Fig. 5.1
Frequency and chemical composition of renal stones
There are several conditions that can contribute to the formation of kidney stones [1, 9]. The urine supersaturation is of great importance in the understanding of kidney stones pathophysiology. Since the concentration rather than the amount of the crystallizing solutes is what ultimately establishes stone formation, reduced urinary volume will amplify the saturation of all solutes and raise the risk of all stone formations [1, 9]. When high concentrations of stone-forming substances such as calcium oxalate, calcium phosphate, or struvite are present in the urine, one or more crystals may form and become trapped within the urinary tract. The crystal may attract other crystals and bind together with them, growing into a stone. Another important factor contributing to stone formation is an uneven balance of acid in the urine [1, 9, 10]. The acidity or alkalinity of the urine affects the ability of stone-forming substances to remain dissolved. Some types of stones will only form in acidic urine; others will form only in alkaline urine. Finally, the lack of substances in the urine that prevent the growth of crystals plays an important role. Normally, substances that inhibit the formation and growth of crystals, such as pyrophosphates, citrates, and magnesium, are present in the urine. A decrease or absence of these substances may cause a stone to develop [9, 10].
Renal colic is the most common clinical manifestation associated with kidney stone and is due to the anatomic progression of a stone from the renal pelvis down the ureter, with dramatic relief upon passage of the stone [1, 11, 12]. Stones that are impacted at the ureteropelvic junction produce flank pain, whereas stones lodged in the proximal ureter (between the ureteropelvic junction and the iliac vessels) cause flank pain radiating to the genitals. Stones lodged at the ureterovesical junction produce voiding urgency and suprapubic discomfort, and they cause pain that radiates into the groin and genitals. Associated symptoms include gross or microscopic hematuria, nausea, and vomiting [1, 11, 12].
Nephrolithiasis can also present as a secondary complication, such as an obstruction or a urinary tract infection [1].
Concomitant with the increasing prevalence of urolithiasis is the growing utilization of imaging for diagnosis, treatment planning, and post-treatment follow-up. Imaging in urolithiasis has evolved over the years due to technologic advances and a better understanding of the disease process. While in the past standard radiography and intravenous urography have played a pivotal role in the diagnosis and treatment of patients with acute ureterolithiasis, since its introduction in the early 1990s, unenhanced CT has become the modality of choice in kidney stones imaging [11, 12].
5.2 State of the Art of Conventional (Multi-detector) CT
Unenhanced CT has emerged as the diagnostic imaging modality of choice for the evaluation of suspected renal colic and has proved useful in the pre-therapeutic assessment of urinary tract stones [11–17].
Since the first description of its utility by Smith et al. in 1995 [18], unenhanced CT has been found to have a high degree of sensitivity (95–98 %) and specificity (96–100 %) in the diagnosis of urolithiasis [12, 19–23]. CT has several advantages over other imaging techniques: it can be performed rapidly, does not require the administration of contrast material, is highly sensitive for the detection of stones of all sizes, and allows for the detection of alternative causes of flank pain [12, 19–22]. Appendicitis, diverticulitis, pancreatitis, and gynecologic lesions are often detected by CT in patients with non-specific abdominal pain mimicking ureteral colic when the diagnosis may not be obvious prior to imaging [12]. An additional benefit of CT is its ability to reveal urinary congenital abnormalities, infections, and neoplasms, whose diagnoses have a greater clinical relevance than that of stone disease [12].
The introduction of MDCT in 1998 has opened up new prospects for CT imaging of kidney stones [11, 12]. Thanks to acquisition of isotropic volume and three-dimensional evaluation of these isotropic data sets, radiologists have been empowered to meet the greater expectations of urologists in the assessment of stone disease [12, 19–24]. The identification of the number, size, and location of calculi and determination of the presence of hydronephrosis (i.e., obstruction) are routinely made with MDCT. High-resolution coronally reformatted images generated automatically from isotropic data sets obtained with 16–64-detector CT allow more rapid and accurate detection of urinary stones than axial images alone [12, 19–24].
The scanning technique and parameters for unenhanced multidetector CT for urolithiasis should be tailored toward the indication. It is also important to keep in mind that a CT protocol for the evaluation of stone disease is not considered equivalent to routine unenhanced abdominopelvic CT [12, 19–23]. The former should typically include scanning of the entire urinary tract from the upper pole of the kidneys to the base of the urinary bladder. Although bladder distention has been recommended for enhanced visualization of distal ureteral stones, the improved accuracy of multidetector CT due to the availability of coronally reformatted images usually obviates any patient preparation [12, 19–23].
Thin (1–3 mm) reconstruction sections are recommended for better detection and characterization of urinary calculi, particularly small stones, due to the reduction in partial volume averaging effect. Acquiring CT scans with a section thickness greater than 5 mm can lead to frequent missing of small urinary calculi and can also affect size and attenuation measurements, due to partial volume averaging effect resulting in lower average values [10, 12, 25]. Virtually, all stones are of sufficient attenuation to be revealed on CT including those that are radiolucent on conventional radiographs, such as uric acid, xanthine, and cystine stones [11]. These stones have an attenuation value (>200 HU) greater than that of the surrounding soft tissue, and multidetector CT helps accurately localize a stone within the renal pelvicaliceal system or ureter. The only known exception is a stone that consists entirely of protease inhibitors, such as indinavir sulfate (Crixivan; Merck, Rahway, NJ), used in the treatment for human immunodeficiency virus infection [12, 26].
The most reliable CT sign for ureterolithiasis is a stone within the ureteral lumen, with proximal ureteral dilatation and a normal distal caliber (Fig. 5.2).
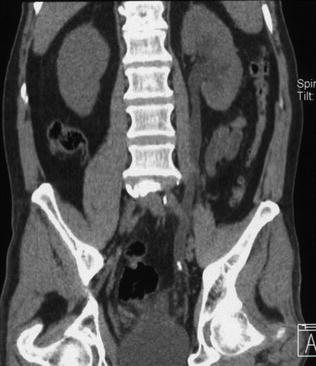
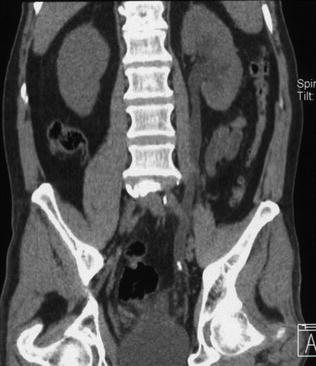
Fig. 5.2
Coronally reformatted unenhanced CT image in a patient with left flank pain permits direct visualization of a small stone located in the lower left ureter with proximal dilatation and normal distal ureteral caliber
In addition to the direct visualization of a stone in the lumen of the ureter, the diagnosis of ureterolithiasis can be confirmed on the basis of several secondary signs by CT as well. The most reliable signs include hydronephrosis, perinephric and periureteral stranding/edema, and unilateral renal enlargement [12]. Ureteral dilatation has a sensitivity of approximately 90 % for use in making a diagnosis of acute ureteral obstruction. Similarly, stranding of the perinephric and periureteral fat has sensitivities of approximately 85 % [12, 27]. In the subgroup of patients with acute flank pain, the presence of both ureteral dilatation and perinephric stranding has a positive predictive value of nearly 100 %, and the absence of both findings has a negative predictive value of approximately 95 % for the diagnosis of acute ureterolithiasis [12, 27].
Less reliable findings include unilateral absence of the white renal pyramid, thickening of the lateroconal fascia, and differences in renal parenchymal attenuation between obstructed and non-obstructed kidneys [12, 27].
One common pitfall in the interpretation of unenhanced CT is the confusion of a phlebolith with a ureteral stone, especially in the pelvis. Therefore, differentiation between stones and other calcific processes is an important issue. Two signs, the “soft tissue rim sign” and the “comet tail sign,” have been described for the differentiation of ureteral stones from these calcifications [12, 27]. The “soft tissue rim sign” consists of a soft tissue attenuation halo around a calcific focus and is very specific for ureteral stones rather than phleboliths [12, 27, 28]. The “soft tissue rim” represents the edematous wall of the ureter around the stone and has a sensitivity of 50–77 % and a specificity of 90–100 % [12, 28]. The presence or absence of the rim is found to correlate with the size of the stone rather than the degree of obstruction [12, 27, 28]. The “comet tail sign” is created by an eccentric, tapering soft tissue area adjacent to the calcification and is a reliable feature in the diagnosis of phleboliths (Fig. 5.3). Another feature that is helpful in differentiation is the central lucent area seen in phleboliths, in contrast to the opacified centers seen in stones [12, 27, 28].
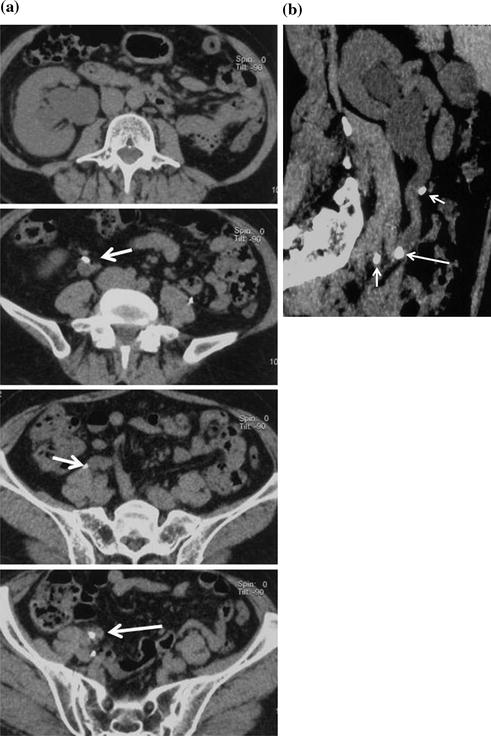
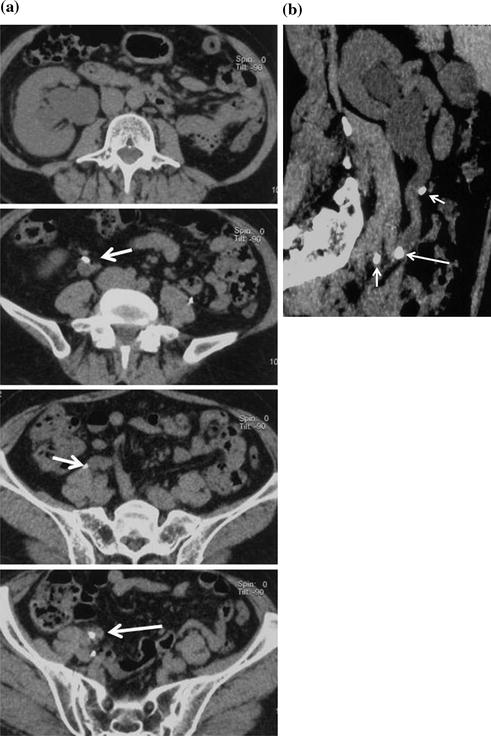
Fig. 5.3
Right ureteral calcolosis with hydronephrosis and multiple phleboliths. Unenhanced axial CT images (a) show right hydronephrosis due to the presence of an iliac ureteral stone. The “soft tissue rim sign” is well appreciated (arrow). Many calcifications close to the ureter are due to phleboliths (short arrows). Parasagittally reformatted CT image (b) easily distinguish the intraureteral stone (arrow) from phleboliths (short arrows)
Once the diagnosis of obstruction by a ureteral stone has been made on CT, prognosis and patient treatment can be guided on the basis of CT findings. Stone burden evaluation at CT is one of the most important factors in determining treatment strategies and management in cases of urolithiasis [12]. Ege et al. [27] reported that stones greater than 6 mm in diameter in the proximal ureter accompanied by more than five secondary signs of obstruction are more likely to necessitate intervention such as endoscopic removal or lithotripsy than those with fewer secondary signs. Novel semiautomatic segmentation tools can also be used to estimate stone volume, which has been shown to be valuable for preoperative planning [12].
Today, multidetector CT is faced with two “burning issues”: the radiation dose to patients and stone composition characterization.
The problem of radiation dose is of particular concern in young individuals who undergo repeated CT examinations due to recurrent stone disease and is consequently likely to be at risk for greater cumulative lifetime exposure [12, 29–33]. Numerous practical approaches for dose reduction in CT for urolithiasis have been advocated in the literature [12, 30–36]. Implementation of strategies targeted at dose reduction at every step of the CT protocol can considerably reduce the radiation dose for each CT examination. One strategy consists of the use of low-dose multidetector CT protocols in which the tube current and tube potential are appropriately lowered [12, 29–36]. Use of very low tube current (50–100 mAs) compared with that used for diagnostic examinations can offer radiation dose reduction of up to 80 % while maintaining diagnostic performance for stone detection [12, 29–36]. An accuracy of 93–97 % has been achieved in the detection of urinary calculi with these low-dose techniques [12, 29–33]. However, the use of these techniques with a fixed tube current is not suitable for patients of different sizes. Rather, the scanning technique must be customized to the patient’s size [12, 37].
Other recently advanced solutions to minimize the radiation dose are automatic tube current modulation technique and iterative image reconstruction algorithms. By modulating noise index and reference milliamperage, it is possible to achieve the objective of radiation dose reduction. However, this technique is problematic in terms of high noise levels, which can cause severe graininess of images and impact accuracy in the diagnosis of small calculi [12, 37]. Iterative image reconstruction algorithm has been recently introduced for MDCT scanners with the goal of reducing image noise [38, 39]. In an iterative reconstruction, a correction loop is introduced into the image generation process [38, 39]. Synthesized projection data are compared to real measurement data in an iterative manner: the update image is refreshed by a correction image, and prior knowledge is imposed on image data. Compared with standard computed filtered back-projection technique, iterative reconstructions permit to acquire CT data at lower radiation dose level while maintaining a higher level of image quality [38, 39].
The other critical issue MDCT has to face with are the knowledge of stone composition, as it is the key determinant of urologic appropriate management [12]. In particular, determination of stone composition is of particular importance because uric acid stones may be treated with urinary alkalinization as a first-line treatment, with surgical treatment being reserved for stones that do not respond to medical therapy, whereas stones of certain compositions (e.g., cystine stones), as well as calcium-based stones of certain attenuation, are extremely difficult to fragment with ESWL [12, 40].
The attenuation values of urinary calculi at conventional 120 kVp, usually fall within certain ranges: uric acid, 200–450 HU; struvite, 600–900 HU; cystine, 600–1100 HU; calcium phosphate, 1200–1600 HU; and calcium oxalate monohydrate and brushite, 1700–2800 HU [12, 41]. Helical CT attenuation as well as stone density can be used to predict stone composition in vitro with 64–81 % accuracy [12]. Although uric acid, cystine, calcium oxalate monohydrate, and brushite calculi have been identified in vitro with an accuracy exceeding 85 % on the basis of attenuation measurements [12, 41], the differentiation between stone types is more complicated and less reliable in vivo than in vitro. Among other factors, it is dependent on the size and accurate placement of the region of interest, it is affected by the section thickness, and MDCT is still unable to fully address this issue.
5.3 Spectral CT: Study Protocols and Clinical Applications
The X-ray attenuation of chemical compositions depends on their density, on their atomic number, and on the energy (X-ray spectrum) of the penetrating beam. The energy dependency of the attenuation is linked to the atomic number; with increasing atomic numbers of the penetrated chemical elements, the energy dependency of the attenuation also increases [42–45]. In urinary calculi, various chemical elements can be found. While calcium oxalate (CaC2O4), calcium phosphate (Ca3(PO4)2), and cystine (C6H12N2O4S2) contain elements with high atomic numbers, uric acid (C5H4N4O3) and struvite ((NH4)MgPO4·6H2O) are mainly composed of elements with lower atomic numbers [42, 43, 46].
The effective atomic numbers of the uric acid stone types fall into a narrow range (approximately 6.84–7.01). The effective atomic numbers of non-uric acid stones are higher and more varied (range 10.78–15.56) [47–50]. The large difference in the effective atomic numbers of uric acid and non-uric acid stones explains the ability of dual-energy CT to differentiate uric acid from non-uric acid stones with high accuracy. However, the differences in the effective atomic numbers between the common non-uric acid stones, such as cystine, struvite, calcium oxalate, brushite, and apatite, are much smaller [51–58]. Spectral-based dual-energy CT analysis became today reliable approach to noninvasively analyze the chemical composition of urinary calculi, by interrogating the attenuation both at high and low energy levels [42–46, 59–61].
Instead of material decomposition, the dual-energy “material labeling” only distinguishes material A from material B, but does not provide the concentration of any material.
For the differentiation between several types of kidney stones, measurements of the effective atomic number or the dual-energy index (DEI) can be used [47, 62]. Measurements of the effective atomic number make use of an additional approximation to the base material decomposition approach. It is assumed that the X-ray attenuation of a material for the relevant energy range is identical to the attenuation of two other materials, that coherent scattering can be neglected, that Compton scattering is independent of the atomic number, and that the photoelectric effect is proportional to the nth power of the atomic number. The measured effective atomic number can be calculated from the true chemical composition of an object if the parameter n, which was used for image calculation, is known. The following equation is adopted to calculate the effective atomic number. The expression is then:
where n i denotes the number density of the atom type i, and Z i denotes its atomic number. For clinical dual-energy CT, the coefficient n is approximately 3. Reliability of the measured effective atomic number decreases for materials with atomic numbers over calcium, which has no limitation in the case of kidney stones.
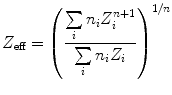
DEI [63], which is closely related to the original CT values x 1 at low kV and x 2 at high kV is calculated using the following equation:
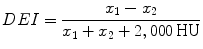
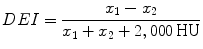
Both the effective atomic number and the DEI are sensitive to partial volume effect, and for small stones (<5 mm), it is potentially difficult to measure the true effective atomic number or the true DEI. The characteristic ratio, on the other hand, is by definition insensitive to this [64].
Quantities which are more stable in the presence of partial volume effects can also be used; as kidney stones are often small and surrounded by soft tissue, the effective atomic number will increase between the apparent surface and the center of a calcified stone. The characteristic ratio can overcome this problem [53, 54].
Kidney stones that are embedded in a certain soft tissue always show CT values at both energies that are between the CT values of the tissue and the CT values of the stone (Fig. 5.4).
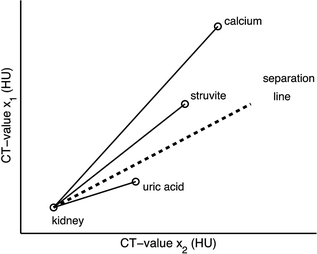
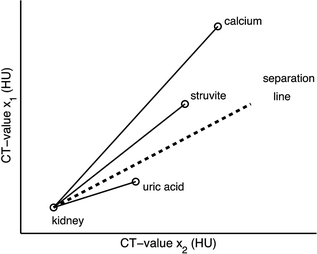
Fig. 5.4
Material labeling for kidney stones: the measured CT values of small, pure stones will be on the connecting lines between kidney and the pure stone material. The slope of the connecting line indicates the stone type
In addition, comprehensive approaches, which use all available information at the same time to distinguish also different types of calcified stones, that have very similar dual-energy properties appear feasible [65].
First results about kidney stone composition characterization have been described using dual-source dual-energy CT platform [53–56, 61] in the differentiation between uric acid and non-uric acid renal stones (Fig. 5.5). Because uric acid calculi comprise approximately 10 % of stone disease and are typically treated medically, using urinary alkalization with stone dissolution, separating this specific subtype of stone provides a potential first management step in a renal stone treatment algorithm [57]. The dual-source dual-energy system offers a rapid characterization tool at the time of initial imaging evaluation, using a three-material decomposition algorithm that assumes every voxel includes a component of water, calcium, and/or uric acid. The voxels are then color coded based on the quantity of each of these components (Syngo Kidney Stone, Siemens AG, Forchheim, Germany). The processing time using this tool is rapid, requiring only few minutes, resulting in display of uric acid stones with a red overlay and non-uric acid with a blue overlay display (Figs. 5.5 and 5.6) [47, 48, 51–58, 66–68].
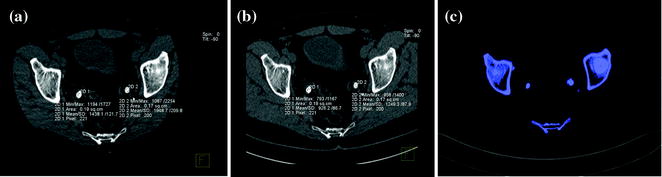
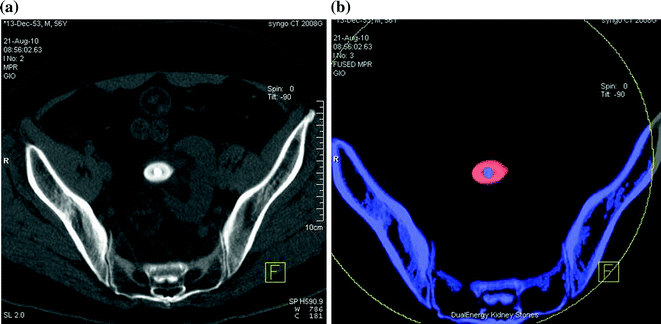
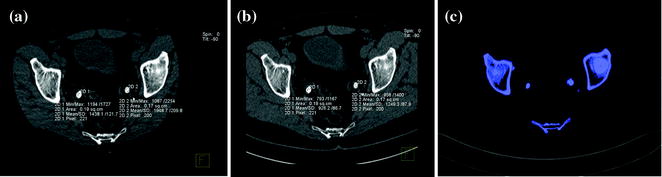
Fig. 5.5
Patient with bilateral pelvic ureteral calculosis. Dual-energy unenhanced axial CT images at 80 kVp (a), at 140 kVp (b), and color-coded image (c) obtained by using Kidney Stone Dual Energy software (Siemens Healthcare). Both stones presented high attenuation value at 80-kVp image (a) compared to the 140 kVp one (b). The 80 and 140 kVp Hounsfield numbers ratio is 1,55 for both stones and the system assigns a blue color indicating a non-uric acid stone composition (c)
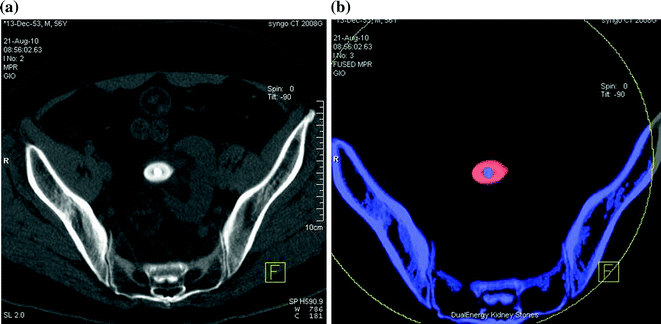
Fig. 5.6
Left ectopic, pre-sacral kidney with an oval stone in the ureteropelvic junction. Low-dose unenhanced single energy (a) and dual-energy color coded (b), obtained by using Kidney Stone software (Siemens Healthcare), axial CT images. Panel b shows that the stone is predominantly composed of uric acid (red color) with a higher attenuation calcific core (blue color)
Differentiation of uric acid from non-uric acid stones was first performed using in vitro models with high sensitivity and accuracy (88–100 %) [57].
In vivo studies for kidney stone characterization also demonstrated high accuracy for differentiating uric acid and non-uric acid stones [51–57]. Separation of uric acid and non-uric acid stones has been performed using a low-dose protocol, with sensitivity of 100 % and specificity of 97 % [42].
Attention has to be paid if stones are closed to ureteral stents. Depending on the chemical composition, stents can be visualized as uric acid or non-uric acid [69, 70], but their high CT value can change the apparent type of nearby stones as mathematical filter algorithms may include them in the analysis of the true stone [70]. The characterization of additional stone types other than uric acid is important to patient’s management, because stones known to be resistant to extracorporeal shock wave lithotripsy, including brushite, cystine, and calcium oxalate monohydrate, can be directed to management by percutaneous or endoscopic stone removal. Moreover, characterization of struvite stones directs the surgeon to total stone removal rather than allowing for small residual fragments that may result in persistent symptoms and recurrent stone growth. Boll and Ziberman [50, 56] demonstrated that a non-commercially available data processing technique with voxel-by-voxel analysis can potentially identify five unique renal stone subtypes both in vitro and in vivo (uric acid, cystine, struvite, calcium oxalate/calcium phosphate, and brushite). Accurate separation of cystine as a unique stone subtype has also been performed in vivo (Fig. 5.7) [57]. However, the ability of dual-energy CT to discriminate between two materials is highly dependent on the difference between each material’s characteristic CT number ratio, which is defined as the ratio of the CT number of a given material in the low-energy image to the CT number of the same material in the high-energy image [48]. The difference between the CT number ratios for any two materials is determined by the separation between the low- and high-energy spectra and the effective atomic numbers of the evaluated materials [48, 49]. The larger spectral separation, the better material discrimination is achieved, especially in the case of non-uric acid stones, which all have similar effective atomic numbers. Spectral separation can be increased by using different filtration on the two X-ray tubes [48, 49]. In the first-generation dual-source scanner (Somatom Definition DS, Siemens Healthcare), both X-ray tubes had the same filtration and the X-ray spectra generated at two different tube potentials have significant overlap [48, 49]. A phantom experiment showed that the use of additional tin filtration on the high-energy X-ray tube of a dual-source CT system operated at 80 and 140 kV can reduce the spectral overlap from 93 to 27 % and significantly improved the separation of non-uric acid stone types by CT number ratio [48]. These data have been further validated by a recent in vivo study which adopted an additional filtration of the high-energy spectrum. This investigation concluded that the use of a tin filter allows for an improved spectral separation between calcium and iodine. [48, 49]. The second-generation dual-source CT system (Somatom Definition Flash, Siemens Healthcare) has a tin filter installed on the high-energy X-ray tube to improve the X-ray spectral separation. This is able to increase the differences in the dual-energy CT number ratio between the different stone types [48, 49]. Using this new dual-source system, an ex vivo study done by Stolzmann et al. [47] showed significant improvement in differentiating uric acid from non-uric acid stones (Fig. 5.8). These experiments showed that dual-energy CT material discrimination was improved, without an increase in radiation dose, compared with single-energy CT techniques. Recently, Qu et al. [49] have demonstrated both in vitro and in vivo that second-generation dual-source dual-energy CT system with tin filtration permits to accurately distinguish up to four different classes of kidney stones, including uric acid stones, cystine stones, struvite/calcium oxalate di- or monohydrate stones, apatite stones, in a patient cohort with a wide range of body habitus by the use of size-adaptive thresholds for each patient [49]. This approach provided an overall accuracy of 79.1 % for stone type characterization [48, 49]. These results are truly promising since the main drawback of first-generation dual-source system operating at 80-/140-kVp settings, is overcome. In fact, the use of a first-generation dual-source system is not recommended for larger patients due to an insufficient photon flux when the tube is operated at 80 kV. This results in excessive image noise if these patients are imaged (Fig. 5.9) [48, 49]. This is an important limitation, for example, for US patient population where the mean body mass index value is usually over 30 kg/m2.