MR imaging is an extremely useful tool in the evaluation of traumatic brain injury in the emergency department. Although CT still plays the dominant role in urgent patient triage, MR imaging’s impact on traumatic brain injury imaging continues to expand. MR imaging has shown superiority to CT for certain traumatic processes, such as diffuse axonal injury, cerebral contusion, and infarction. Magnetic resonance angiography and magnetic resonance venography allow emergent vascular imaging for patients that should avoid ionizing radiation or intravenous contrast.
Key points
- •
MR imaging is commonly performed as an adjunct imaging modality after initial trauma evaluation with CT.
- •
MR imaging is the most sensitive imaging modality for diagnosis of diffuse axonal injury (DAI), parenchymal contusion, and traumatic cerebral infarction.
- •
MR imaging is just as sensitive as CT for the detection of acute subarachnoid hemorrhage (SAH) and is more sensitive in detection of subacute to chronic SAH.
- •
Magnetic resonance angiography (MRA) is a useful tool for interrogating the intracranial vasculature in children, pregnant patients, and patients with renal insufficiency or acute kidney injury.
Introduction
Approximately 1.7 million Americans present to emergency departments (EDs) each year with head trauma. Of these patients, 52,000 die and 275,000 require hospitalization. Traumatic brain injury (TBI) is a contributing factor in one-third of all traumatic deaths in the United States, with motor vehicle accidents comprising the leading cause of TBI-related mortality.
CT traditionally serves as the initial imaging modality of choice in the evaluation of TBI secondary to its widespread availability, speed, cost, and sensitivity for both acute intracranial hemorrhage and fractures. There has been a steady increase, however, in the use of MR imaging by EDs over the past decade. Factors contributing to the increased rate of MR imaging use include improved availability, reduced cost, faster acquisition times, and lack of ionizing radiation. MR imaging also offers improved soft tissue contrast with increased sensitivity for detection for a wide variety of traumatic pathologies, such as DAI and cerebral contusions. This increased sensitivity for TBI is particularly useful in the setting of mild head trauma where initial imaging CT may be negative ( Fig. 1 ).
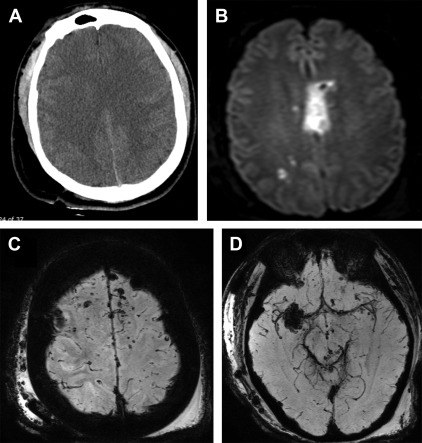
The brain and spine are the most common anatomic locations to be imaged with MR imaging by the ED. The most common indications for emergent MR imaging of the brain are stroke, headache, and trauma. This article provides a succinct review of the MR imaging appearance of the common traumatic intracranial pathologies that are encountered in an ED setting.
Introduction
Approximately 1.7 million Americans present to emergency departments (EDs) each year with head trauma. Of these patients, 52,000 die and 275,000 require hospitalization. Traumatic brain injury (TBI) is a contributing factor in one-third of all traumatic deaths in the United States, with motor vehicle accidents comprising the leading cause of TBI-related mortality.
CT traditionally serves as the initial imaging modality of choice in the evaluation of TBI secondary to its widespread availability, speed, cost, and sensitivity for both acute intracranial hemorrhage and fractures. There has been a steady increase, however, in the use of MR imaging by EDs over the past decade. Factors contributing to the increased rate of MR imaging use include improved availability, reduced cost, faster acquisition times, and lack of ionizing radiation. MR imaging also offers improved soft tissue contrast with increased sensitivity for detection for a wide variety of traumatic pathologies, such as DAI and cerebral contusions. This increased sensitivity for TBI is particularly useful in the setting of mild head trauma where initial imaging CT may be negative ( Fig. 1 ).
The brain and spine are the most common anatomic locations to be imaged with MR imaging by the ED. The most common indications for emergent MR imaging of the brain are stroke, headache, and trauma. This article provides a succinct review of the MR imaging appearance of the common traumatic intracranial pathologies that are encountered in an ED setting.
Intra-axial trauma
Contusions
Cerebral contusions are parenchymal bruises that occur when the brain has an impact on the surface of the skull, falx cerebri, or tentorium. Blunt, closed head injury is the most frequent cause of brain contusions. The inferior frontal and anterior temporal lobes are the most common locations for cerebral contusion because the adjacent anterior and middle cranial fossae of the skull base have a relatively sharp, irregular contour. The occipital lobes are almost always spared from contusion because the bordering occipital bone exhibits a smooth inner table. A contusion at the site of trauma is referred to as a coup contusion . When the contusion is 180° opposite the site of direct impact, the contusion is termed, contrecoup ( Fig. 2 ). Contusion may be difficult to visualize on initial CT examination in an ED. Contusions, however, have a tendency to enlarge and become more apparent on follow-up imaging.
Contusion by definition must involve the cortical surface of the brain, a feature that helps differentiate from subcortical DAI. The imaging appearance of cerebral contusion may vary from subtle petechial hemorrhage to multiple large parenchymal hematomas with severe mass effect. A CT examination classically demonstrates foci of hyperattenuating hemorrhage within a wedge-shaped region of hypodense cerebral edema. MR imaging is more sensitive than CT for the detection of cerebral contusion. The fluid-attenuated inversion recovery (FLAIR) sequence is superior to T1-weighted and T2-weighted sequences for detection of cerebral edema in the setting of a brain contusion ( Fig. 3 ). The appearance of hemorrhagic contusions change on T1-weighted and T2-weighted sequences along a predictable pattern based on the age of blood products ( Table 1 ). Hemorrhagic contusions often contain blood products in various stages of degradation within the hematoma, with the evolution progressing in a centripetal fashion. Subacute blood products are one of only a few pathologic processes that are hyperintense on T1-weighted sequences.
Time | Blood Product | T1 | T2 | T2* | |
---|---|---|---|---|---|
Hyperacute | <24 h | Oxy-Hgb | Iso | Bright | Dark rim |
Acute | 1–3 d | Deoxy-Hgb | Iso | Dark | Dark |
Early subacute | 3 d to 1 wk | Intracellular met-Hgb | Bright | Dark | Very dark |
Late subacute | >1 wk | Extracellular met-Hgb | Bright | Bright | Variable signal intensity |
Chronic | Months | Hemosiderin | Dark | Dark | Very Dark |
Gradient-recalled echo (GRE) and susceptibility-weighted images (SWIs) are the most sensitive sequences for detection of hemorrhagic contusion. Hemorrhage appears as black “blooming” artifact on these sequences. There is growing evidence in the literature confirming SWI’s superiority to GRE for the detection of intracranial hemorrhage. Foci of DWI hyperintensity within a cerebral contusion may reflect either blood products or regions of cytotoxic edema and neuronal death.
Penetrating Injury
Penetrating injuries are characterized by a projectile piercing the skull, dura, and brain. The projectile leaves a trail of destruction with damaged brain parenchyma and torn vasculature. When the cerebral defect is linear in morphology, the term, cerebral laceration , is often used ( Fig. 4 ). Gunshot and stabbing assaults are the most common sources of penetrating injury.
CT imaging can show metallic density ballistic fragments, comminuted skull fractures, macerated brain parenchyma, cerebral contusion, and liner lacerations. MR imaging is not often performed in the ED on patients with penetrating cerebral injuries to avoid the potential harm that may be caused by displacement of intracranial metallic bullet fragments and the high rate of patient mortality shortly after admission. When performed, MR imaging can further characterize the primary penetrating injury as well as detect superimposed DAI and cerebral infarctions.
Diffuse Axonal Injury
Severe rotational acceleration/deceleration stress on the brain results in DAI. The cerebral gray matter and white matter have different density and stiffness and, therefore, accelerate and decelerate at different speeds. This differential movement results in stretching or shearing injury centered at the junction between the cerebral gray and white matter. Motor vehicle collisions are the most common causes of DAI. DAI has a strong predilection for the gray-white junction of the frontotemporal lobes, corpus callosum, brainstem, and internal capsule. Adams and colleagues introduced a staging system for DAI in 1989. The stage of DAI is determined by lesion location and helps predict patient prognosis ( Table 2 ).
Grade | Anatomic Region of Injury |
---|---|
1 | Gray-white matter junction (most common in frontal and temporal lobes) |
2 | Grade 1 with additional lesions in corpus callosum (most common in splenium) |
3 | Grade 2 with additional lesions in brainstem |
There is often a significant discordance between the dismal clinical appearance of the DAI patient and the surprisingly normal-appearing head CT. Noncontrast head CT performed in an ED is often normal in the setting of mild to moderate DAI. A majority (80%) of DAI lesions are nonhemorrhagic and these foci are particularly difficult to diagnose with CT. CT may demonstrate a few punctate, hyperattenuating foci of hemorrhage. These foci represent the tip of the iceberg, with additional nonvisualized brain injury highly likely. CT in an ED also allows fast triage and exclusion of concurrent injuries, such as skull fractures, cerebral herniations, contusions, and parenchymal hematomas.
MR imaging has a much higher sensitivity than CT for DAI, particularly for the more common nonhemorrhagic variety. Paterakis and colleagues examined more than 1000 trauma cases over a 2-year period. They isolated 33 patients who had normal admission head CT examinations but clinical evidence of TBI. Follow-up MR imaging performed with 48 hours confirmed DAI in 24 of the 33 patients (73%). MR imaging shows small, ovoid foci of restricted diffusion and T2 hyperintensity in the setting of acute DAI ( Figs. 5 and 6 ). The long axis of the ovoid DAI lesion is aligned in the direction of the regional white matter fibers. In the setting of hemorrhagic DAI, MR imaging demonstrates oval foci of gradient susceptibility.
It is imperative that a trauma MR imaging protocol includes a heme-sensitive sequence. Spitz and colleagues have shown SWI more sensitive than FLAIR imaging for detection of lesions in the setting of hemorrhagic DAI. SWI is more sensitive than traditional T2* GRE imaging for the detection of intracranial blood products in a variety of pathologic conditions. Beauchamp and colleagues reported that SWI can reveal up to 30% more lesions than CT or conventional MR imaging in the setting of TBI. In another study, Tong and colleagues concluded that SWI depicts significantly more hemorrhagic DAI lesions than does conventional T2* GRE imaging.
Traumatic Cranial Nerve Injury
Cranial nerve injury is not uncommon in the setting of head trauma. In general, cranial nerves I through VII are most frequently injured. Blunt trauma is associated with injury of the olfactory, facial, and vestibulocochlear nerves. Penetrating orbital trauma or fractures through the optic canal may result in optic nerve injury. The oculomotor, trochlear, and abducens nerves are commonly injured or even avulsed in the setting of trauma ( Fig. 7 ). Transverse temporal bone fractures have a high incidence of facial nerve injury at the level of the geniculate ganglion. MR imaging is the modality of choice in evaluating the cranial nerves and may reveal irregularity or discontinuity of the nerve fibers. Dedicated high-resolution, thin MR imaging sequences are required for interrogation of the cranial nerves. Heavily T2-weighted 3-D sequences, such as constructive interference in steady state (CISS) and fast imaging employing steady-state acquisition (FIESTA), are typically selected because they provide the needed special resolution to delineate these small structures. Hemorrhage and edema may be evident on routine MR imaging sequences at the root entry zone of the brainstem.
Extra-axial trauma
Subarachnoid Hemorrhage
Bleeding into the space between the arachnoid membrane and the pia results in SAH. Trauma is the most common cause of SAH. Additional nontraumatic causes of SAH include aneurysm rupture, arteriovenous malformation, vasculopathy, and coagulopathy, among other entities. SAH is exceedingly common in the setting of moderate to severe head injury and is associated with both blunt and penetrating head trauma. SAH can often be found in isolation or adjacent to subdural hematomas (SDHs) and parenchymal contusions.
On CT, acute SAH manifests as curvilinear hyperattenuation that insinuates along the surface of the brain parenchyma and fills the cerebral sulci, slyvian fissures, and basilar cisterns. MR imaging has been shown to be just as good as CT in detection of acute SAH and superior to CT in detection of subacute to chronic SAH. Verma and colleagues examined 25 consecutive patients that presented to the ED with acute SAH. They found that the SWI and FLAIR MR imaging sequences yielded a distinctly higher detection rate for SAH than CT. The SWI and FLAIR sequences played complementary roles in SAH detection ( Figs. 8 and 9 ). SWI was superior for detection of central SAH (interheispheric and intraventricular) and FLAIR was superior for detection of peripheral SAH over the convexities. The high sensitivity of FLAIR for SAH is confounded by a high false-positive rate. Cerebrospinal fluid (CSF) pulsation can result in flow-related artifacts in the basilar cisterns that may be confused for SAH on the FLAIR sequences ( Fig. 10 ). FLAIR hyperintensity in this anatomic region should alert the radiologist to examine the CT or additional MR imaging sequences (SWI, T2*, and T1) to exclude SAH. FLAIR hyperintensity in the cerebral sulci is also not specific for SAH. The differential diagnosis includes meningitis ( Fig. 11 ), leptomeningeal carcinomatosis, melanosis, artifact from poor CSF suppression ( Fig. 12 ), and hyperoxygenation in the setting of anesthesia.
