Mark D. Iafrati • Joseph Zuniga
INTRAVASCULAR DELIVERY OF THERAPEUTIC AGENTS
Peripheral arterial disease (PAD) is a chronic condition that primarily arises from atherosclerosis and ultimately results in the occlusion of arteries to the limbs. It affects millions of Americans and is responsible for a significant burden of mortality, loss of limb, and decreased functional capacity and quality of life. Epidemiologic studies have estimated the prevalence to be at least 10% in the general population, with an almost twofold increase observed in people older than 70 years of age (approximately 15% to 20%).1,2
As PAD evolves, it causes progressive impedance in blood flow to distal tissues and subsequent ischemia. In severe cases, it causes critical limb ischemia (CLI), a condition characterized by rest pain, impaired wound healing, ulceration, and gangrene. CLI has classically been managed surgically via bypass procedures. Unfortunately, a large fraction of these procedures have proved unsuccessful due to the inadequacy of autologous veins for use as a bypass conduit, the occlusion of downstream arteries (poor “runoff”), or concurrent microvascular disease which impairs wound healing despite a successful bypass procedure. Ultimately, limb amputation remains the only option for several patients. Major limb amputation, however, is itself associated with significant (15% to 20%) perioperative mortality. For one-third of amputation survivors, amputation of the remaining limb will eventually be required.3
The need for safe and effective treatment cannot be overemphasized. The last 10 years have been witness to a dramatic shift to less invasive therapeutic approaches for PAD. In a study done by Goodney and associates4 in 2009, a threefold increase in endovascular procedures was documented alongside a 42% decrease in bypass surgery. This shift to less invasive therapy was accompanied by a 25% decrease in major lower extremity amputations and a decrease in overall mortality.4 Although other factors including improved medical management and wound care have undoubtedly played a role in this decline, the change appears to be at least partially driven by the successful use and safety of endovascular lower extremity revascularization.
Despite the tremendous progress made in the medical management and endovascular treatment of PAD, a significant number of patients referred to as no option critical limb ischemia (NO-CLI) patients have exhausted their conventional therapeutic options. Mechanical revascularization techniques, both bypass and endovascular, require an inflow source, an outflow target, and a conduit. For patients lacking any of these components, an alternative treatment approach using percutaneous or catheter-based delivery of pharmaceutical or biologic agents may offer the next best hope for symptom relief and limb salvage. This chapter will present a review of some of the more promising minimally invasive therapeutic options.
THERAPEUTIC AGENTS
Growth Factors
Angiogenesis is the formation and growth of new blood vessels from a previously existing vascular bed. This complex process entails disruption of vascular basement membranes, subsequent migration and proliferation of endothelial cells, and formation and maturation of blood vessels, all occurring under the strict regulation of growth and inhibitory factors.
Normally in adults, angiogenesis is a dormant process. The presence of angiogenesis in the setting of limb ischemia is of biologic interest as the ability to form new vessels to provide collateral arterial circulation may prove beneficial in the amelioration of ischemia caused by chronic arterial occlusion.
Multiple growth factors are involved in promoting formation of new blood vessels. Vascular endothelial growth factor (VEGF), in particular, plays a crucial role in angiogenesis mainly because its receptors are predominantly localized in the endothelial cells of blood vessels. Studies have also revealed lower levels of VEGF in the affected leg of CLI patients,5 hence supplementation with this growth factor has been hypothesized to assist in arterial collateralization.
Animal and clinical studies have shown that administration of VEGF either systemically or locally stimulates therapeutic angiogenesis.3,6,7 Its delivery, however, remains a big challenge. With a short half-life of about 1 hour, high dosages may be required to achieve desired effects.7 Systemic administration may also result in new vessel growth in undesired locations, such as in the retina, and raises the possibility of carcinogenesis.8
In 2008, the U.S. Food and Drug Administration reviewed the potential for carcinogenesis with prolonged use of the topical platelet-derived growth factor becaplermin. It was concluded that the increase in the risk of death from cancer in patients with prolonged exposure to topical becaplermin was five times higher than in those patients who did not use the product.9
Given the nonspecificity of delivering growth factors via infusion catheters, several delivery systems have been developed to limit systemic effects and improve target specificity. The introduction of a delivery vehicle made of cross-linked DNA–gelatin nanospheres greatly increased the stability of DNA, transfection efficiency, and target specificity.
Animal studies have shown the use of intra-arterial gene transfer via angioplasty using balloons coated with plasmid VEGF in improving blood supply to the ischemic limb.10–12 Isner et al.13 in 1996 reported that this method worked on a 71-year-old patient with limb ischemia and tissue loss. They administered human plasmid VEGF to the popliteal artery using a hydrogel-coated angioplasty balloon and reported increased collateral vessel formation on follow-up angiography at 4 weeks. Adverse events directly related to angiogenesis included the development of spider angiomas on the ankle and foot of the same extremity that was treated. Transient peripheral edema was also observed in the treated extremity likely due to the increased vascular permeability induced by VEGF. This was treated with diuretics and resolved by 4 weeks. Despite improvement in collateral flow, the gangrene could not be reversed and the patient eventually required a major amputation about 5 months after the procedure.8,13 Multiple randomized controlled trials (RCTs) using intra-arterial as well as intramuscular transfer of growth factor genes have been subsequently been reported.
Hammer and Steiner14 in 2013 analyzed 12 RCTs studying local administration of growth factors (VEGF, FGF, HGF, Del-1, HIF-1 alpha) using plasmid or viral gene transfer by intra-arterial or intramuscular injections. A total of 1,494 patients with the majority suffering from CLI (64 %) were included. This meta-analysis showed neither a significant benefit nor harm for gene therapy for all-cause mortality (odds ratio [OR] 0.88; 95% confidence interval [CI], 0.62 to 1.26), amputations (OR 0.64; 95% CI, 0.31 to 1.31), or ulcer healing (OR 1.79; 95% CI, 0.8 to 4.01). Despite the potential benefit seen from the individual clinical trials, no clear overall benefit was seen from gene therapy for PAD regardless of severity.14
Prostanoids
Prostaglandins are lipid compounds known to exert significant vasoactive effects. Specific types of prostaglandins are known to produce vasodilation, motivating researchers to explore the application of this property in the management of CLI.
When used either alone or in conjunction with angioplasty, intravenous or direct intra-arterial administration of prostaglandin E1 (PGE1) has been reported to markedly improve microcirculation.15,16 This is evident through enhancement in efficacy parameters such as transcutaneous oxygen tension and ankle–brachial index (ABI). The effect of PGE1 is attributed not only to its vasodilatory property but also to several other factors such as inhibition of ischemia-induced neutrophil activation, reduction of platelet activation, improvement in hemorheologic properties and cellular metabolism, reduction in the number of circulating endothelial cells, and inhibition of adhesion molecule expression.15
Heider and associates16 discussed the role of PGE1 post percutaneous transluminal angioplasty (PTA). They demonstrated that angiography causes significant impairment of peripheral microcirculation in patients with intermittent claudication. Peripheral oxygen tension is significantly reduced after PTA and remains decreased for the next 4 weeks. This impairment can be addressed with PGE1 therapy by exploiting two of its pharmacologic effects: (1) the vasodilatory property, which diminishes angioplasty-caused vasoconstriction, and (2) its endothelial-protective effect, which has been shown to protect endothelial function after disturbance by nonionic contrast material.
In a recent meta-analysis by Ruffolo et al.17 in 2010, 20 publications describing RCTs on the use of prostanoids on CLI were reviewed. Prostanoids were found to be effective in relief of rest pain (risk ratio [RR] 1.32; 95% CI 1.10 to 1.57, P = .003) and ulcer healing (RR 1.54; 95% CI, 1.22 to 1.96). Iloprost in particular showed favorable results in terms of preventing major amputations (RR 0.69; 95% CI, 0.52 to 0.93). Despite these positive results, however, the authors of the meta-analysis believe that there is no conclusive evidence with regard to long-term effectiveness and safety of different prostanoids in patients with CLI.
Stem Cell
The management of CLI from severe PAD is a challenge for the vascular medicine specialists. This is especially true for patients on whom conservative management has failed and who, at the same time, are not suitable for either surgical or endovascular revascularization due to existing comorbidities or anatomy.
A promising treatment strategy emerged about a decade ago for patients whose only other option in the past was to undergo limb amputation. The inspiration for this treatment arose from the observation that cells in the bone marrow or the peripheral blood that express CD34 surface markers have the capacity to transform into functional endothelial cells in vitro. 18,19 These stem cells have the capability of producing angiogenic factors and thus may play a potential role in the management of tissue ischemia by facilitating blood vessel development. Several studies done in the early 2000s reported remarkable outcomes when stem cell therapy was used to improve peripheral blood circulation in preclinical models. 20,21
The Therapeutic Angiogenesis using Cell Transplantation (TACT) study was the first clinical trial to ascertain the feasibility of this concept. It demonstrated that the intramuscular implantation of autologous bone marrow cells into critically ischemic legs significantly improved ABI, transcutaneous oxygen pressures, and rest pain.22 Multiple clinical trials have yielded similar encouraging results.23,24 Although most cellular therapy for CLI has been administered intramuscularly, a few studies have reported intra-arterial injection of bone marrow aspirate either alone or in combination with an intramuscular injection produced no significant difference in treatment result when compared to intramuscular implantation. These studies claim that intra-arterial administration ensured that the stem cells would reach all targeted vessels in an antegrade manner, allowing perfused ischemic muscle regions to receive a high concentration of stem cells. However, concern remains about the possibility of arterial puncture site complications, downstream arterial thrombosis, and the possibility that the cells will not reach the intended tissues of interest in patients with extremely poor baseline perfusion.25
Current knowledge supports intramuscular administration of bone marrow cells as a relatively safe, feasible, and potentially effective treatment for the patient with CLI which may reduce the need for major amputations. A recent systematic review of RCTs reporting intramuscular administration of bone marrow mononuclear cell concentrates in 291 NO-CLI patients (treatment group: 149, control: 142) reported a reduction in major limb amputation risk from 25.4% to 14.8% (P = .03).23 Furthermore, the treatment was found to be safe with few treatment-related complications. Pivotal studies are underway with the objective to verify the efficacy of this novel therapy.
Anti-inflammatory Agents
The process of restenosis after vessel injury is mainly thought to involve inflammatory cells located in the vessel adventitia such as macrophages, dendritic cells, lymphocytes, neutrophils, and mast cells. Within hours after injury, these cells initiate the release of other inflammatory modulators such as cytokines, growth factors, and reactive oxygen species, which contribute to smooth muscle and adventitial cell proliferation. This same inflammatory response has also been noted to occur after vascular procedures.26,27
In line with the hypothesis, Owens et al.27 did a prospective, first-in-man study to determine the feasibility and safety of percutaneous perivascular delivery of dexamethasone after treatment of the femoral–popliteal segment. Using an over-the-wire microinfusion catheter, a microneedle was deployed into the vessel adventitia to deliver dexamethasone. Twenty patients with a mean age of 66 years with Rutherford category 2 to 5 were enrolled in this study. The range of lesion length treated was 8.9 ± 5.3 cm, half of which involved chronic total occlusions. Technical success of drug delivery was 100%, and no procedural or drug-related adverse events were noted. The preliminary results at 6 months suggest that perivascular dexamethasone treatment may improve outcomes following angioplasty to the femoral and popliteal arteries. The mean Rutherford score decreased from 3.1 ± 0.7 (median, 3.0) preoperatively to 0.5 ± 0.7 at the end of the study period (median, 0.0, P < .00001). The ABI index increased from 0.68 ± 0.15 to 0.89 ± .19 (P = .0003). Only two lesions reoccluded.28 These preliminary results are encouraging.
Antiproliferative Agents
Another mechanism by which restenosis has been postulated to occur is through neointimal hyperplasia. In line with this, antiproliferative agents have been used to prevent restenosis after vascular procedures. Among these agents, paclitaxel, sirolimus, and everolimus have been well studied for this purpose. Their use was heightened with their incorporation into balloons and stents.28,29 Because the function of these antiproliferative agents is intimately tied to their delivery techniques, a more detailed description of these agents is presented in the following sections describing device considerations.
DEVICES
In the modern era of endovascular interventions for PAD, multiple devices have been developed to deliver therapeutic agents as close as possible to problematic regions with the goal of localizing treatment to prevent undesirable systemic effects.
The standard endovascular technique of using guidewire and catheter has undergone very minimal change throughout the years, and improvements were primarily centered on modifying the physical attributes of the devices being used.
Porous Balloon Catheters
A porous balloon catheter is a variation of the standard balloon catheter used in percutaneous angioplasty. With its reservoir and peripheral perforations, it is well suited for the administration of various therapeutic agents during or after balloon angioplasty.
The porous balloon catheter was developed with the intent to create an instrument that could deliver high concentrations of therapeutic agents locoregionally to the involved segment of the vasculature, thus allowing medications to work without exposing the entire circulation to the agent’s effects. Wolinsky and Thung,30 through their work with canine arteries in 1990, pioneered the use of porous balloon catheters in local drug delivery. This technique has been particularly significant in the prevention of restenosis. By itself, balloon angioplasty causes vessel damage, which may produce restenosis of the treated segment in the long term. It has been shown that the addition of therapeutic agents, such as reviparin, decreases the incidence of restenosis.31
Several factors such as the size of the particles within the infusate, infusion pressure, and infusate volume should be considered when using porous balloon catheters. Large particles cause more severe vascular damage, incite an inflammatory reaction, and promote intimal thickening. Similar detrimental effects are seen when trying to force a large volume of infusate through the porous balloon catheter. The use of infusates containing nanoparticles has been proven to cause less vascular damage than an infusate containing microparticles. Also with smaller particles, less infusion pressure is required to achieve deeper agent penetration with potentially greater treatment effect.
Porous balloon catheters are also used in thrombolysis. Specifically, the ClearWay (Atrium Medical, Hudson, New Hampshire) balloon catheter (Fig. 44.1) allows direct administration of thrombolytic agents within the thrombus. Benefits observed with the use of this catheter included a decreased lytic requirement and reduced duration of thrombolysis,32 thus reducing the potential for hemorrhagic complications related to prolonged exposure to the thrombolysis agent.
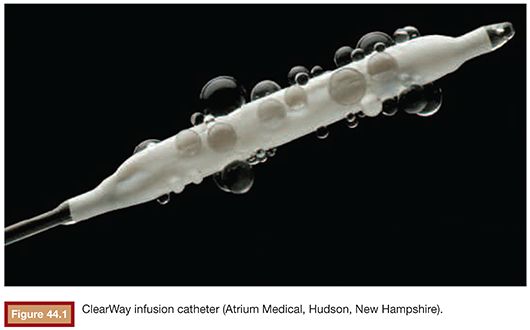
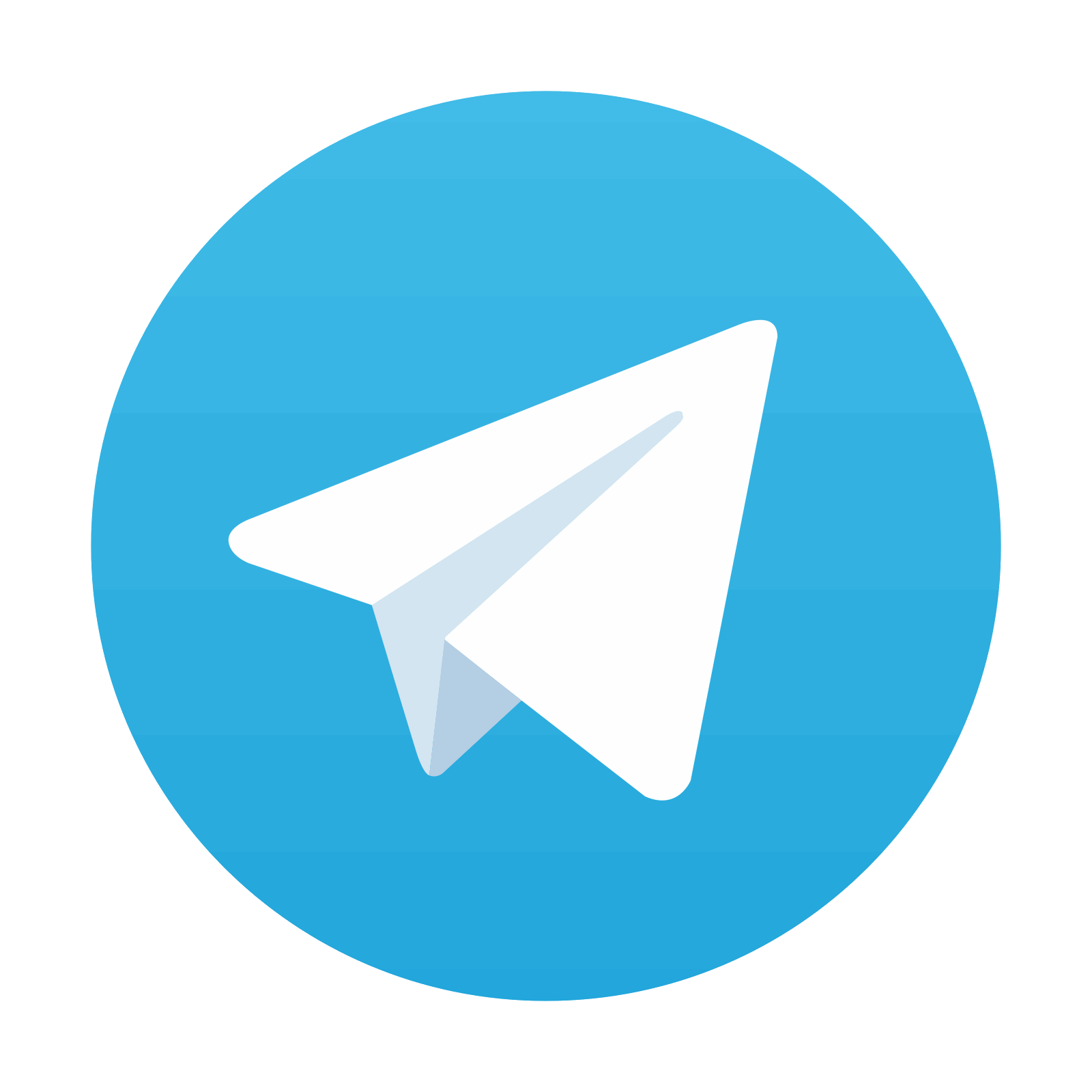
Stay updated, free articles. Join our Telegram channel

Full access? Get Clinical Tree
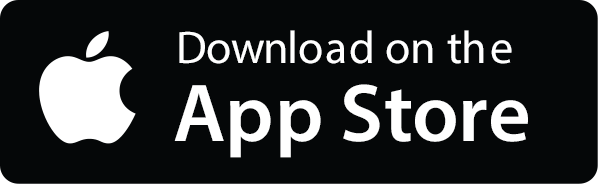
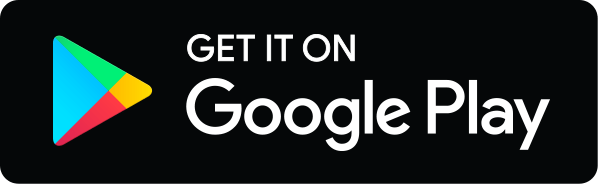