow, regurgitant pathology, and mixed lesions. Shunt lesions occur within the heart (intracardiac) or outside the heart (extracardiac). The direction and magnitude of shunting depend on the size of the communication and the relative resistances of the pulmonary and systemic circulations. Obstructive lesions can affect the inflow or outflow of blood and vary widely in severity. Regurgitant disease is rarely found in isolation and is frequently secondary to the primary pathology. In mixed lesions, which account for a significant number of cyanotic heart defects, there is mixing of the systemic and pulmonary venous returns.
SPECIFIC CONGENITAL HEART DEFECTS
The section that follows provides an overview of selected congenital heart defects. A brief discussion of anatomy, pathophysiology, and management is presented followed by the detailed TEE evaluation of each anomaly. This information is summarized in Tables 19.1 and 19.2.
Atrial Septal Defect
Anatomy
The term ASD is used to refer to lesions that result in atrial level shunting. This may not necessarily imply a deficiency of the atrial septum itself. Four main types include ostium secundum, ostium primum, sinus venosus, and coronary sinus defects (Fig. 19.5). ASDs account for approximately 30% of all cases of CHD detected in adults and in general are more common in females.
Ostium secundum defects are commonly located in the central portion of the interatrial septum in the region of the fossa ovalis accounting for 70% of all atrial communications (Fig. 19.6, Video 19.1). Associated abnormalities include mitral valve prolapse and mitral regurgitation.
Ostium primum defects (also known as partial AVSDs) are located in the inferior aspect of the interatrial septum (Fig. 19.7, Video 19.2). They account for approximately 20% of ASDs and are associated with a cleft in the anterior mitral leaflet and mitral regurgitation. This defect is considered within the spectrum of AVSDs and may be seen in patients with Down syndrome, although the complete form of the defect is more frequently the case.
Sinus venosus defects occur posteriorly adjacent to the entrance of the superior vena cava (SVC) or inferior vena cava (IVC) into the RA (Fig. 19.8, Video 19.3). The superior defect is the most common type. They account for 5% to 10% of ASDs. In this lesion, straddling of the caval vein over the atrial septum is commonly seen. These defects are often associated with partial anomalous pulmonary venous drainage from the right lung due to deficiency of the wall that normally separates the veins and LA leading to pulmonary vein unroofing.
TABLE 19.1 Congenital Heart Disease in the Adult: Physiology, Prevalence, Associated Lesions, Treatment, and Prognosis
TABLE 19.2 Transesophageal Echocardiography in Congenital Heart Disease
FIGURE 19.5 Atrial septal defects. The graphic representation depicts the typical location of the various interatrial communications as follows: Centrally located ostium secundum defect, inferiorly located ostium primum defect, sinus venosus defect near either the superior vena cava (SVC) or inferior vena cava (IVC) entrance and frequently associated with anomalous pulmonary venous drainage (arrow), and coronary sinus defect. RA, right atrium; RV, right ventricle. (From Perloff JK. The Clinical Recognition of Congenital Heart Disease. 4th ed. Philadelphia, PA: WB Saunders; 1994:293–380, reproduced with permission.)
FIGURE 19.6 Secundum atrial septal defect. Left panel: Midesophageal four-chamber view depicting a moderate size central defect in the atrial septum (arrow), typical of a secundum atrial septal defect. Right panel: Corresponding color Doppler interrogation demonstrating left-to-right atrial level shunting (blue flow) across the defect. LA, left atrium; LV, left ventricle; RA, right atrium; RV, right ventricle.
FIGURE 19.7 Primum atrial septal defect. Left panel: Midesophageal four-chamber view depicting a relatively small defect in the inferior aspect of the atrial septum (arrow), location characteristic of a primum atrial septal defect. Right panel: Corresponding color Doppler interrogation demonstrating left-to-right atrial level shunting across the defect. An aneurysm, also known as a tricuspid pouch, is seen billowing into the right ventricle (RV) representing remnants of endocardial cushion tissue. LA, left atrium; LV, left ventricle; RA, right atrium.
FIGURE 19.8 Sinus venosus atrial septal defect. Left panel: Midesophageal bicaval view depicting a communication in the interatrial septum near the entrance of the superior vena cava (arrow). The findings are typical of a superior vena cava-type sinus venosus atrial septal defect. Right panel: Corresponding color flow mapping demonstrating left-to-right atrial level shunting across the communication. The superior vena cava frequently overrides this type of defect, frequently associated with anomalous pulmonary venous drainage. LA, left atrium; RA, right atrium.
FIGURE 19.9 Atrial septal defect occluder device. Midesophageal aortic valve short-axis view with rightward transducer rotation demonstrating an atrial septal defect closure device straddling the interatrial septum. AO, aorta; LA, left atrium; RA, right atrium; RV, right ventricle.
Coronary sinus defects result from a communication between the LA and the coronary sinus (coronary sinus septum). These defects are relatively rare (less than 2% of ASDs) and frequently occur in association with other malformations. They are typically seen within the context of a persistent L-SVC draining to an unroofed coronary sinus. The orifice of the coronary sinus in this setting is usually large.
Pathophysiology
The physiologic consequence of an ASD is determined by the degree of shunting (predominant direction is usually left to right). The defect size, ventricular compliances, and PA pressures determine the magnitude of the shunt. A large defect leading to pulmonary overcirculation results in right-sided diastolic volume overload manifested as RA, RV, and PA dilation. Over time, atrial arrhythmias and heart failure can develop. Mild-to-moderate elevations of PA pressure can be seen in older patients; however, severe pulmonary hypertension rarely occurs. Adults may remain asymptomatic and an ASD may represent an incidental finding on echocardiography.
Management
Most patients with large defects undergo surgical closure. Selected ostium secundum defects may be amenable to percutaneous closure in the cardiac catheterization laboratory (Fig. 19.9, Video 19.4). Suitability for transcatheter device occlusion of secundum ASDs includes size of the defect (<38 mm) and the presence of adequate rims of surrounding atrial septal tissue. Transcatheter device closure can also be considered in patients with a PFO and a history of cerebrovascular events in order to limit the risk of paradoxical emboli. Device closure of other types of interatrial communications is not feasible due to the presence of critically important structures surrounding the defects that may be compromised by the occluder.
Transesophageal Echocardiographic Evaluation
Suggested cross sections for a focused examination include midesophageal (ME) four-chamber (4 CH), ME bicaval, and ME AV short-axis (SAX).
Goals of the two-dimensional examination are the following:
• Definition of defect location and size (detection of a PFO or small interatrial communication may require the intravenous injection of agitated saline or other contrast material and a Valsalva maneuver to identify right-to-left shunting)
• Determination of defect rims (5 mm ideally):
– Anterosuperior (aortic rim): ME AV SAX view, distance between aortic ring and defect (lack of rim does not preclude device deployment)
– Posterior: ME 4 CH view, gap between coronary sinus and defect
– Inferoanterior: ME 4 CH, space between the defect and atrioventricular valves
– Superior-posterior: ME bicaval view, distance between the SVC and defect
– Inferoposterior: ME bicaval view, gap between the IVC and defect
• Assessment of mitral valve anatomy (evaluate for prolapse/cleft: Prolapse may occur in association with secundum ASDs; a cleft in the anterior leaflet is commonly seen in partial AVSDs)
• Evaluation of pulmonary venous drainage (all cases, but particularly, sinus venosus ASDs)
• Examination of right-sided structures for dilation and motion of interventricular septum (flattened or paradoxical motion related to volume load)
• Evaluation of RV wall thickness, suggestive of pulmonary hypertension
• Assessment of ventricular function
Goals of the Doppler examination are the following:
• Assessment of flow across defect: Nature, direction, and velocity
• Detection of tricuspid, mitral, and pulmonary valve (PV) regurgitation
• Measurement of tricuspid regurgitant jet velocity (VTR) for estimation of PA systolic pressure as follows:
RV systolic pressure = 4(VTR)2 + RA pressure
In the absence of RV outflow obstruction:
PA systolic pressure = RV systolic pressure
• Interrogate pulmonary venous flows
• Estimation of shunt magnitude (pulmonary and systemic blood flows) in the absence of significant atrioventricular valve regurgitation
Goals of the examination during transcatheter device closure are the following:
• Determination of tissue rims (lack of inferior or posterior rim unfavorable)
• Assessment of relation of defect to surrounding anatomic structures
• Measurement of maximal defect diameter (balloon stretch sizing)
• Detection of leaks around the balloon
• Guidance for device deployment/device stability
• Assessment of residual shunting
Goals of the examination after surgical repair/catheter intervention are the following:
• Detection of residual interatrial shunting
• Evaluation of atrioventricular valve competence
• Assessment of ventricular function
• Evaluation of proper positioning of device during transcatheter closure (unobstructed flow in systemic and adjacent pulmonary veins following device deployment)
• Exclusion of complications related to the intervention (for device: Embolization, erosion)
Applications of 3D imaging are the following:
• Enhances spatial details of the defect (size, location, rims, relationships between defect/device, and adjacent anatomic structures)
• Facilitates continuous visualization of the 3D relations while monitoring ASD device deployment (Fig. 19.10, Video 19.5)
FIGURE 19.10 Three-dimensional transesophageal echocardiographic image of atrial septal defect occluder device. The image displays the right atrial disc and the delivery cable prior to release of the closure device.
• Evaluates for appropriate ASD device position and entrapment of septal rims around the occluder
• Allows for acquisition of ventricular volumes, ejection fraction
VENTRICULAR SEPTAL DEFECT
Anatomy
VSDs are classified by location into four major groups: Perimembranous, muscular, doubly committed outlet and inlet defects (Fig. 19.11). They can occur in isolation or as part of complex malformations. An isolated VSD is the most common congenital cardiac pathology diagnosed in infancy. Since 60% of smaller defects close spontaneously and larger defects are usually repaired in childhood, VSDs account for only 10% to 15% of defects observed in adults with CHD.
FIGURE 19.11 Ventricular septal defects. Graphic rendering of interventricular septum as seen from the right ventricular aspect. Ventricular septal defects are classified by location into four major groups: Perimembranous, muscular, doubly committed (subarterial), and inlet defects. Muscular defects can occur anywhere in the trabecular or inlet portion of the interventricular septum. In this figure, a portion of the aortic valve can be visualized through the perimembranous defect.
Perimembranous defects account for approximately 70% of VSDs, involve most or all of the membranous septum, and may extend into the muscular region. Associated findings can include an aneurysm of the membranous septum that is composed of tricuspid valve tissue. On echocardiography this appears as a tissue pouch and often limits shunting across the defect (Fig. 19.12, Video 19.6). Other pathology may consist of a subaortic membrane or AV cusp herniation/prolapse (resulting in aortic regurgitation). A perimembranous VSD may also be seen in the presence of obstruction within the RV cavity related to muscular band hypertrophy that divides the RV into two chambers (double-chambered right ventricle or DCRV).
FIGURE 19.12 Perimembranous ventricular septal defect. Left: Midesophageal four-chamber view depicting a large defect in the membranous region partially covered by aneurysmal tissue (arrow). Right: Corresponding color Doppler flow mapping demonstrating a significant amount of ventricular level left-to-right shunting across the defect. LA, left atrium; LV, left ventricle; RA, right atrium; RV, right ventricle.
Muscular defects are defined by their location in the muscular portion of the ventricular septum. They account for 20% of VSDs, can be isolated or multiple (“Swiss cheese”-type) and are often located in the central or apical portion of the trabecular septum (Fig. 19.13, Video 19.7).
FIGURE 19.13 Muscular ventricular septal defects. Image displays two muscular ventricular septal defects (VSDs) as demonstrated in a transgastric left ventricular short-axis cross section. Two defects are seen, a larger one in the midmuscular region and a second, smaller one at the apex. LV, left ventricle; RV, right ventricle.
Doubly committed outlet defects (also known as supracristal, subarterial, subpulmonary, infundibular, or conal VSDs) are located in the infundibular septum immediately below the PV. They account for 5% of VSDs and are frequently associated with AV prolapse resulting in regurgitation (Fig. 19.14, Video 19.8).
FIGURE 19.14 Supracristal (conal, subarterial) ventricular septal defect. Left panel: Midesophageal aortic valve long-axis view demonstrates prolapse of the aortic valve (right coronary cusp) through a subarterial ventricular septal defect (arrow). Right panel: Systolic frame demonstrating shunting across the ventricular communication. Ao, aorta; LA, left atrium; LV, left ventricle; RVOT, right ventricular outflow tract.
Inlet defects account for approximately 5% of VSDs and are located in close proximity to the atrioventricular valves in the posterior or inlet portion of the ventricular septum (Fig. 19.15, Video 19.9). These defects predominate in patients with Down syndrome. An associated primum ASD within the context of a common atrioventricular valve annulus is part of the defect known as a complete AVSD (canal defect or endocardial cushion defect). As previously noted, these are also commonly seen in individuals with Down syndrome.
FIGURE 19.15 Inlet ventricular septal defect. Midesophageal four-chamber view depicting a large ventricular septal defect (VSD) in the inlet portion of the ventricular septum (arrow). Note the fact that both atrioventricular valves appear to be at the same level in contrast to the normal offset where the tricuspid valve has a more apical insertion into the ventricular septum as compared to the mitral valve. This is a typical finding in this type of defect. LA, left atrium; LV, left ventricle; RA, right atrium; RV, right ventricle.
Pathophysiology
The physiologic consequences of a VSD are determined by the size of the defect and the pulmonary vascular resistance. Moderate-to-large defects are associated with significant left-to-right shunting, left heart dilation, and symptoms of heart failure. Pulmonary vascular changes can occur leading to severe pulmonary hypertension in patients with large, long-standing VSDs and substantial pulmonary overcirculation. This in turn can lead to reversal in the direction of the shunt through the defect (right-to-left shunting) and resultant cyanosis. Increased pulmonary vascular resistance secondary to irreversible vascular changes leads to a condition known as Eisenmenger syndrome. Affected adults have a decreased survival rate and generally are not considered candidates for surgical repair.
Management
Most symptomatic patients require intervention. Surgical closure of an isolated VSD is most frequently accomplished through a transatrial or transpulmonary approach. Thus, the location of the communication has important implications for surgical access to the defect. In selected cases transcatheter device occlusion may be an option. This has been more commonly applied to muscular communications due to the distant anatomic relationship of this type of defect to the atrioventricular valves/outflow tracts (Fig. 19.16, Video 19.10). In addition, these types of defects (particularly if multiple) can present significant challenges for surgical closure. A catheter-based intervention can be accomplished either percutaneously in the cardiac catheterization laboratory or in the operating room using a perventricular hybrid approach. The latter strategy uses a combination of surgical and interventional techniques. During a typical procedure, following a sternotomy, the free wall of the RV is punctured with a needle, a wire is introduced and a sheath is placed across the wire. TEE guides placement of the device across the defect. Surgery for associated pathology can then be performed using conventional techniques.
FIGURE 19.16 Muscular ventricular septal defect occluder device. A ventricular septal defect (VSD) occluder device is seen (arrow) in the midmuscular portion of the ventricular septum in this transgastric short-axis view. LV, left ventricle; RV, right ventricle.
Transesophageal Echocardiographic Evaluation
Suggested cross sections for a focused examination include ME 4 CH, ME AV long-axis (LAX), ME right ventricular inflow–outflow (ME RV in–out), and deep transgastric (TG) LAX.
Goals of two-dimensional examination are the following:
• Assessment of defect location, size, and extension
• Inspection for associated abnormalities, including septal aneurysm
• Evaluation of AV for deformity (herniation and prolapse)
• Examination of PAs and left-sided cardiac structures for dilation
• Inspection for findings suggestive of pulmonary hypertension (RV wall thickness and configuration of interventricular septum during systole)
Goals of the Doppler examination are the following:
• Confirmation of the presence of the defect, evaluation for additional defects
• Determination of the nature, magnitude, and direction of shunt flow
• Detection of tricuspid and/or aortic regurgitation (color Doppler)
• Estimation of PA systolic pressure by:
– Tricuspid regurgitant jet peak velocity (VTR)
– VSD jet peak velocity (VVSD):
RV systolic pressure = Systolic blood pressure – 4(VVSD)2
In the absence of RV outflow obstruction:
RV systolic pressure = PA systolic pressure
In the absence of LV outflow obstruction:
Systemic systolic blood pressure = LV systolic pressure
• Determination of flow restriction across VSD (high-velocity VSD jet suggests restriction vs. low-velocity flow of a nonrestrictive defect)
• Estimation of the magnitude of the shunt (pulmonary to systemic blood flow ratio) in the absence of significant atrioventricular valve regurgitation
Goals of the examination during transcatheter device closure are the following:
• Determination of VSD anatomy, size, and relationship to ventricular inflows and outflows
• Evaluation of valvar competence
• Detection of leaks around the balloon, if balloon sizing is performed
• Early detection of complications related to device positioning
• Guidance and monitoring during device deployment
• Assessment of leaks across device
Goals of the examination after surgical repair/catheter intervention are the following:
• Detection of residual shunts
• Determination of potential changes in valvar regurgitation
• For percutaneous intervention: Evaluation of device position in septum and relative to adjacent structures
• Assessment of ventricular function
PATENT DUCTUS ARTERIOSUS
Anatomy
During fetal life, the arterial duct connects the PA to the descending Ao (Fig. 19.17) near the level of the left subclavian artery. This communication allows for RV output into the Ao bypassing the lungs under normal fetal conditions of increased pulmonary vascular resistance. Failure of closure of this communication in the neonatal period results in persistent ductal patency. This lesion accounts for approximately 8% of cases of CHD. As in many other defects, a PDA can be found in isolation or associated with additional cardiac defects.
FIGURE 19.17 Patent ductus arteriosus. Diagram depicting the ductus arteriosus as it connects the junction of the main and left pulmonary arteries to the descending aorta in the region adjacent to the origin of the left subclavian artery.
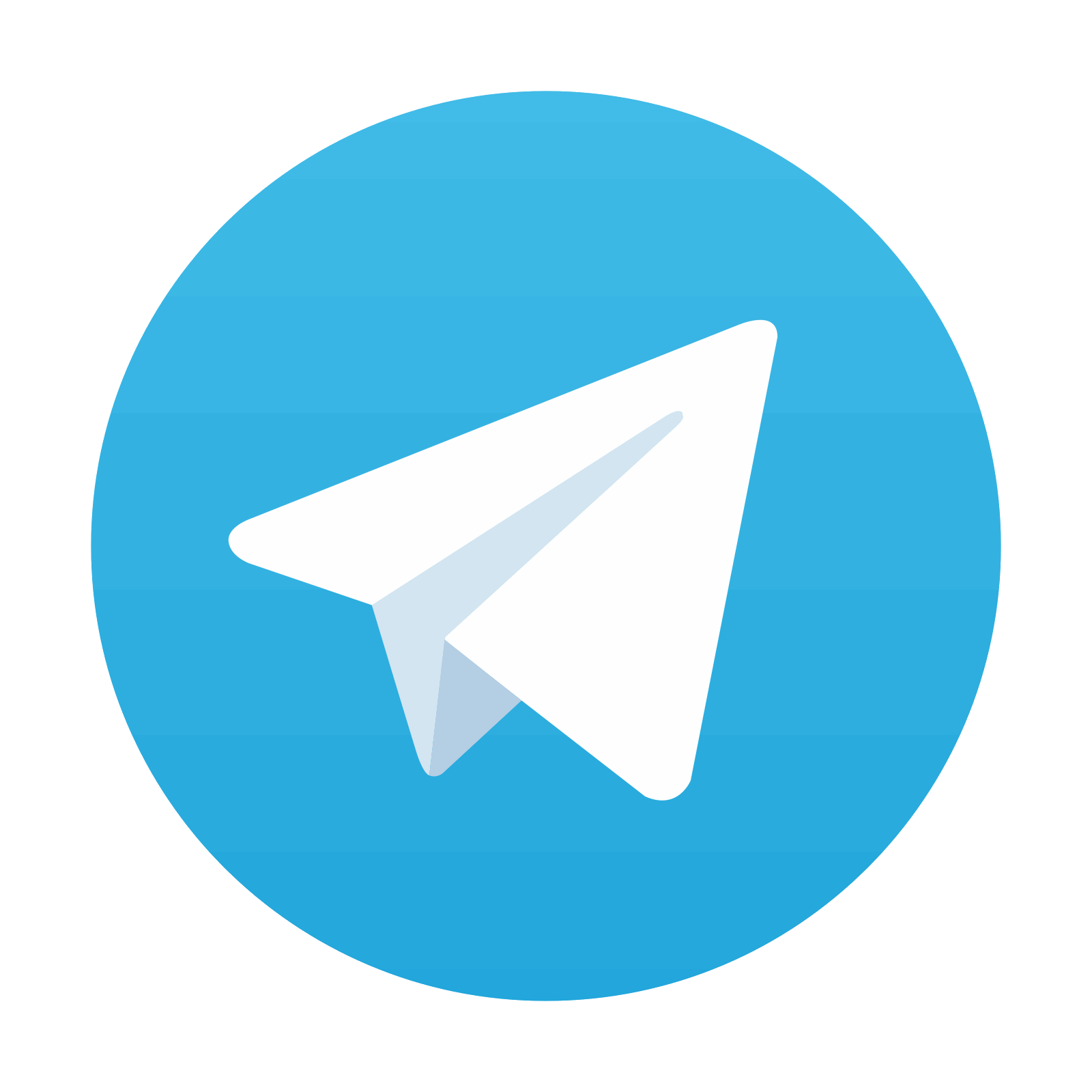
Stay updated, free articles. Join our Telegram channel

Full access? Get Clinical Tree
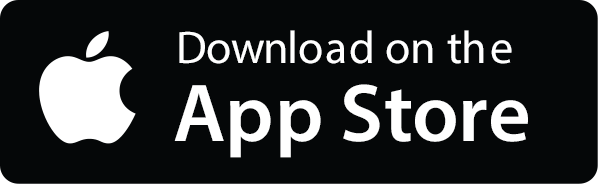
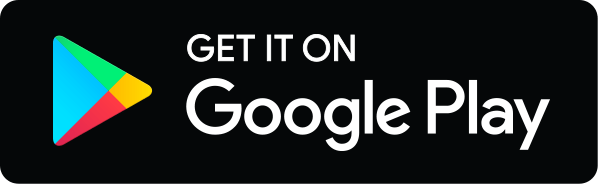