Functional neuroimaging provides means to understand the relationship between brain structure and associated functions. Functional MR (fMR) imaging can offer a unique insight into preoperative planning for central nervous system (CNS) neoplasms by identifying areas of the brain effected or spared by the neoplasm. BOLD (blood-oxygen-level–dependent) fMR imaging can be reliably used to map eloquent cortex presurgically and is sufficiently accurate for neurosurgical planning. In patients with brain tumors undergoing neurosurgical intervention, fMR imaging can decrease postoperative morbidity. This article discusses the applications, significance, and interpretation of BOLD fMR imaging, and its applications in presurgical planning for CNS neoplasms.
Key points
- •
Functional MR imaging provides reliable in vivo assessment of the eloquent cortex and can be used to identify sensorimotor, language, and visual regions.
- •
Key limitations of BOLD task-fMR imaging include:
- ○
Necessity of patient cooperation and ability of patients to perform the required task, thus limiting its application in young and elderly patients as well as those with neurocognitive limitations.
- ○
BOLD fMR imaging is motion sensitive.
- ○
In instances where tumor involves the eloquent cortex, postoperative changes limit BOLD fMR imaging assessment of perisurgical sites.
- ○
Tumor and tumor microenvironment can affect normal hemodynamic response, resulting in neurovascular uncoupling and leading to false-negative BOLD fMR imaging signal changes.
- ○
Introduction
Functional neuroimaging provides a means to understand the relationship between brain structure and function. Functional MR (fMR) imaging can offer unique insight into preoperative planning for central nervous system (CNS) neoplasms by identifying areas of the brain affected or spared by the neoplasm. The development of fMR imaging presented a breakthrough in imaging acquisition and analysis as well as patient management. Since its discovery in 1992 by Ogawa and colleagues, the blood-oxygen-level–dependent (BOLD) fMR imaging technique has become the dominant in vivo imaging technique for functional brain imaging. BOLD fMR imaging provides functional information without requiring invasive electrodes, radiation, or intravenous contrast agent. The BOLD sequence uses differences in tissue magnetic susceptibility properties (T2∗ effect) between oxyhemoglobin (diamagnetic) and deoxyhemoglobin (paramagnetic). The BOLD fMR imaging signal depends on cerebral blood flow, cerebral blood volume, and cerebral metabolic rate. The net difference between tissue oxyhemoglobin and deoxyhemoglobin during the hemodynamic response (known as the hemodynamic response function [HRF]) is what generates the MR imaging signal. The “BOLD effect” assesses coupling of oxygenated blood flow and neuronal metabolism during functional tasks, resulting in a net difference in oxyhemoglobin and deoxyhemoglobin, which generates the BOLD signal. Hemoglobin’s magnetic properties depend on the reduction-oxidation of iron between Fe 2+ and Fe 3+ states (ie, oxygenated and deoxygenated states). These changes result in an increase in local tissue-derived signal intensity on T2∗-weighted MR images. BOLD fMR imaging provides good spatial resolution for effective mapping of CNS function in patients whose tumor and/or peritumoral edema is adjacent to eloquent cortex.
This article discusses the applications, significance, and interpretation of BOLD fMR imaging and its relevance to presurgical planning in patients with CNS neoplasms.
Blood-oxygen-level–dependent functional MR imaging and the eloquent cortex
Sensorimotor
At many medical centers in the United States, BOLD fMR imaging is used to evaluate the sensorimotor system by providing an effective, low-risk, noninvasive means of evaluating the eloquent cortex, comparable with intraoperative mapping techniques. BOLD fMR imaging can be used to identify critical areas of interest to the neurosurgeon by discerning key functional areas of gray matter on structural MR imaging. This is particularly important in settings where tumor and/or peritumoral edema is in close proximity to eloquent cortex. The primary motor cortex, located in the precentral gyrus, is responsible for generating neural impulses that control motor movement. Any significant injury to this region can result in irreversible paresis. The primary sensory cortex is located in the postcentral gyrus. Separated by the central sulcus, the motor and sensory gyri are somatotopically organized.
In the pre-BOLD fMR imaging era and in instances where BOLD fMR imaging is not available, traditional anatomic landmark approaches are used to identify the precentral gyrus and intraoperative electrodes are used to map out the hand-foot motor regions. Traditionally, the “reverse omega sign” is used to identify the hand motor region; however, this is not always reliable to because of anatomic variation and/or distortion of the homunculus by neoplasm or edema. Motor functions activated by the primary somatosensory cortex, such as the planning, execution, and control of specific behavior, is a complex neural process, and delineating neuronal function solely according to anatomic landmarks can be unreliable. In addition, the lack of reliable anatomic landmarks make it difficult to precisely localize the facial motor region on the precentral gyrus. Supported by clinical and neural data, 3 main motor areas (hand, face/lips/tongue, and foot) can be reliably identified on fMR imaging with good agreement between BOLD fMR imaging maps and intraoperative functional mapping. The foot motor region is usually located medially along the parasagittal aspect of the precentral gyrus at the level of the interhemispheric fissure ( Figs. 1 and 2 ). The direct intraoperative cortical stimulation of this region is complicated by the presence of the adjacent superior sagittal sinus and can be further complicated by the presence of nearby edema, tumor, and/or aberrant vasculature such as a developmental venous anomaly. The 3 main functional areas (face, hand, foot) span the precentral gyrus (lateral to medial) and can be reliably assessed on task-based fMR imaging. It is important that patients with paresis can elicit motor and sensory activation with sensory stimulation of the hand, face, or foot through induced motor signals. During an fMR imaging examination, studies have shown paretic patients to induce more head motion as they experience difficulty moving the affected limb, leading to motion artifact and misregistration of BOLD signal.
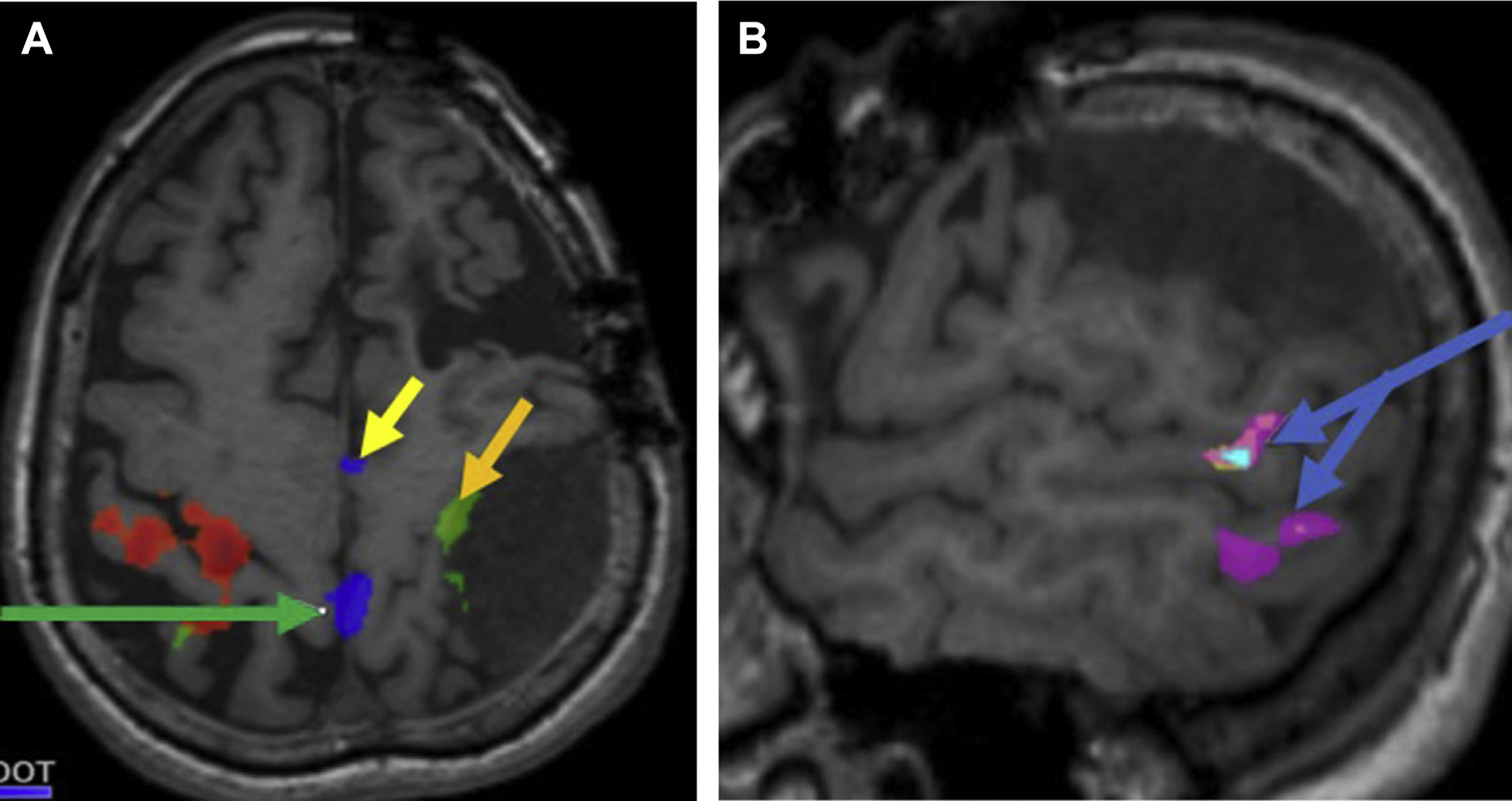
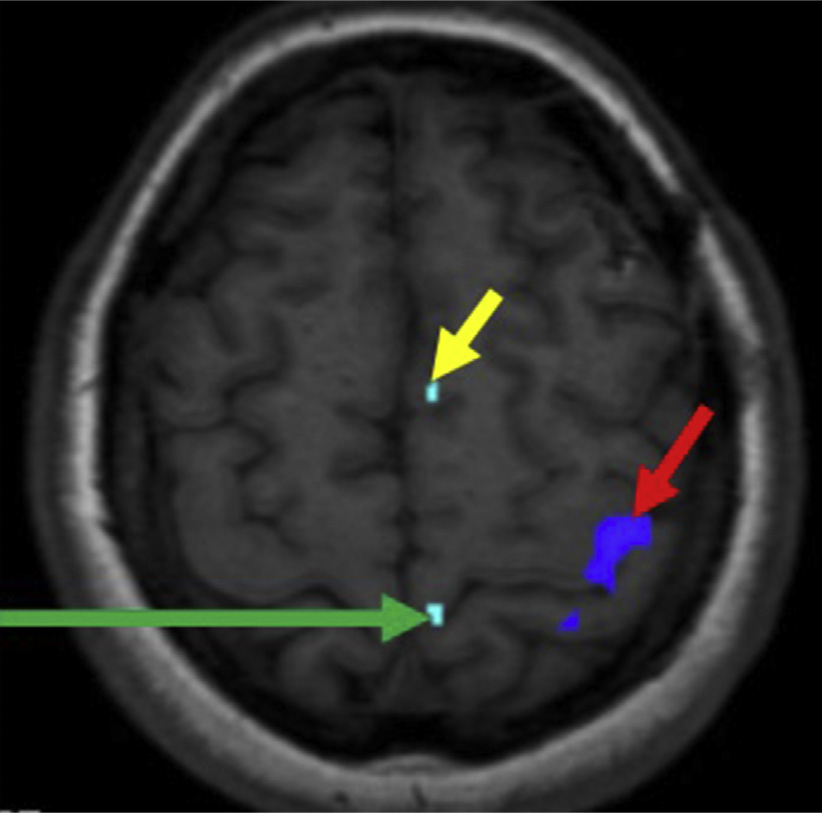
Also important are secondary motor areas, which when damaged can lead to significant morbidity, thus increasing the importance of precise fMR imaging localization. The secondary areas of the brain of interest for neurosurgical planning include the pre–supplementary motor area (pre-SMA) and the supplementary motor area (SMA). The SMA consists of a posterior SMA, which is most commonly identified adjacent to the precentral sulcus and is normally active during motor tasks (see Figs. 1 and 2 ). The anterior portion of the SMA ( Fig. 3 ) is more active during language activation and its borders are less well defined. Recent studies have suggested that the motor region of the SMA is somatotopically arranged. Studies have shown that direct activation of the SMA influences speech, which is evident during different language tasks, such as silent verbal fluency and repetition. Motor planning is largely associated with the SMA. Within the SMA is a centralized region that remains active during both language and motor tasks. It is also acknowledged that the SMA plays an important role in the planning and execution of movements, and that both passive and active tasks can be reliably detected through BOLD fMR imaging.
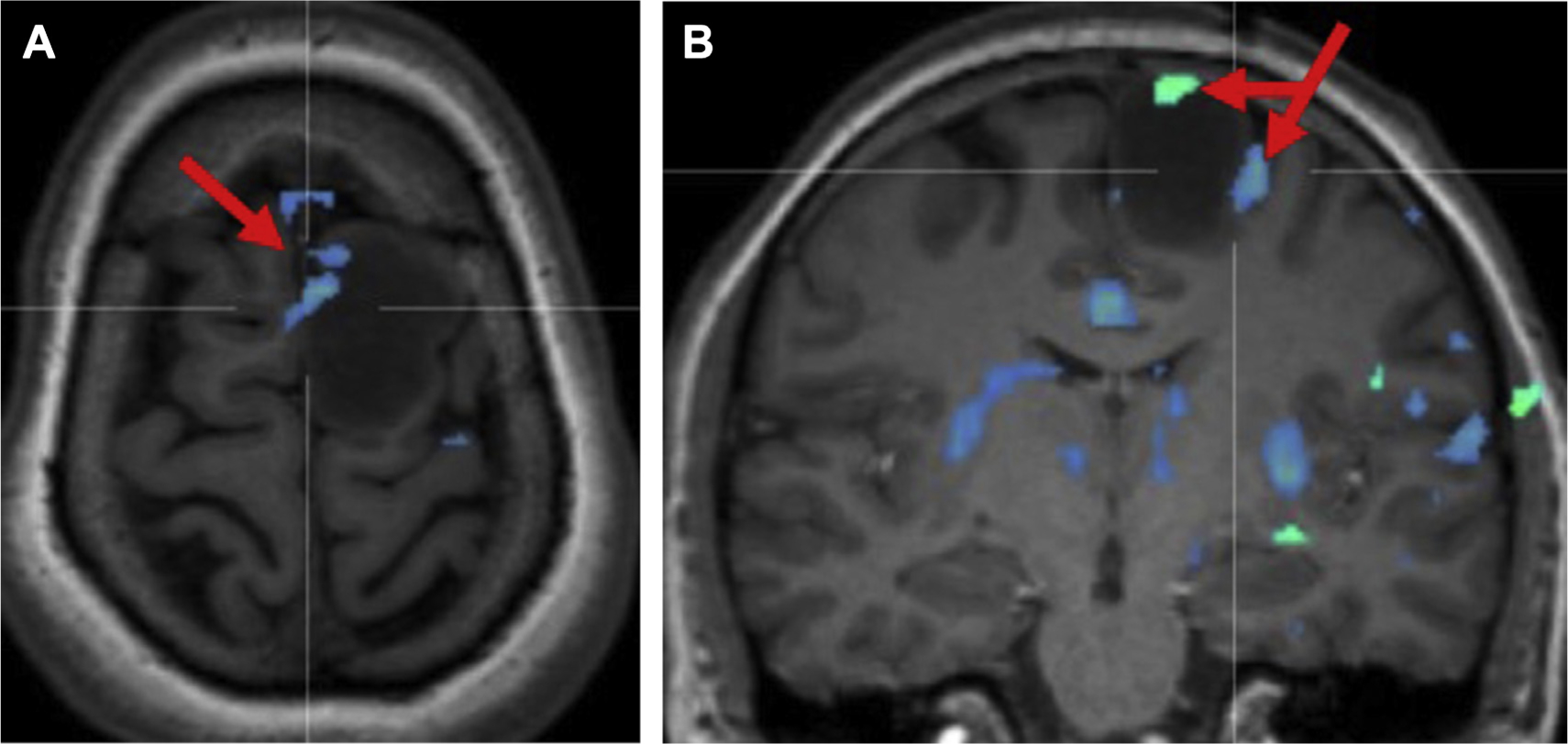
Because surgical resection of a brain neoplasm poses a risk to the eloquent cortex with potential for permanent neurologic damage, preoperative BOLD fMR imaging is particularly useful in cases where tumor and/or peritumoral edema is in close proximity to eloquent cortex. Using fMR imaging techniques, surgical teams can plan tumor resection through noninvasive visualization of the brain and analysis of lesion localization in relation to anatomic landmarks. Furthermore, fMR imaging derived information can also be used in counseling patients about the risks involved with surgical resection and in prospectively developing an appropriate surgical approach.
Language (Broca and Wernicke Areas) and Memory
The Intracarotid Amobarbital Test, otherwise known as “Wada” testing, has been considered the gold standard for determining the language-dominant hemisphere. Although it is well known that the left cerebral hemisphere is usually the dominant center for language in most of the population, adequate preoperative testing is required to properly establish cerebral language dominance ( Fig. 4 ). Owing to its high level of accuracy in predicting hemispheric language dominance and its noninvasive nature, task-fMR imaging is the standard of care in medical centers where it is available. Silent word-generation paradigms are most commonly used to elicit activation in the Broca area, most commonly located in the inferior frontal gyrus ( Fig. 5 ). Silent sentence completion is the most frequently used paradigm to elicit activation in the Wernicke area (see Fig. 5 ). Of importance is that language regions (Wernicke more so than Broca) tend to be somewhat more broadly distributed and require careful image acquisition and postprocessing to ensure accurate depiction of the language network and limit overestimation or underestimation of these regions. To improve precision of localizing Broca and Wernicke regional activity, additional language paradigms are delivered including rhyming, antonym generation, object naming, and/or passive story listening.
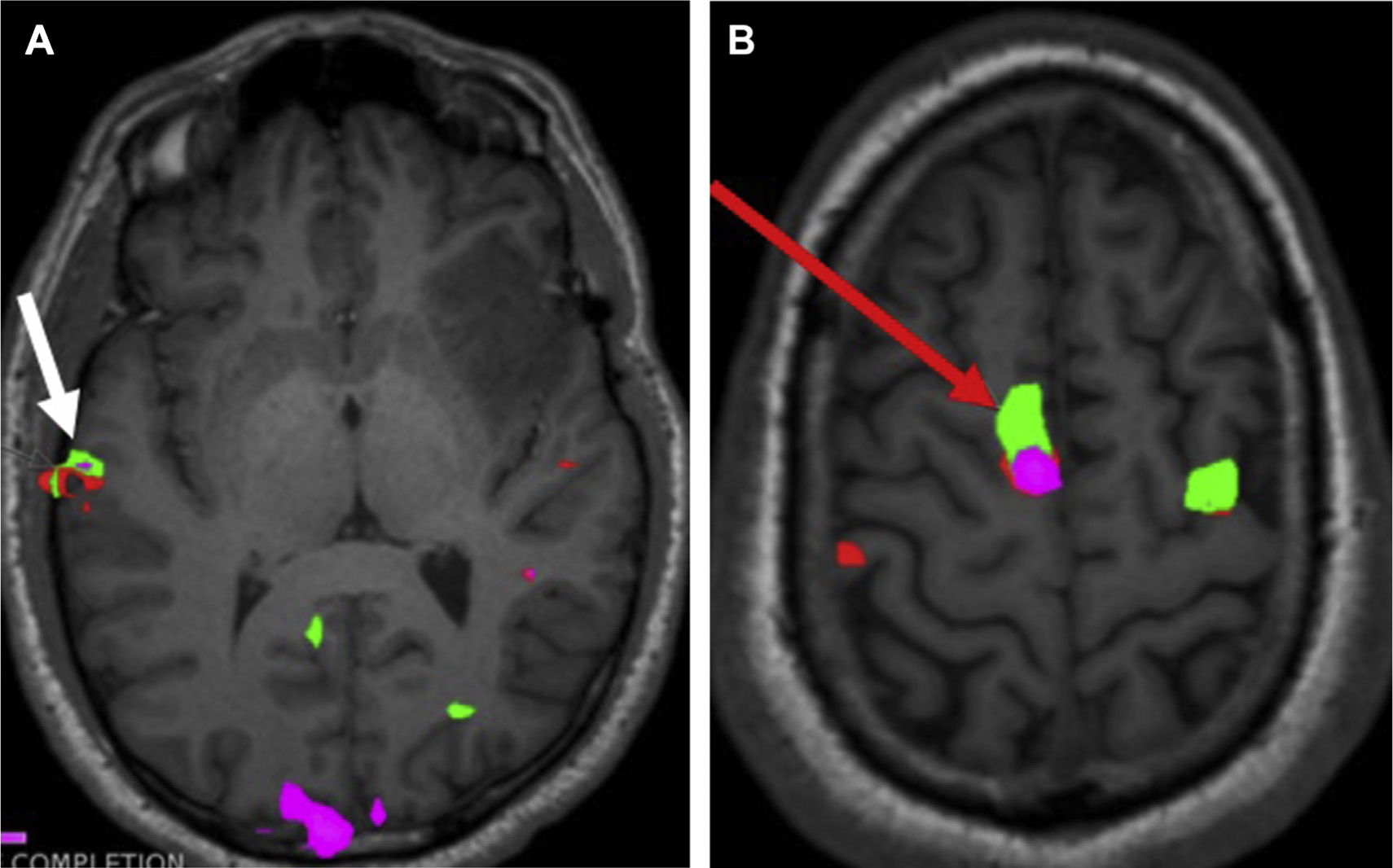
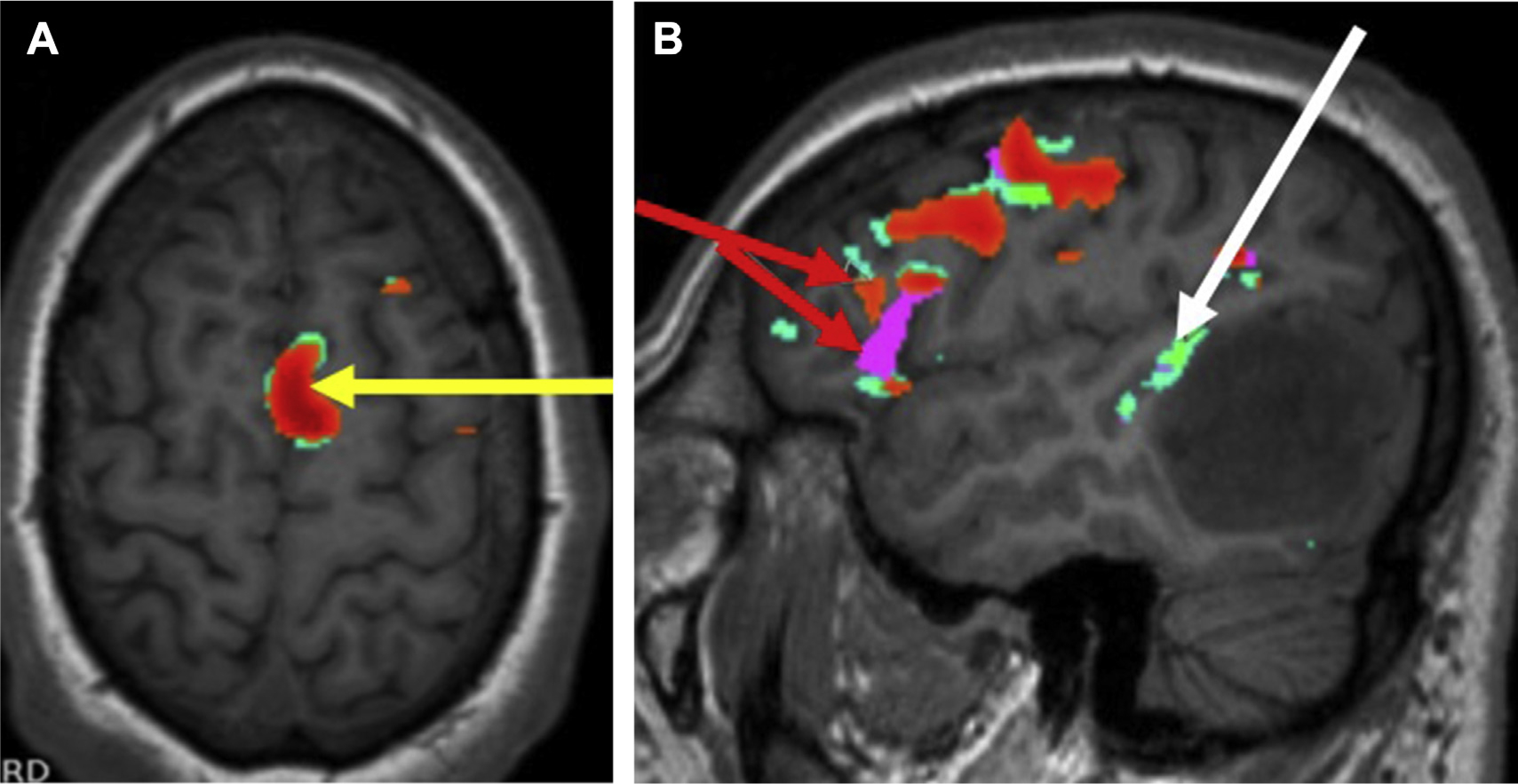
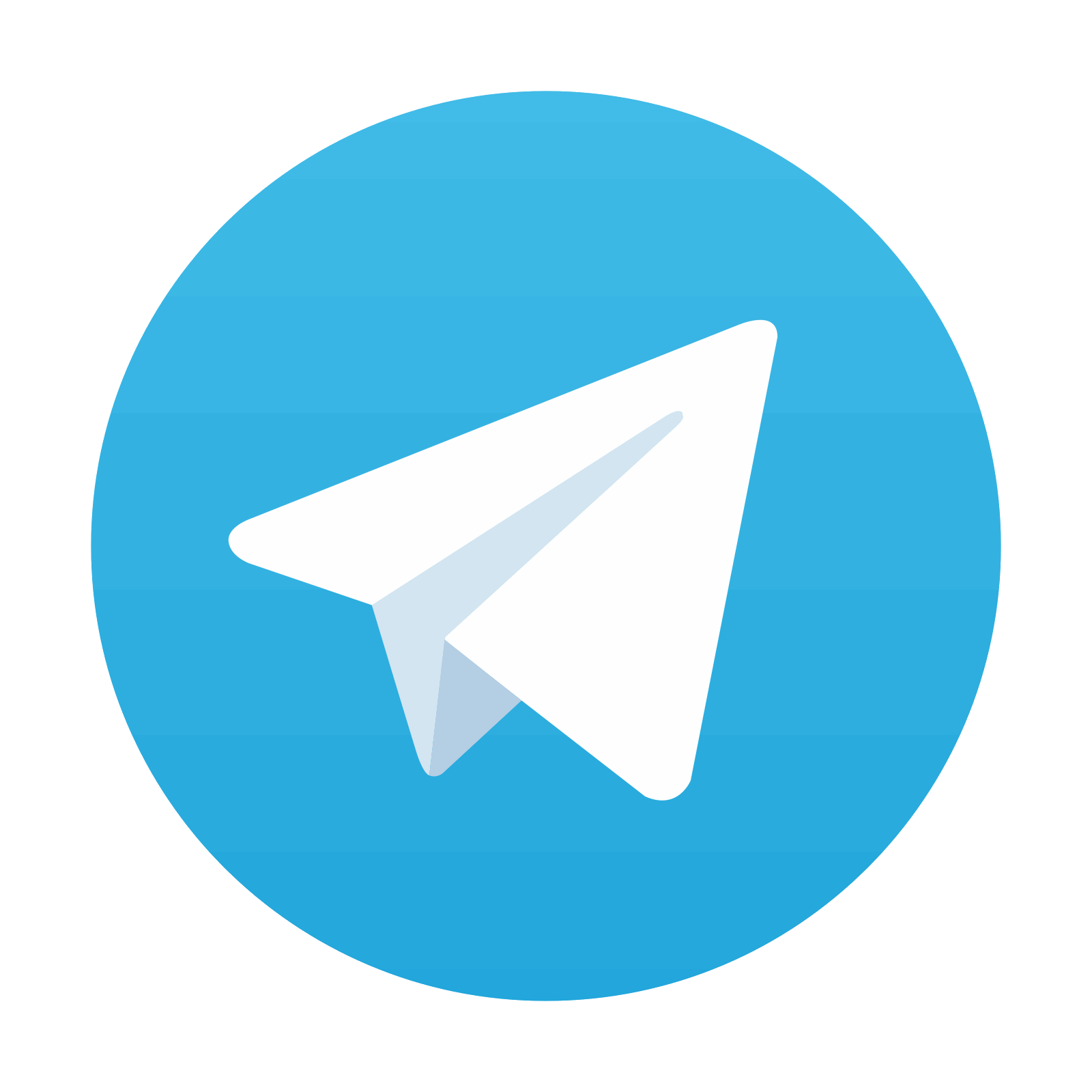
Stay updated, free articles. Join our Telegram channel

Full access? Get Clinical Tree
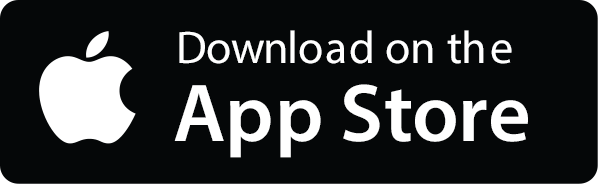
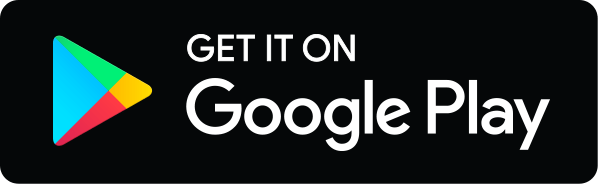
