Key Facts
- •
Muscle is a dynamic tissue that can be subject to direct and indirect traumatic injuries.
- •
Type 1 muscle fibers contain more numerous mitochondria, enabling longer, sustained contractions that are more resistant to fatigue (e.g., soleus). Type 2 fibers elicit faster, shorter, more powerful contractions, which are optimal for short energy bursts and tend to hypertrophy with training (e.g., hamstring muscles).
- •
In skeletally immature individuals, the apophyseal growth plate represents the weakest point along the myotendinous unit.
- •
Delayed-onset muscle soreness is a symptom of exercise-induced, indirect muscle damage that typically begins gradually, approximately 8 to 12 hours postexercise.
- •
Myotendinous strain injuries are among the most common activity-related indirect traumatic muscle injuries. Acute injury occurs at the myotendinous junction and is classified as first degree (stretch injury), second degree (partial tear), or third degree (complete rupture).
- •
Direct traumatic muscle injuries include contusions and lacerations.
- •
Tendinopathy denotes a continuum of disorders of the tendon including degeneration (tendinosis), partial tear, and complete rupture, which develop over an extended period of time.
- •
Complications of traumatic muscle injuries include hematoma formation, myonecrosis, and myositis ossificans.
- •
Calcification in myositis ossificans may take at least 6 to 8 weeks to be seen, making follow-up radiographs or computed tomography useful. Because calcification is difficult to see on magnetic resonance imaging, the appearance of myositis ossificans may be confused with other lesions including tumors.
The late 20th and early 21st century societies have seen an increase in consumer health consciousness and physical fitness as individuals are in constant pursuit of longer and healthier lives. It is generally widely accepted, and evidence continues to show that physical activity is associated with overall good mental and biologic health. A regular exercise regimen is associated with reduced risk of death and morbidity from a number of major diseases including cardiovascular, which has ranked as the number one cause of mortality in society for a number of years. Coincident with a rise in the so-called baby boomer and elderly generations, an increasing number of individuals are engaging in exercise and participating in sports, activities that have traditionally been associated more with the adolescent, young adult, amateur, or professional athlete. Although health benefits are seen with moderate, well-balanced activity programs, enthusiastic and sometimes overzealous “weekend warriors” challenge themselves and the limits of their anatomy to the breaking point, literally. Pain and disability result as a consequence of traumatic injury, which is often muscular in nature.
This chapter focuses on the spectrum of traumatic muscle injuries. A large percentage of traumatic muscle injuries are frequently clearly associated with precipitating activity, as in the setting of the acute muscle strains. More direct forms of traumatic muscle injuries include lacerations and contusions. Although imaging plays a limited role in diagnosis in the acute setting when a clear temporal relationship between an activity or direct injury correlates with the onset of pain and loss of function, it may assist in grading the extent and severity of injury. Imaging may assist in treatment planning, performance prognosis, and evaluation of delayed complications such as myositis ossificans. In clinical circumstances where confounding history or delayed presentation hinders the practitioner’s diagnosis, imaging may play a crucial role in patient assessment. For instance, a number of neoplastic or inflammatory conditions can frequently masquerade as muscle injury. For these reasons, patients are often referred for imaging evaluation.
In order to recognize the appearance of traumatic muscle injuries, one must have an understanding of the normal anatomic structure and organization of muscle, and this chapter will begin with an overview of muscle morphology and risk factors for muscle injury. Imaging techniques will be discussed briefly, with an emphasis on magnetic resonance (MR) technology as the imaging modality of choice. The remainder of the chapter will then address specific traumatic muscle injuries. Common acute injuries to skeletal muscle include strains and contusions. The importance of myotendinous strain will be emphasized, given that this represents the most frequently encountered form of traumatic muscle injury in clinical practice.
ANATOMIC CONSIDERATIONS AND RISK FACTORS
Muscle is a biologic tissue whose structure is uniquely tailored for force generation and movement. Based on percentage mass, muscle is the single largest tissue in the body, comprising up to 50% of the total body weight in some individuals. The basic structural unit of muscle is the myofiber, which consists of a multinucleated cell that is the smallest complete contractile system. In the maturation of muscle fibers, a unique characteristic metabolic profile may develop, dividing structural muscle units into type 1 and type 2 fibers. Every muscle is composed on a combination of both types of fibers in varying percentages that helps to define its metabolism. Type 1 fibers contain more numerous mitochondria, enabling longer, sustained contractions that are more resistant to fatigue. A good example of muscle dominant in type 1 fibers would include a postural muscle such as the soleus. Type 2 fibers elicit faster, shorter, more powerful contractions, which are optimal for short energy bursts and tend to hypertrophy with training, as in the hamstring muscles.
Numerous myofibers are bundled into fascicles, which in turn are grouped into the entities we recognize as individually named muscles. Highly compartmentalized skeletal muscles are architecturally arranged in a manner to establish their functional properties, which in the majority of instances is to produce joint motion. Compartmentalized muscle is generally bounded by fascia, a tough connective tissue investment. In addition to maintaining the structural integrity of muscle, fascia plays an important role in the pathogenesis of muscle disorders such as muscle herniation and compartment syndrome and influences the patterns of spread of neoplastic and infectious processes.
Muscles function to produce movement by acting across joints. Most muscles cross one joint, but a number of muscles span two joints. Methods of generating force across joints include concentric and eccentric muscle contractions. Concentric contraction occurs when the load acting on the muscle is less than the maximum tetanic tension generated by that muscle, resulting in active muscle shortening, as one might witness with biceps curls. Eccentric contraction occurs when the force on a muscle increases beyond the maximum force that muscle is capable of generating, resulting in active muscle lengthening.
With respect to activity-related traumatic injuries, and with myotendinous strain injuries predominating, the most commonly affected muscles tend to involve those that cross two joints. As a result, these muscles are generally activated as they are being stretched, resulting in a propensity for eccentric contractions. These muscles also tend to possess a greater proportion of type 2, fast twitch fibers. Muscles most susceptible to injury during rapid acceleration and speed activities based on these biomechanical and anatomic considerations include the hamstrings (i.e., semimembranosus), gastrocnemius, and rectus femoris.
Muscles that cross two joints, such as the hamstrings, are most susceptible to myotendinous strain injuries.
A multitude of other risk factors are known to play a role in traumatic and, more specifically, activity-related muscle injury. A history of muscle strain injury represents one of the most recognized risk factors for future insult to that muscle. Inadequate or overzealous rehabilitation resulting in early return to activity before complete healing is another recognized risk factor for injury. Poor conditioning, insufficient muscle strength, abrupt higher performance activity level, and increasing age are among some of the other known clinical risk factors.
Risk factors for activity-related muscle injury include a history of muscle strain, return to activity before complete healing, poor conditioning, insufficient muscle strength, abrupt increase in activity level, and increasing age.
Another feature to be considered in muscle injuries is the viscoelasticity properties of skeletal muscle. A simplified explanation of this concept is that repetitive stretching of a muscle reduces the load on the muscle-tendon unit at any given length, thereby increasing its viscoelasticity profile. Viscoelasticity is also temperature dependent. These principles provide a simplified explanation for the way warm-up combined with stretching prior to exercise seems to be beneficial in improving the viscoelasticity profile of muscle that, in turn, is protective against strain injuries.
Finally, in discussing the pathophysiology of traumatic muscle injuries, the importance of the myotendinous unit should be understood. The myotendinous unit consists of the muscle, the myotendinal (muscle-tendon) junction, the tendon, and the osseous attachment sites of the tendon. The myotendinous unit transmits the forces generated by muscles to their points of attachment to bone via the tendons of origin and insertion. When muscle injury occurs, the severity and location of injury along the entire myotendinous unit is influenced by a multitude of factors, including age; the rate, magnitude, and duration of stress loading; and the presence of underlying disorders such as tendinopathy. Normal tendons without underlying disease are much stronger than either their osseous attachment sites or the myotendinal junction.
The myotendinal junction contains interdigitating tendinous fibers amongst finger-like processes of skeletal muscle linked by a thick basement membrane ( Figure 12-1 ). Despite its complex ultrastructure, the myotendinal junction represents the relative weak point of the normal myotendinous unit. The myotendinal junction possesses poor intrinsic viscoelastic properties and lower capacity for energy absorption when compared with muscle, tendon, or its osseous attachments, thereby placing it at great risk for acute strain injury.
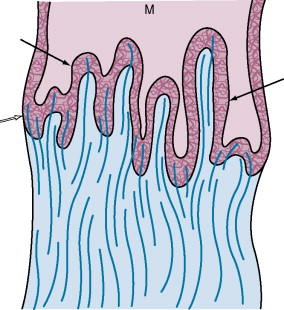
The myotendinous junction is the area most vulnerable to acute strain injury.
IMAGING CONSIDERATIONS IN THE DIAGNOSIS OF TRAUMATIC MUSCLE INJURIES
Acute traumatic muscle injuries are mostly self-limited and generally do not require imaging for diagnosis or management. The diagnosis begins with a careful history, taking close note of the relationship of onset of symptoms with the occurrence of trauma. Physical examination is confirmatory when there is evidence of swelling or ecchymosis and functional muscle testing reveals a loss or limitation of function supporting the clinical history. However, there are certain clinical circumstances where imaging might provide important information to the evaluating clinician ( Box 12-1 ). For example, in high-performance or professional athletes, imaging may help to determine whether a conservative treatment regimen or surgical repair is indicated. Imaging in these instances helps to grade the severity and localize the extent of injury. The additional information garnered through imaging helps in assessing the individual’s risk of possible re-injury if an insufficient treatment regimen is prescribed. Another situation whereby imaging plays an important role is in determining the presence of underlying tendinopathy as a potential risk factor for future injury. Muscle pain or a soft tissue mass of uncertain etiology in the setting of confounding history or without a specific inciting traumatic incident would also be important to assess with imaging, as specific findings may direct the radiologist to suggest an unsuspected muscle injury (rather than a tumor) as a source. Finally, potential complications of muscle injuries, such as hematoma, herniation, and myositis ossificans, can also be evaluated by imaging.
In a high-performance athlete to determine whether conservative or surgical treatment is appropriate
Determining the presence of underlying tendinopathy
Muscle pain or mass of uncertain cause
Detecting complications of muscle injury (hematoma, herniation, myositis ossificans)
The imaging of traumatic muscle injuries, as in all facets of musculoskeletal imaging, has evolved over the past several decades. Radiography can be helpful in the acute setting if associated bony injury is also suspected. This technique can be useful in the assessment of apophyseal avulsion injuries that frequently occur in children and adolescents. Chronic sequelae of muscle injury may also be seen and diagnosed using radiographs, as in the case of myositis ossificans. Bone scintigraphy can be more sensitive than radiography in detecting early stages of myositis ossificans. However, radiography and bone scintigraphy are limited by their inability to fully characterize soft tissue abnormalities.
Computed tomography (CT) is an important adjunctive procedure in the evaluation and diagnosis of musculoskeletal trauma. It is generally widely available, easy and fast to perform, and capable of providing multiplanar and three-dimensional image reconstructions. CT is superior to radiography in the imaging of complex osseous trauma and does add some further characterization of soft tissues that is far superior to that of radiography. The disadvantages of CT include its use of ionizing radiation and its improved but less than ideal soft tissue representation that is limited in terms of detail and specific lesion characterization when compared with other modalities available, such as ultrasound and magnetic resonance imaging (MRI). However, CT offers its greatest advantage in being able to demonstrates soft tissue calcifications that are frequently overlooked by MRI.
Ultrasound is a very versatile imaging tool that is highly adaptable to clinical practice and assessment of muscle. Traumatic muscle injuries such as complete tendon ruptures or intramuscular hematomas from direct or indirect injuries can be assessed. Generally, ultrasound evaluation of muscle is ideally performed with high-frequency (5 to 10 MHz) linear array transducers. Deeper-seated muscle abnormalities may require the use of a low frequency (2 to 4 MHz) curvilinear probe. The field of view (FOV) can be a limitation for ultrasound in the imaging of relatively larger muscles groups; the introduction of extended FOV imaging helps to improve the accuracy and representation of spatial relationships within muscles. However, with the advent of MRI, exquisite anatomic detail and characterization of soft tissues with a large FOV to incorporate imaging of an entire limb segment has made this modality one of the best suited for evaluation of traumatic muscle injuries, which are frequently soft tissue in nature. MRI is highly sensitive to hemorrhage and edema that may result from muscle injury, also making it the imaging method of choice for muscles, tendons, and ligaments.
TECHNICAL CONSIDERATIONS AND PRINCIPLES OF MRI IN TRAUMATIC MUSCLE INJURIES
Standard MRI Protocol
The goal of imaging is to identify, localize, and characterize abnormalities, which are predominantly soft tissue in nature with respect to traumatic muscle injuries. There are general imaging principles that may be applied with respect to designing a protocol suitable for imaging of muscle. First, a capsule may be placed over the patient’s point of maximal symptoms to help localize the abnormality. Longitudinal (long axis) and orthogonal transverse (short axis) planes should be included in all imaging studies. The longitudinal plane is used to identify an abnormality and determine its length. The orientation of the longitudinal plane varies according to the location of the suspected lesion relative to bone. For lesions anterior or posterior in location, sagittal imaging is favored, whereas coronal imaging is preferred for lesions at the medial or lateral aspects of the limb. The transverse plane aids in additional lesion localization and establishing its anatomic relationship to adjacent structures, such as the myotendinous junction and neurovascular bundle. The FOV should be large enough to allow visualization of the full length of the lesion in question. In the thigh or lower leg, the FOV may be expanded as such to include the asymptomatic side for comparison. This aids in the detection of subtle, asymmetric abnormalities in the symptomatic leg and also allows for assessment of subtle changes in overall muscle bulk that can help to determine the chronicity of the injury.
Once protocol imaging planes are established, pulse sequences are prescribed to characterize tissue according to its T1 and T2 relaxation properties. A combination of pulse sequences may be used, and at least one should employ a fat-suppression technique. For example, when evaluating a suspected muscle abnormality in the thigh, a combination of T1-weighted and inversion recovery (IR) fast spin echo (FSE) sequences can demonstrate most lesions. Fat-suppressed T2-weighted images may also be used to supplement IR pulse sequences. The T1-weighted sequences optimize depiction of fat and normal anatomic planes. Inconspicuous areas of edema, hemorrhage, and intramuscular lesions that cannot be detected on T1-weighted sequences are often better evaluated on IR images. Because of its outstanding sensitivity to edema and subtle abnormalities, the IR or fat-suppressed T2-weighted images are best suited for detecting most pathologic changes. Transverse imaging may employ a combination of T2-weighted and high-resolution fat-suppressed proton density sequences.
Specialized Adjuncts to Routine MRI
Supplemental sequences can be added to a routine protocol as additional tools for further injury or lesion characterization. Gradient-echo images with T2* weighting accentuate certain paramagnetic effects. The most useful application of this technique is increased conspicuity of the blood product hemosiderin, whose paramagnetic properties result in an accentuated “blooming” effect.
Hemosiderin is low in signal on all pulse sequences but is particularly prominent on gradient echo sequences.
These distinctive findings can help to identify and demonstrate myotendinous abnormalities related to remote strain.
Intravenous administration of gadolinium-based contrast material is not routinely necessary as an aid to the diagnosis of muscle injury. In certain circumstances, it can assist in distinguishing between solid and necrotic lesions or may assist in assessing for enhancing components indicating potentially hemorrhagic neoplasm that could masquerade as an intramuscular hematoma. Areas of myonecrosis (muscle infarction), a potential complication of a traumatic muscle injury, could also be detected as areas that do not enhance after intravenous contrast administration.
TRAUMATIC MUSCLE INJURIES: SPECIFIC CONDITIONS
An extensive array and wide spectrum of injuries may be associated with trauma to skeletal muscle. A practical approach to muscle trauma is to categorize injuries in terms of mechanism as direct or indirect ( Box 12-2 ). In direct injuries, as implied, a force is contiguously applied to muscle resulting in immediate trauma to the underlying tissue as may occur with laceration, contusion, or pressure necrosis. Indirect injuries occur through a more complex mechanism of action and dissipation of forces than may be seen with myotendinous strain injuries during forceful eccentric muscle contractions. The remainder of the chapter will focus on various imaging features of the most common traumatic muscle injuries and the potential complications that may arise as a result of such injuries.
DIRECT
Contusion
Laceration
Pressure necrosis
INDIRECT
Exercise (DOMS)
Avulsion: apophyseal or tendinous
Myotendinous strain
Indirect Muscle Injury
Exercise and Delayed-Onset Muscle Soreness
Muscle pain, tenderness, and swelling that develop approximately 24 hours following an unfamiliar or unaccustomed exercise or activity is a common experience for both the elite athlete and the deconditioned “couch potato.” The symptoms, which can range from mild tenderness to functionally limiting pain, are usually referred to as delayed-onset muscle soreness (DOMS). There is no specific instant at which an injury occurs, nor is there generally one particular moment of trauma or acute onset of pain recalled by the patient. Rather, DOMS is a symptom of exercise-induced, indirect muscle damage that typically begins gradually, approximately 8 to 12 hours postexercise. Activities that consist of predominantly eccentric muscle action have the potential to cause this condition. Soreness continues to increase, especially when the affected muscle contracts, stretches, or is palpated, culminating in maximal symptoms at about 2 to 3 days after the activity. Symptoms then slowly dissipate by approximately 1 week. Although the precise mechanism remains uncertain, it is generally thought that DOMS is caused by reversible ultrastructural damage and inflammation mediated through a variety of physiologic factors and is usually self-limited.
DOMS can be commonly seen in muscle groups such as the quadriceps following downhill running or walking activities or the lower back muscles with stressful physical labor. The MRI appearance of DOMS shows diffuse muscle swelling and intramuscular edematous changes as high signal intensity on T2-weighted or IR sequences ( Figure 12-2 ). An important practical fact to keep in mind is that exercise may also cause immediate, transient increases in signal intensity on MRI that may persist up to half an hour after the activity. This effect, which could be confused for pathologic edema or DOMS, is secondary to an increase in extracellular water that accompanies exercise. Fortunately, most individuals are not usually imaged immediately following a workout on the elliptical machine.
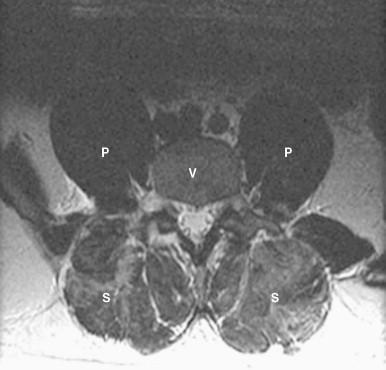
The clinical diagnosis of DOMS is usually not a difficult one, but the diagnostician should be aware of this entity because muscle signal abnormalities on MRI may remain despite resolution of symptoms. In addition, not infrequently, the T2 hyperintense muscle edema pattern can appear similar to findings seen in mild grade 1 myotendinous strain injuries. However, the lack of acute onset of symptoms with DOMS allows for easy clinical differentiation from myotendinous strain injuries.
Injuries of the Myotendinous Unit
Building upon the fundamentals of an understanding of myotendinous anatomic structures and considerations discussed earlier in the chapter, a pathophysiologic approach to the interpretation of imaging of myotendinal unit injuries may be applied. When an eccentric stretching force is applied to skeletal muscle, exceeding the capacity of the system, injury occurs somewhere along the myotendinal unit. A number of risk factors can influence the point at which an injury is likely to occur, and several of these have been alluded to previously in the chapter. For example, age is one important factor to consider. In children and adolescents, the unfused apophysis serves as an attachment site for tendons to the central skeleton. When the apophyseal growth plate is open, it represents the weakest point along the bone-tendon-muscle interface. Therefore apophyseal avulsions are more likely to occur than other myotendinal injuries.
In children, the unfused apophysis is more vulnerable to injury than the musculotendinous junction, explaining the higher incidence of avulsion injuries in this age group. In adults, the tendon is more vulnerable and an avulsion fracture should suggest an underlying bone abnormality such as metastasis.
As the apophysis fuses in young adults, biomechanical failure tends to involve the myotendinous junction at the muscle-tendon interface, which represents the weakest link and site of injury with forceful, eccentric, muscle contractions. In contrast, as the tendon itself becomes diseased, as may be seen in older adults or as a result of overuse or impingement syndromes, failure tends to occur at the level of the degenerated tendon or at its weakened attachment site to bone. It is thus important for radiologists and clinicians to be aware of how these factors influence the pattern and site of injury that occurs along the myotendinal chain of muscle, tendon, and bone.
Apophyseal Avulsion Injuries
Age is an important factor in determining the weakest point along the myotendinous unit. In skeletally immature individuals, the apophyseal growth plate represents the weakest point along the myotendinous unit and is frequently the site of injury. As the force generated by muscle exceeds the attachment of the apophysis to the skeleton, an apophyseal avulsion injury results.
The most frequent sites of apophyseal avulsion injuries occur around the pelvis ( Box 12-3 ). Avulsion of the ischial apophysis by the hamstrings is common in dancers and runners. Other common sites associated with sprinting and kicking sports include the anterosuperior and anteroinferior iliac spines. Patients typically present with loss of function and localized tenderness at the avulsive injury site.
SITE OF TENDON-BONE ATTACHMENT
Anterior superior iliac spine
Anterior inferior iliac spine
Ischial tuberosity
Lesser trochanter of femur
Greater trochanter of femur
MUSCLE
Sartorius
Rectus femoris
Hamstrings (semimembranosus, semitendinosis, biceps femoris)
Iliopsoas
Gluteus medius
Familiarity with the anatomy of the major tendinous attachments sites to bone is indispensable in arriving at the correct diagnosis. In the acute phase, radiography is often diagnostic ( Figure 12-3 ). A displaced apophyseal fracture fragment is usually evident unless the apophysis is not yet ossified or is only minimally displaced. In this instance, MRI may be beneficial. The findings on MRI are along a spectrum depending on the timing and severity of the injury. In minimally displaced avulsive injuries, localized edema with increased T2 hyperintense fluid signal cleft at the apophyseal junction might be the only indication of an injury ( Figure 12-4 ). As the degree of displacement increases, abnormal signal in the muscle and surrounding soft tissues from hematoma and inflammation can be quite striking. Displaced bone fragments can also sometimes be difficult to identify by MRI alone.
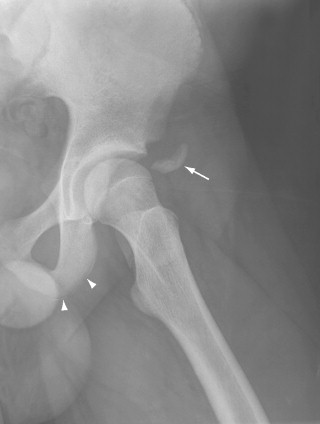
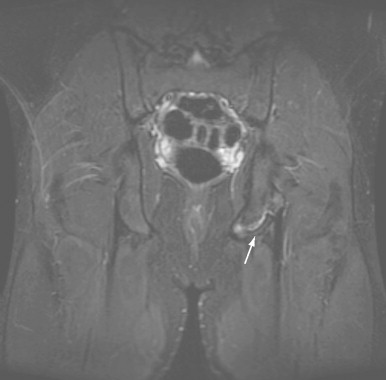
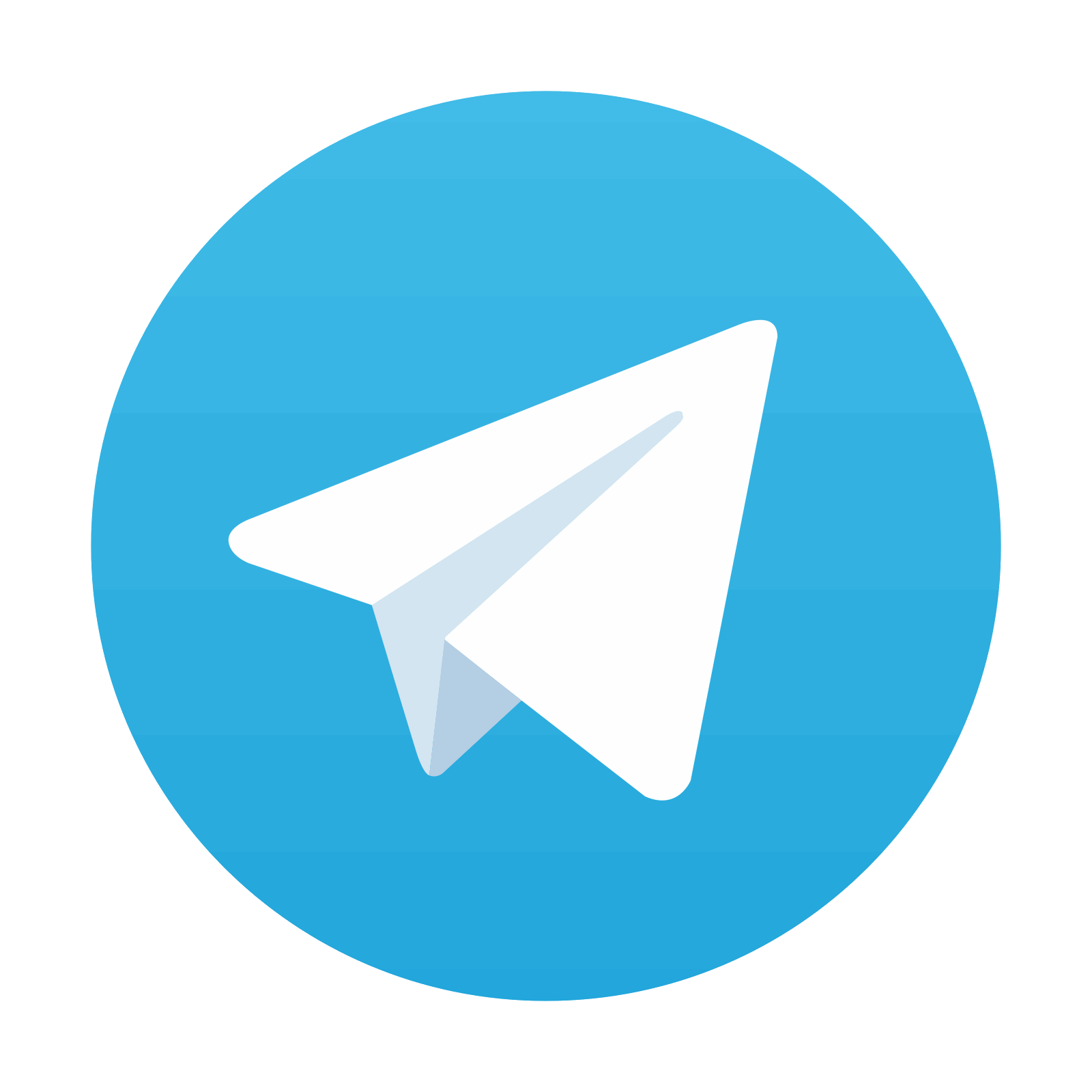
Stay updated, free articles. Join our Telegram channel

Full access? Get Clinical Tree
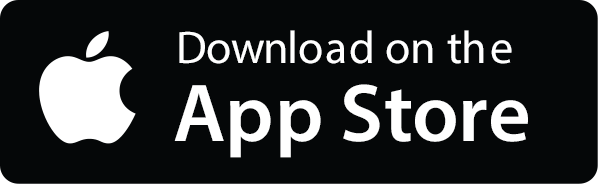
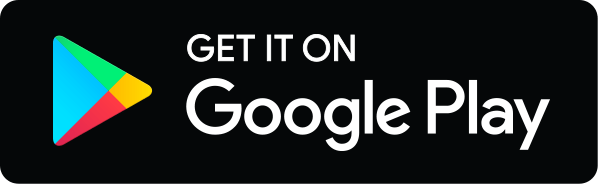
