Respiration has been shown to be an important factor influencing the quality of cardiovascular magnetic resonance (CMR) images. In addition to cardiac motion, which can be addressed reasonably well by electrocardiographic (ECG) triggering, respiratory motion moves the position and distorts the shape of the heart by several millimeters between inspiration and expiration. In 1991, Atkinson and Edelman showed the detrimental effects of breathing on the quality of cardiac studies by showing improved detail (fast low angle shot) in breath-hold segmented fast gradient echo images compared with conventional non–breath-hold images. Although breath-holding produces images that are free of respiratory motion artifact, it is not without problems. The breath-hold position may vary from one breath-hold scan to the next, giving rise to misregistration effects, and it may also vary during the breath-hold period itself, resulting in image blurring and artifacts. In addition, the scan parameters are limited by the need to perform imaging within the duration of a comfortable breath-hold period, and for a number of patients, this period may be very short.
An alternative to breath-holding is to monitor respiratory motion throughout the data acquisition period and to correct the data for that motion, either in real time or through postprocessing, with the efficacy of both techniques being strongly dependent on the accuracy of the method of motion assessment. During normal tidal respiration, the superior-inferior (SI) motion of the diaphragm is approximately four to five times the anterior-posterior motion of the chest wall, and consequently, diaphragm motion is the most sensitive measure of respiratory motion. In 1989, Ehman and Felmlee were the first to introduce navigator echoes for measuring the displacement of a moving structure and to demonstrate their use in determining diaphragm motion during respiration. The navigator echo is the signal from a column of material oriented perpendicular to the direction of the motion to be monitored. On Fourier transformation, this signal results in a well-defined edge of the moving structure. The navigator echoes may be interleaved with the imaging sequence and, consequently, enable the motion to be determined throughout the data acquisition period.
In CMR, there have been a number of developments in the use of navigator measurement to reduce the problems of respiratory motion. This chapter discusses these developments, considers the various choices that have been studied in the implementation of navigators, and discusses their importance. There are many variables in the application and use of navigator echoes, and although there have been some attempts to study these, it is unlikely that we are close to optimizing their application.
Use of Navigator Information
There are two distinct ways of using navigator echoes to reduce the problems of respiratory motion in CMR, which are multiple breath-holding with feedback and free-breathing methods. The first of these uses the navigator information to provide visual feedback on the diaphragm position to subjects to allow them to hold their breath at the same point repeatedly. The second uses the navigator echo measurement as an input to some form of respiratory gating algorithm while the patient breathes normally. Fig. 7.1 shows actual respiratory trace data in a subject when performing multiple breath-holds and when free breathing. In both cases, a navigator acceptance window, typically 5-mm wide, is defined, and all data acquired when the navigator is outside of this window are ignored. The resulting image therefore consists of data acquired over a narrow range of respiratory positions. The respiratory or scan efficiency is defined as the percentage of ECG triggers that fall within the navigator acceptance window and is a measure of the data rejection rate, which in turn determines the overall scan duration. As the navigator acceptance window is reduced, the rejection rate increases and the scan efficiency decreases. Fig. 7.2 shows the residual diaphragm displacements that occurred during data acquisitions performed during conventional breath-holding, breath-holding with navigator feedback, and navigator free-breathing in normal subjects. Both navigator techniques result in images acquired over a reduced range of diaphragm displacement compared with those acquired using repeated conventional breath-holding. In addition, they allow a longer overall scan time. This allows for averaging of data to improve the signal-to-noise ratio, increasing the k -space coverage for improved spatial resolution, and increasing the temporal resolution by reducing the number of individual image views acquired per cardiac cycle.
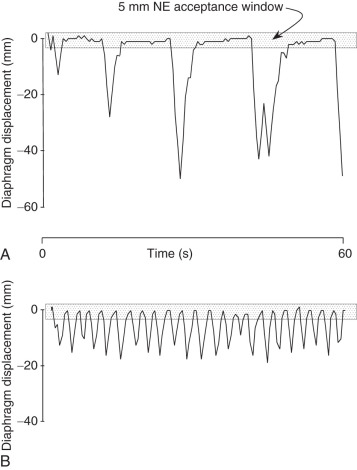
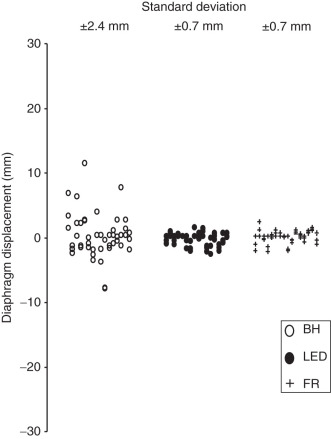
Multiple Breath-Hold Methods
Wang and colleagues were the first to show the use of a respiratory feedback monitor to reduce misregistration artifacts in consecutive breath-hold segmented fast gradient echo coronary artery images and to show improved image quality from averaging scans acquired over multiple breath-holds. When used in informed healthy volunteers, this technique has been shown to produce good results with reasonable scan efficiency. However, a period of training is required, and the process can be problematic, particularly with patients who have difficulty holding their breath because of a combination of illness and anxiety. Although it might be expected that breath-holding with respiratory feedback would enable the completion of a cardiac study much more quickly than when using the free-breathing methods described later, because of the time required for training and the required rest periods between breath-holds, the overall examination times are longer than anticipated. In fact, in a group of patients with coronary artery disease, there was no significant difference between the overall examination times with the two techniques, although the same study showed that, in a group of normal healthy subjects, multiple breath-holding resulted in a time reduction of 20%.
Free-Breathing Methods
Free-breathing methods require very little cooperation from the patient. The main disadvantage is the potential for respiratory drift, which can cause considerably reduced scan efficiency. Recently, therefore, most effort has gone toward improving scan efficiency with this approach.
Much of the early work used retrospective respiratory gating. With this method, data acquisition was oversampled, typically by a factor of five, and then sorted retrospectively so that the final image was constructed from data acquired over the narrowest possible range of respiratory positions. In 1995, Hofman and associates showed that, using this approach, the image quality of three-dimensional (3D) coronary acquisitions was improved over those acquired with multiple averages. However, scan efficiency was poor (20% for an oversampling ratio of five), and although the final image was constructed from the narrowest respiratory window possible, the range was highly dependent on the subject’s breathing pattern during the long acquisition period and was often still unacceptably high.
After the introduction of prospective control techniques, navigators have most commonly been used with a simple accept-reject algorithm where data are acquired or not, depending on whether the navigator measurement is within a predefined acceptance window. Oshinski and coworkers were the first investigators to show high-quality coronary artery images with such an approach. The problem with this method, however, is that for reasonably high scan quality, a narrow acceptance window of 5 mm or less is required, and this generally results in relatively poor scan efficiency. In addition, as noted earlier, many subjects and patients undergo a drift in diaphragm position over time, such that the predefined acceptance window becomes less and less suitable as the study progresses. The diminishing variance algorithm overcomes this problem because it does not use a predefined acceptance window. With this method, one complete scan is acquired and the navigator positions are saved for each line of data. At the end of the initial scan, the most frequent diaphragm position during that scan is determined, and a process of reacquiring lines of data that were acquired with diaphragm positions furthest offset from this position begins. As time progresses, the range of diaphragm positions for the data making up the final set is considerably reduced. In addition to the lack of requirement of an acceptance window, this method has the advantage that an image can be reconstructed at any time after the initial dataset is complete.
Another alternative to the simple accept-reject algorithm that can improve both image quality and scan efficiency is to use a k -space ordering that depends on diaphragm position. Two similar approaches have been suggested, based on the finding that the center of k -space appears to be more sensitive to motion than the edges. Jhooti and colleagues developed a phase-encode ordered method that used a dual acceptance window of 5 mm for the center of k -space and 10 mm for the outer regions. This approach allowed much greater scan efficiency than other methods, while retaining scan quality ( Fig. 7.3 and Table 7.1 ). An alternative method, initially developed by Sinkus and Bornert to address general respiratory motion and more recently applied to imaging of the coronary arteries, used a tailored acceptance window through k -space as opposed to phase-encode ordering to obtain a very similar result. Both of these phase-ordering or windowing techniques use a predefined navigator acceptance window, and scan efficiency is reduced when the respiratory pattern changes during study acquisition. This has been more recently addressed by Jhooti and colleagues, who developed a technique combining the benefits of phase ordering with an automatic window selection that enables the highly efficient acquisition of high-quality coronary artery images without the need for a predefined acceptance window. 3D motion-adapted gating is a similar technique that yields images comparable with standard prospective navigator gating, with significantly improved scan efficiency. A further development of these ideas, termed continuously adaptive windowing strategy (CLAWS), was described by Jhooti et al. in 2010. This novel and dynamic acquisition strategy ensured all potential navigator acceptance windows are possible and acquires an image with the highest possible efficiency regardless of variations in the respiratory pattern. Unnecessary prolongation of scan durations due to respiratory drift or navigator acceptance window adjustments are avoided. Because CLAWS requires no setting of the acceptance window, nor monitoring of the navigator traces during the scan, operator dependence is minimized and ease of use improved. This method has been applied by Keegan et al. to improve the respiratory efficiency of 3D late gadolinium enhancement imaging and by Jhooti et al., who combined it with biofeedback to increase the navigator efficiency for imaging the thoracic aorta.
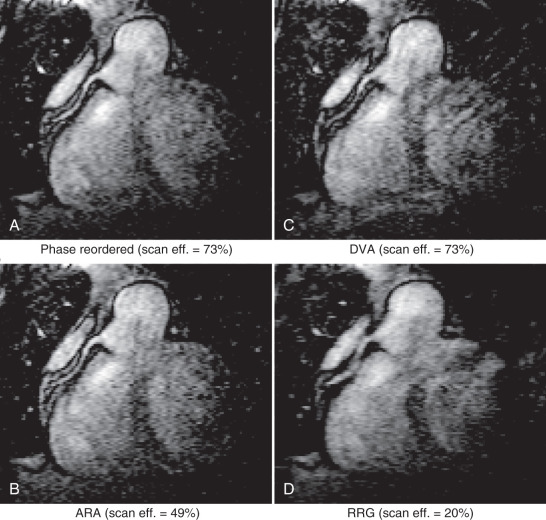
Phase Ordered | ARA | DVA a | RRG | |
---|---|---|---|---|
Image quality mean score | 4.4 | 4.7 | 6.6 | 6.8 |
Scan efficiency | 72 (±11.6) | 48 (±11.5) | 72 (±11.6) | 20 |
a Scan time for the DVA technique is set to that of the phase-ordered technique.
b Mean image quality scores (1 = excellent, 10 = very poor) and scan efficiencies (± standard deviation) for data acquired using phase ordering, an ARA, the DVA, and RRG in 15 subjects.
Navigator Echo Implementation
Method of Column Selection
Two methods have been used for the generation of a navigator echo.
With the spin echo technique, a spin echo signal is generated from the column of material formed by the intersection of two planes, one excited by a 90-degree radiofrequency (RF) pulse and the other by a 180-degree RF pulse. The column cross section may be either rectangular or rhombic, depending on the orientation of the two planes. This approach is very robust and produces an extremely well-defined column. However, it cannot be repeated rapidly, and care must be taken to ensure that the column selection planes do not impinge on the region of interest.
The alternative approach is to use a selective two-dimensional (2D) RF pulse to excite a column of approximately circular cross section. Although this technique is much more sensitive to factors such as shimming errors, which can potentially cause blurring and distortion of the column, with a reduced flip angle, it can be repeated more rapidly and the navigator artifact is less extensive.
Both methods are used routinely for research studies on coronary imaging, without any reported problems.
Correction Factors
In CMR, navigator echoes are most frequently used to measure the position of the diaphragm. However, the motion of the heart is not straightforward, and only the inferior border that sits on the diaphragm will move to the same extent, whereas superiorly, the relative motion will be reduced. This was first studied by Wang and associates, who measured the displacement of the right coronary artery root, the origin of the left anterior descending artery, and the superior and inferior margins of the heart relative to the diaphragm in 10 healthy subjects. For the right coronary artery origin, the mean (± standard deviation) relative displacement (or correction factor [CF]) was 0.57 (± 0.26). McConnell and coworkers first used this CF to track the position of the imaging slice during breath-holding and showed improved image registration relative to untracked acquisitions. In free-breathing studies, the CF was first applied by Danias and colleagues, who showed that tracked image quality was maintained as the navigator acceptance window increased from 3 mm to 7 mm, whereas in untracked images, it decreased significantly. This technique, called real-time prospective slice following, or slice tracking, is now used routinely for both 2D and 3D methods of acquisition. Of note, however, is the relatively high standard deviation of the CF discussed earlier, which reflects considerable intersubject variation in the degree of cardiac motion with respiration. This was also observed by Danias and coworkers, who used real-time 2D echo planar imaging to study the SI motion of the heart as a function of navigator position. The accuracy of slice-following techniques will obviously depend on the accuracy of the CF implemented. In 1997, Taylor and colleagues showed how a subject-specific factor could be measured rapidly with end-inspiratory and end-expiratory breath-hold scans before the coronary imaging protocol. Fig. 7.4 shows the relationship between the motion of the right hemidiaphragm and the coronary ostia measured in one subject, with the slope of the graph giving the CF. Fig. 7.5 shows two examples of subjects with very different CFs, showing how a wider acceptance window can be used, thus improving scan efficiency. The need for a subject-specific CF has further been confirmed in 3D coronary angiography, where its use was found to yield optimal image quality. In 2002, Keegan and associates further developed this area of work by studying the variability of CFs in the SI, anterior-posterior, and right-left directions for both breath-holding and free-breathing. The study concluded that subject variability in CFs, together with within-subject differences between breath-holding and free-breathing, is such that slice following should be performed with subject-specific factors determined from free-breathing acquisitions.
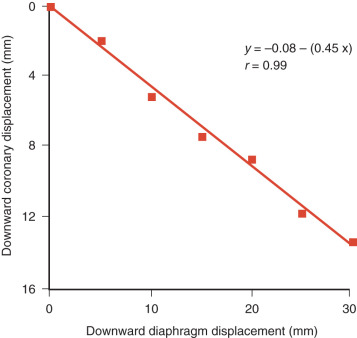
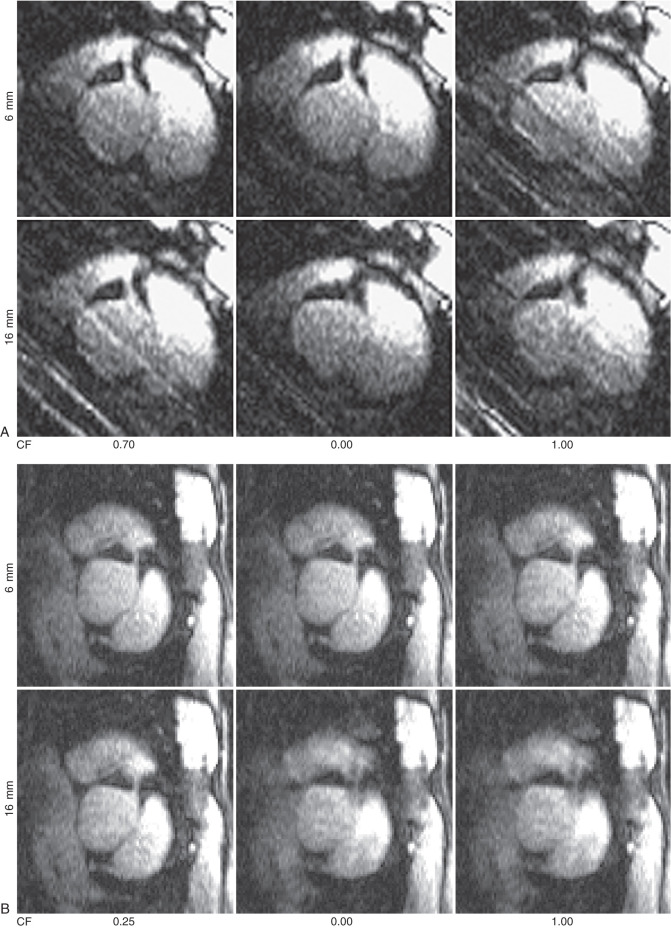
An additional or alternative approach to the real-time slice following described earlier is to use a postprocessing adaptive motion correction technique to correct an image retrospectively for movement occurring during data acquisition. This technique, which can be used to correct a 2D acquisition for in-plane displacement or a 3D acquisition for in-plane and through-plane displacement, may not appear to be an attractive option initially, but it has the advantages of allowing the CF to be optimized for each individual patient and provides an alternative approach to those centers with scanners that do not have a real-time decision-making capability. This approach has been implemented with both segmented gradient echo and interleaved spiral coronary artery acquisitions, with promising results.
Column Positioning
The degree of diaphragm motion detected by the navigator echo is dependent on the positioning of the navigator column. The dome of the right hemidiaphragm is higher than that of the left, and the two move coherently with respiration, but to differing degrees. Motion of the diaphragm is also greater posteriorly than anteriorly (anterior and dome excursions are 56% and 79%, respectively, of posterior excursions), and at the level of the dome, it is greater laterally than medially. The CF implemented in real-time slice following or postprocessing adaptive motion correction, as described earlier, is strongly dependent on the positioning of the navigator column and further supports the use of a subject-specific factor, as described in the previous section.
McConnell and colleagues studied the effects of varying the navigator location on the image quality of coronary artery studies. Navigators were positioned through the dome of the right hemidiaphragm, through the posterior portion of the left hemidiaphragm, through the anterior and posterior left ventricular walls, and through the anterior left ventricular wall, as shown in Fig. 7.6 . The advantage of the latter navigator position is that it would eliminate the need for a CF, as described in the previous section, relating the navigator-echo-measured displacement to the coronary artery motion. The results are summarized in Table 7.2 and show no significant differences in the image quality scores obtained with varying navigator location. There was a tendency for the anterior left ventricular wall navigator scans to be longer in duration, but the difference did not reach statistical significance. One of the problems with monitoring the heart itself is the complex anatomy, making it more difficult to find a suitable position for the navigator column. More sophisticated methods of positioning the column may further improve this method of monitoring cardiac motion.
