CHAPTER 12 White Matter
The supratentorial white matter is the gross structure formed by the axons that arise from neurons situated within the gray matter (Fig. 12-1).1–3 The term white matter derives from the glistening fatty “white” myelin sheath that surrounds many of the axons. These axons may be heavily myelinated, lightly myelinated or unmyelinated. The proportion of myelinated fibers increases with phylogenetic, embryologic, and developmental growth. From shrews to whales, the composition of the cerebral white matter shifts from compact, slow-conducting unmyelinated axons to large fast-conducting myelinated axons.4 The fastest of these have conduction times of 1 to 5 ms across the neocortex and less than 1 ms from eye to brain.4
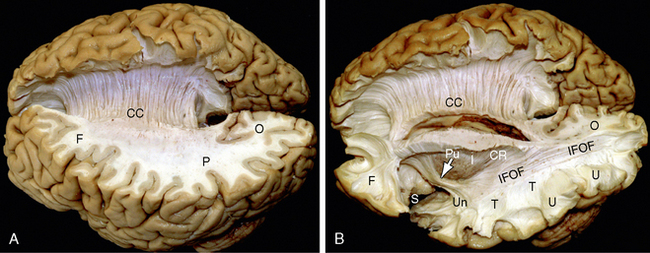
FIGURE 12-1 Gross dissection of the human brain by the Klingler technique (Dr. Nicklaus Krayenbühl). A, View from above and the left side. Axial section through the frontal (F), parietal (P), and occipital (O) lobes of the left hemisphere discloses the white matter within the centrum semiovale (F-P-O) and the upper border of the corpus callosum. Klingler dissection of the medial surface of the right hemisphere exposes the multiple, superiorly arching commissural fibers (CC) that extend through the corpus callosum into the medial portion of the opposite hemisphere. B, Further dissection of the left hemisphere exposes projection fibers (uncinate fasciculus [Un], internal capsule [I]), short association U fibers (U), and long association fibers (inferior fronto-occipital fasciculus [IFOF]) within the white matter. The uncinate fasciculus (Un) arches over the sylvian fissure (S) to interconnect the temporal lobe (T) with the frontal lobe (F). The inferior fronto-occipital fasciculus extends the length of the hemisphere to interconnect the occipital lobe with the ipsilateral frontal lobe. Its fibers characteristically compact into a narrow bundle (white arrow) where the tract traverses the floor of the external capsule just inferolateral to the putamen (Pu). In this position, it courses immediately superior to the fibers of the uncinate fasciculus. The vertical fibers of the corona radiata (CR) converge to form the compact internal capsule (I) that passes medial to the putamen.
Commissural fibers are bihemispheric fibers that cross the midline to interconnect the two cerebral hemispheres. Homotopic commissural fibers interconnect corresponding regions of the two hemispheres.2,3 Heterotopic commissural fibers connect one cortical area with noncorresponding areas of the contralateral hemisphere.2 In many cases, the regions to which heterotopic commissural fibers pass are the same regions to which the contralateral cortex sends association fibers within its own hemisphere.2
Projection fibers are unihemispheric fibers that convey impulses from the cortex to subcortical regions such as the basal ganglia, diencephalon, brain stem, and the spinal cord (often after crossing over the midline in the brain stem).2,3
Association fibers are unihemispheric fibers that connect diverse regions of the same hemisphere. Short association fibers pass through the deep layers of the cortex and/or the subcortical white matter as short curved U fibers to interconnect adjacent cortical areas.2 Long association fibers lie more deeply within the white matter and span large distances across a single hemisphere.
Within the white matter, the axons gather into bundles that are classified by their position and function. The nomenclature of fiber bundles is highly variable. On average, rounded bundles of fibers are designated striae, fascicles, and tracts, whereas broad sheets of fibers are designated laminae, capsules, and radiations. These names are broadly descriptive of the size and shape of the fiber bundles but are often used historically or idiosyncratically with little adherence to precise definition.5 With that understanding, here are some descriptions:
Striae are thin bundles of fibers that pass longitudinally across the brain.
Fascicles are microscopically determinable groups of fibers.5
Tracts are groups of axons subserving a similar or corresponding function.5
Laminae are relatively thin sheets of axons that proceed in a similar direction.
Capsules are curved sheets of fibers that partially enclose gray matter structures.
Radiations are broad sheets of fibers that arch together to or from one target.
Decussations are crossings of two fiber tracts in the midline. This term has been generalized to signify both the sites at which the fiber tracts cross the midline and the names of the crossing tracts.
ANATOMY
Gross Appearance
Grossly, the white matter of the cerebral hemispheres forms the thick layer between the cortical gray matter of the surface and the deep gray nuclei of the basal ganglia and thalami (Fig. 12-1). Superior to the lateral ventricles the white matter assumes the shape of each hemisphere, so it is designated by the aggregate term centrum semiovale. Peripherally, the white matter extends outward into every gyrus to form the central white matter cores (digitations) of the gyri. Centrally, it extends medially, bilaterally, to cross the midline as commissures and decussations and extends craniocaudally to form the corona radiata and the capsules of the hemispheres. Table 12-1 lists some of the fiber tracts discussed in this chapter and summarizes a few of their key connections.1–8 These will be elaborated on within each section.
The fiber tracts of the brain course through the cerebral hemispheres in multiple directions within multiple overlapping layers. On average, commissural fibers course transversely between the hemispheres. Projection fibers course obliquely craniocaudal within one hemisphere and may extend caudally outside the hemisphere. Long association fibers course obliquely, anteroposteriorly, within one hemisphere. Careful dissection of the brain from the lateral surface, medial surface, and superior surface discloses the major layers of these fibers and their orientation (Figs. 12-2 and 12-3; Box 12-1).9–11
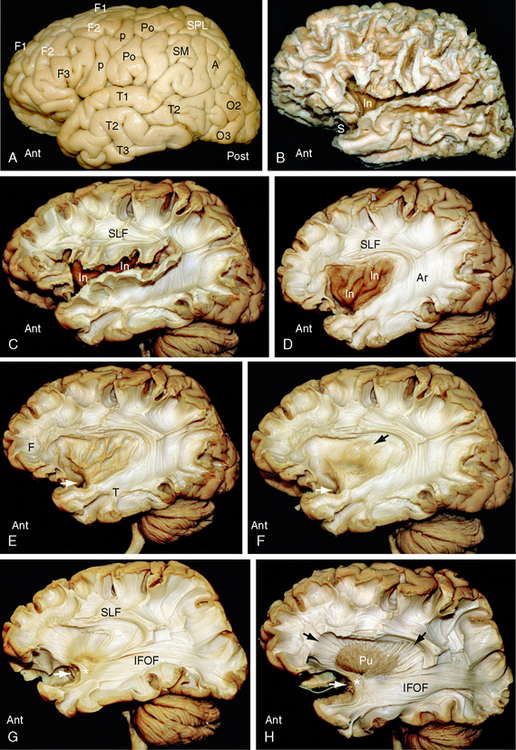
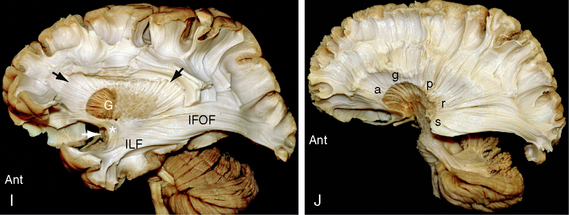
FIGURE 12-2 Dissection of the human brain from the lateral aspect by the Klingler technique (Dr. Nicklaus Krayenbühl). Ant, anterior; Post, posterior. A, Lateral surface of the hemisphere. Removal of the leptomeninges and vessels exposes the cortical gray matter. F1, superior frontal gyrus; F2, middle frontal gyrus; F3, inferior frontal gyrus; p, precentral gyrus; Po, postcentral gyrus; SPL, superior parietal lobule; SMG and AG, the supramarginal gyrus and angular gyrus of the inferior parietal lobule; T1, superior temporal gyrus; T2, middle temporal gyrus; T3, inferior temporal gyrus; O2, middle occipital gyrus; O3, inferior occipital gyrus. B, Scraping away the cortical gray matter exposes the white matter cores (digitations) of the gyri and the short association U fibers that interconnect them. The insula (In) just becomes visible through the now-wider sylvian fissure. C, Resecting the superficial digitations exposes the superior longitudinal fasciculus (SLF) superior to the sylvian fissure and insula (In). Short association U fibers interconnect the adjacent gyri. D, Resecting the overhanging margins of the frontal, parietal and temporal opercula exposes the underlying insula (In) and the relationship of the SLF and the arcuate fasciculus (Ar) to the insula (In). E, Careful resection of the insular cortex then exposes the thin layer of white matter that makes up the underlying extreme capsule. Fiber tracts passing from the temporal lobe (T) to the frontal lobe (F) must pass deep to the limen insulae (white arrow) through the anterior inferior portion of the extreme capsule. F, Removal of the extreme capsule exposes the gray matter of the claustrum (brownish color indicated by the black arrow). White arrow as in E. G, Resection of the claustrum exposes the fibers of the external capsule and fibers passing toward the internal capsule (see H and I). The temporal white matter displays the inferior fronto-occipital fasciculus (IFOF) and the uncinate fasciculus (asterisk) (see also Fig. 12-1B). The inferior fronto-occipital fasciculus forms part of the external sagittal stratum lateral to the temporal and occipital horns. White arrow as in E and F. H, Resection of the external capsule exposes the putamen (Pu) and its relationship to the radiating fibers (black arrows) converging toward the internal capsule deep to the putamen. The anterior end of the long inferior fronto-occipital fasciculus (IFOF) courses around the deep end (white arrow) of the sylvian fissure with and immediately superior to the uncinate fasciculus (asterisk). I, Partial removal of the anterior putamen exposes the medially positioned globus pallidus and the course of the fibers (black arrows) converging to the internal capsule deep to both nuclei. White arrow as in E to G. J, Complete resection of the putamen and globus pallidus (lenticular nucleus) and the uncinate fasciculus reveals the radial array of the fibers forming the corona radiata. The curvature of the fibers about the (resected) lenticular nucleus shows the positions of the anterior limb (a), genu (g), posterior limb (p), retrolenticular (r), and sublenticular (s) portions of the internal capsule. Resection of much of the anterior temporal lobe and more lateral portions of the posterior temporal and occipital lobes exposes posterior portions of the optic radiations as they traverse the retrolenticular portion of the internal capsule and then pass posteriorly to the occipital cortex in the external sagittal stratum.
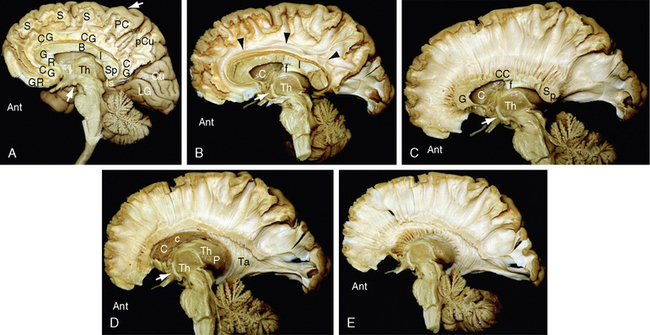
FIGURE 12-3 Dissection of the human brain from the medial aspect by the Klingler technique (Dr. Nicklaus Krayenbühl). A, Medial surface of the brain after removal of the cortical gray matter of the frontal and parietal lobes exposes the rostrum (R), genu (G), body (B), isthmus (I), and splenium (Sp) of the corpus callosum, the digitations and U fibers of the gyrus rectus (GR), cingulate gyrus (CG), superior frontal gyrus (S), paracentral lobule (PC), precuneus (pCu), and isthmus (Is) of the cingulate gyrus. The cortex of the cuneus (Cu) and lingual gyrus (LG) of the occipital lobe remains in place. The upper white arrow indicates the central sulcus on the medial surface. Th, medial surface of the thalamus; lower white arrow, mammillary body. B to E, The temporal lobe has been resected. B, Further dissection reveals the cingulum bundle (black arrowheads) coursing through the cingulate gyrus as it arches over the corpus callosum. The midportion of the fornix has been resected to expose the caudate nucleus (C). The posterior portion of the fornix (f) is applied to the undersurface of the corpus callosum at the isthmus (I). The anterior column (white arrow) of the fornix extends to the mammillary body (obscured here but visible in the subsequent images). The thin curved stria medullaris thalami courses along the medial wall of the thalamus (Th) to interconnect the septal nuclei with the habenular nuclei. The position of the stria medullaris thalami marks the site at which the roof of the third ventricle inserts into the medial surface of the thalamus. Additional portions of the thalamus are just becoming visible posterosuperior to the stria. C, Removal of the digitations and U fibers of the medial gyri of the hemisphere exposes the commissural fibers (CC) passing to and from the frontal, parietal, and occipital lobes to form the corpus callosum. Temporal contributions to the corpus callosum are not shown in this specimen. Other labels are as in A and B. D, Resection of additional portions of the corpus callosum and entry into the lateral ventricle exposes the inner surface of the lateral wall of the ventricle along much of its length. Anteriorly, the head (C) and body (c) of the caudate nucleus form the inferolateral wall of the lateral ventricle. Further posteriorly, the thalamus (Th) forms the floor of the body of the lateral ventricle. The tapetum (Ta) of the corpus callosum forms the lateral wall of the temporal horn, atrium, and occipital horn of the lateral ventricle. A far greater extent of the medial surface of the thalamus is now seen anteroinferior and posterosuperior to the curved stria medullaris thalami. Other labels are as earlier designated. E, Further removal of the corpus callosum and removal of much of the caudate nucleus exposes the thalamic peduncles converging/diverging to interconnect the thalamus with all portions of the cortex (see also Fig. 12-14D).
BOX 12-1 Klingler Technique for White Matter Dissection
Data from Klingler J, Gloor P. The connections of the amygdala and of the anterior temporal cortex in the human brain. J Comp Neurol 1960; 115:333-369; Türe U, Yasargil MG, Friedman AH, Al-Mefty O. Fiber dissection technique: lateral aspect of the brain. Neurosurgery 2000; 47:417-427; and Yasargil MG. Surgical anatomy of supratentorial midline lesions Neurosurg Focus 2005; 18(6b):E1.
Microscopy
Histologic sections of the cerebral white matter demonstrate the orientations and bundling of the axons and their myelin sheaths (Figs. 12-4 and 12-5). The cerebral white matter contains neuroglial support cells such as oligodendroglia and astrocytes but does not contain neuronal cell bodies.12 The oligodendroglial cells are moderate-sized cells that extend short branched processes to ensheath adjacent segments of up to 40 neighboring axons (Fig. 12-6).12 Within each segment, the membrane process of the oligodendroglial cell wraps spirally around the axon and then condenses into a tight myelin sheath of up to 150 layers.13 The myelin sheath remains continuous with the cell membrane of the oligodendroglial cell but displays a simplified chemical composition. Because each segment of myelin derives from one oligodendroglial cell, the myelin sheath of each axon is composed of multiple different segments supplied by different oligodendroglial cells. These segments are separated from each other by “gaps” designated the nodes of Ranvier. In myelinated axons, nerve impulses “jump” rapidly down the axon from node to node (saltatory conduction), rather than pass slowly along the entire length of the axon.
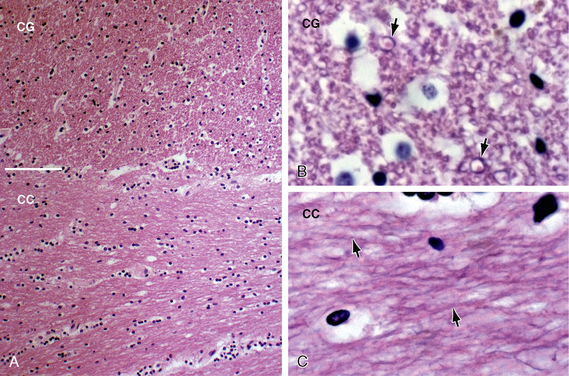
FIGURE 12-4 White matter histology. Hematoxylin and eosin (H&E) stain colors the myelin and cytoplasm shades of pink and the nuclei blue. A, Coronal section through the interface (white line) of the corpus callosum (CC) with the white matter of the cingulate gyrus (CG) (original magnification, ×10). Routine H&E sections resolve the transverse orientation of the callosal fibers (CC) versus the longitudinal orientation of the cingulate white matter (CG) but usually do not distinguish the axon and myelin sheath from the surrounding neuropil. B and C, Magnified coronal sections of the cingulate gyrus (B) and corpus callosum (C) (original magnifications, ×60). Higher magnification shows the myelin sheaths (black arrows) displaced away from the cytoplasm of the central axon by fixation artifact. In cross section (B), the myelin sheaths appear as thin, slightly darker “donut rings” surrounding small slightly lighter dot-like central axons. In longitudinal sections (C), the sheaths (black arrows) appear as thin dark lines coursing in bundles.
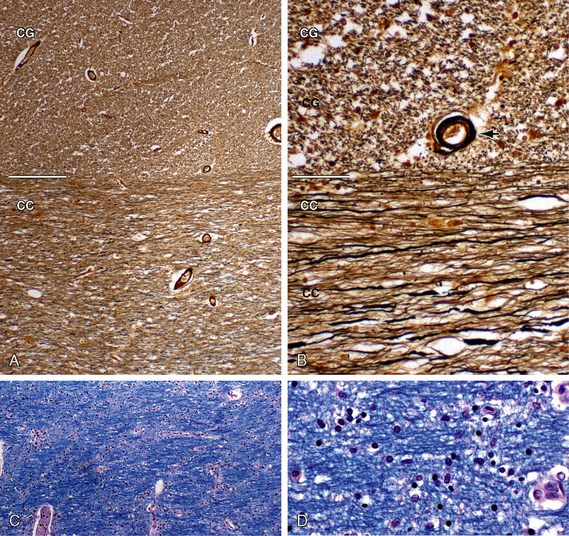
FIGURE 12-5 White matter histology. Axons and myelin. A and B, Bielschowsky stain for axons (original magnifications, ×4 (A) and ×20 (B). The Bielschowsky modified silver stain renders axons black and myelin shades of brown. This clearly distinguishes the linear transverse fibers of the corpus callosum (CC) from the dot-like cross sections of the longitudinally oriented cingulate fibers (CG). Black arrow, blood vessel. C and D, Luxol fast blue (LFB) stain for myelin with H&E counterstain (original magnifications, ×4 (A) and ×20 (B). LFB stains myelin blue. H&E stains myelin and cytoplasm pink and nuclei blue. LFB stains demonstrate the differing orientations of the interwoven fibers, their aggregation into fiber bundles, and the spacing of their myelin sheaths.
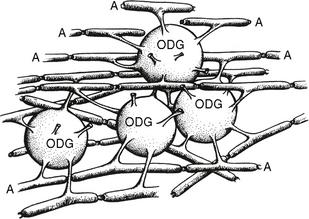
FIGURE 12-6 Each oligodendroglial (ODG) cell extends processes from its cell wall to enwrap segments of multiple adjacent axons (A). These establish the central myelin sheaths.
(Modified from van der Knaap MS, Valk J. Magnetic Resonance of Myelination and Myelin Disorders, 3rd ed. Berlin, Springer, 2005.)
The astrocytes help to establish and preserve the structure and integrity of the myelin and axons. They stimulate the oligodendroglial cell precursors to proliferate, migrate, and differentiate.12 They assist in the initial adhesion of the oligodendroglial cell processes to the axons and release growth factors that promote myelination.12 The astrocytes participate in the conduction of impulses at the nodes of Ranvier and serve to recycle the excitatory neurotransmitter glutamate at synapses.12 Astrocytes also help to maintain the cerebrospinal fluid-brain and blood-brain barriers. After damage to the white matter, the astrocytes enlarge, proliferate, and accumulate glycogen and filaments, a process designated gliosis.12 The gliosis may occur within a region of normal or regenerating myelin, or it may be seen to replace all normal tissue as a glial scar.12 Emery reviews the regulation of oligodendrocyte differentiation and myelination in detail.14
Central nervous system (CNS) white matter is approximately half myelin and half protein.12 It has a lower water content (72%) than gray matter (82%).12 As a percent of total lipid weight, the lipids of CNS myelin are cholesterol (25% to 28%), galactolipid (27% to 30%), and phospholipids (40% to 45%).12 No single lipid is unique to myelin, but high concentrations of cerebroside are typical of myelin.12 In contradistinction to the lipids, most of the myelin proteins are unique to myelin.12 Sixty to 80 percent of the total myelin protein is proteolipid protein and myelin basic protein.12 Myelin-associated glycoprotein constitutes about 1% of myelin proteins.12
The thickness of the myelin sheath varies from axon to axon. Single oligodendroglial cells that extend processes to axons of differing size establish myelin sheaths of different thicknesses about each axon. The ratio of the outer diameter of the axon to the outer diameter of the combined axon plus sheath, designated the g ratio, is stated to be relatively stable at about 0.65.15 However, the range of g ratios varies considerably across axons within a single fiber tract.16 Furthermore, the g ratio appears to increase with axon diameter. That is, larger axons have relatively thinner myelin sheaths.16
The axonal cytoskeleton contains neurofilaments and microtubules in a ratio of 5 to 10:1.16 The caliber of the axons is influenced by the number of neurofilaments and their spacing. Neurofilament number is regulated by neurofilament synthesis (gene expression). Neurofilament spacing depends on the lengths of the side arms that extend outward from the filament, perpendicular to the long axis of the filament. Phosphorylation of the side arms increases the length of the side arm and increases the interfilament distance. Such phosphorylation appears to be regulated by myelin-associated glycoprotein synthesized by the oligodendrocytes.
Commissural Fibers
Commissural fibers are fibers that cross the midline to interconnect the two hemispheres. Homotopic commissural fibers interconnect corresponding regions of the two hemispheres.2 Heterotopic commissural fibers connect a cortical area with noncorresponding areas of the contralateral hemisphere.2 In humans, the three major supratentorial commissures are the corpus callosum (great commissure), the anterior commissure, and the hippocampal commissure (synonyms: commissure of the fornices, psalterium). The corpus callosum and the major portion of the anterior commissure are formed by crossing axons that arise from the neocortex. A small anterior portion of the anterior commissure designated the basal telencephalic bundle is formed by crossing paleocortical and archicortical fibers. The hippocampal commissure is formed by crossing archicortical fibers (Table 12-2). Other, less prominent commissures include the anterior hypothalamic commissure (of Ganser), dorsal supraoptic commissure (of Meynert), ventral supraoptic commissure (of Gudden), the posterior commissure, the habenular commissure, and the commissures of the superior and inferior colliculi.
In rhesus monkeys, only 2% to 3% of all cortical neurons give rise to axons that cross to the opposite hemisphere.17 Each commissure carries different numbers of crossing fibers. Because the crossing axons vary in size and in degree of myelination, there is no constant relationship between the cross-sectional area of the commissure and the numbers of crossing fibers. There is no consistent relationship or proportion between the numbers of axons crossing in one tract versus another.17 Data from rhesus monkeys are provided in Table 12-2 to convey a feeling for the numbers and types of fibers within each commissure of a primate.17
Corpus Callosum
The corpus callosum is the largest connective structure in the brain (Fig. 12-7). More than 200 million to 300 million axons interconnect the two cerebral hemispheres over a broad span. Medially, these fibers converge into a thick, compact plate of fibers that arches beneath the interhemispheric fissure. Laterally, the thick sheet of fibers fans out into wide areas of the two hemispheres. These interhemispheric connections do not simply link all portions of the two hemispheres. Instead, the commissural fibers link only those areas functionally related to the midline.3 In the somatic cortex, commissural fibers through the body predominantly link those cortical areas representing the trunk, rather than those representing the peripheral hands and feet. In the visual cortex, commissural fibers through the splenium interconnect only those cortical areas representing the vertical meridian.3
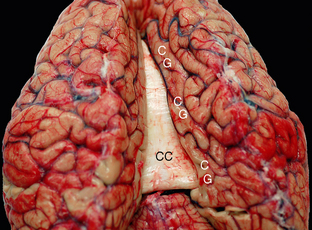
FIGURE 12-7 Fresh unfixed cadaver brain viewed from above and behind. The commissural fibers form transverse ridges on the superior surface of the corpus callosum (CC) as they cross between the two cerebral hemispheres. The cingulate gyri (CG) encircle the corpus callosum on each side.
Grossly, the corpus callosum displays a rostrum, genu, body, isthmus, and splenium (Figs. 12-3 and 12-8). The isthmus of the corpus callosum is the point at which the fornix abuts the undersurface of the corpus callosum. Functionally, the isthmus divides the corpus callosum into two portions: (1) a prominent anterior associative segment that carries the commissural fibers of the frontal associative cortex and (2) a smaller posterior splenial segment that carries the commissural fibers of the primary visual (calcarine) cortex as well as the more associative posterior parietal and medial occipitotemporal cortices.
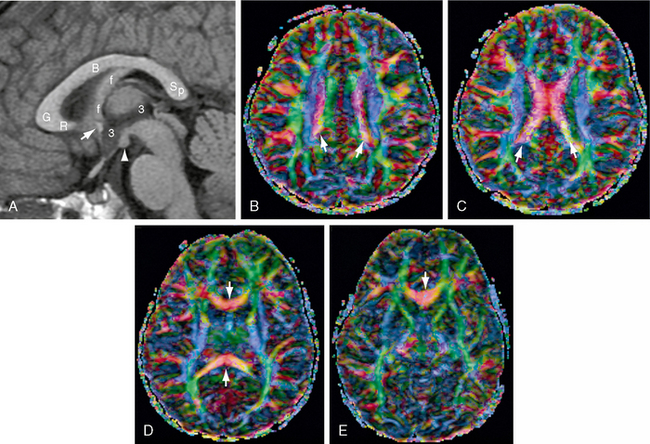
FIGURE 12-8 Corpus callosum. MR images (1.5 T) from a 4-year-old girl. A, Midsagittal T1W MR image for orientation. Because the corpus callosum is curved, axial sections will display the body of the corpus callosum (B) most superiorly, the genu (G) and splenium (Sp) inferior to the body, and the rostrum (R) most inferiorly. The anterior commissure (white arrow) lies in the anterior wall of the third ventricle (3) at the junction of the rostrum with the lamina terminalis. The fornix (f) abuts the inferior aspect of the corpus callosum at the isthmus and then curves downward behind the anterior commissure en route to the mammillary body (white arrowhead). B to E, Diffusion tensor imaging (DTI) color maps. B, As the commissural fibers converge toward the corpus callosum, they form narrow parasagittal bands (white arrows) of inferomedially directed (pink) fibers between the sagittally oriented cingulum bundles (green) medially and the vertically oriented fibers of the corona radiata (blue) laterally. C, The commissural fibers cross the midline in the body of the corpus callosum (red transversely oriented fibers). D, Below the body, the genu and splenium (red fibers, white arrows) extend into more inferior sections. E, The rostrum (red fibers, white arrow) is the lowest portion of the corpus callosum in axial sections.
The commissural fibers cross through the corpus callosum in defined sequence.
Histology
The commissural axons arise from neurons located predominantly in the intermediate cortical layers.18 Callosal fibers that interconnect primary sensorimotor regions are large and heavily myelinated. Callosal fibers that interconnect associative areas are small and poorly myelinated. The highest density of large fibers (3 to 5 μm) is found at the isthmus (for motor, somatosensory, and auditory cortex) and in the posterior splenium (for visual cortex). The highest density of thin fibers (<0.4 μm) is found in the genu and anterior splenium (high-order prefrontal and temporoparietal associative areas). The largest callosal fibers in humans correspond to fibers interconnecting the primary auditory cortex.18
Function
The corpus callosum facilitates interhemispheric interactions for communicating and integrating perceptual, cognitive, learned, and volitional information. It is important for the performance of visual and tactile tasks that require transfer of sensory information between the cerebral hemispheres. Commissural fibers crossing through the anterior portion and body of the corpus callosum are essential to perform temporally independent bimanual finger movements. Commissural fibers passing through the posterior corpus callosum play an important role in visual and visuospatial integration. Callosal fibers are also important for higher-order cognition, including normal social, attentional, and emotional function.6
Imaging
From anterior to posterior, fibers cross through the corpus callosum in the same order that their cortices appear on the cerebral surface. Connections of the prefrontal cortex cross through the genu and anterior part of the body. Premotor connections cross within the midbody of the corpus callosum. Then, in order, come the commissural fibers arising in the primary motor strip (M1), the primary sensory strip (S1), and the posterior parietal cortices. Fibers arising in temporal and occipital cortices cross through the splenium of the corpus callosum. Wahl and colleagues showed that the cortical motor fibers connecting defined body representations of M1 cross through circumscribed, somatotopically organized regions of the corpus callosum.19 Although the callosal fibers cross the midline in a defined order, front to back, they do not appear to be restricted to crossing within specific fractions of the length of the corpus callosum. That is, one cannot say that fibers from any one area consistently cross through, for example, the third sixth of the length of the corpus callosum.20,21
Routine sectional CT and MRI display the corpus callosum well in sagittal and coronal planes, but less well in the axial plane (Fig. 12-8). Diffusion tensor tractography (DTT) displays the fibers arching through the corpus callosum in relation to their points of origin (Fig. 12-9). Thus, DTT can document the precise location through which specific fibers cross in one individual, enabling more precise surgical planning.6,22,23
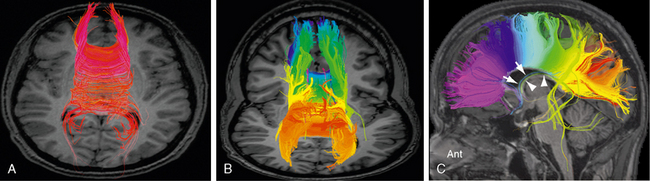
FIGURE 12-9 Corpus callosum. Diffusion tensor MR tractography at 3.0 T. A, The red commissural fibers describe a narrow arc of curvature as they extend to and from the cortical areas related to the midline. B and C, Tractograms of the corpus callosum, color coded from anterior to posterior, display the concordance between the anteroposterior position of the cortical areas of origin and the anteroposterior points at which those fibers cross the midline through the corpus callosum. The precommissural hippocamposeptal portion of the fornix (white arrow, blue fibers) and the retrocommissural hippocampomammillary portion of the fornix (white arrowheads, green fibers) are also displayed. Other stray fibers remain.
The Anterior Commissure
The anterior commissure is a narrow, highly compact fiber bundle that has the shape of bicycle handlebars. Medially, the anterior commissure crosses the midline in the anterior wall of the third ventricle, just rostral to the anterior columns of the fornix (Fig. 12-10; see also Fig. 11-3D).2,24 From there, on each side, the anterior commissure extends laterally through the basal portions of the caudate and putamen, inferior to the anterior border of the globus pallidus, and then through the temporal stem to reach the temporal and occipital lobes.25 Anteriorly, the anterior commissure lies medial to and parallels the course of the uncinate fasciculus.18,26 Posteriorly, the anterior commissure parallels the course of the inferior longitudinal fasciculus.26 A thin anteroinferior portion of the anterior commissure designated the basal telencephalic bundle interconnects non-neocortical (allocortical) portions of the hemispheres including the two olfactory bulbs, the anterior olfactory nuclei, the primary olfactory cortices, and probably the two amygdalae.2,18,26 A larger posterior neocortical limb of the anterior commissure interconnects portions of the orbitofrontal cortices, portions of the insulae, adjoining limbic portions of the temporopolar cortices, and the inferior temporo-occipital cortices.26 Studies of fiber degeneration pathways in humans show that the majority of anterior commissural fibers arise from the inferior temporal cortex and distribute to the contralateral hemisphere both homotopically and heterotopically (to the amygdala and possibly the inferior frontal cortex).25 An additional group of anterior commissural fibers also arises from the inferior and lateral occipital cortices.25 The anterior commissure probably also links the two nuclei accumbens septi.3
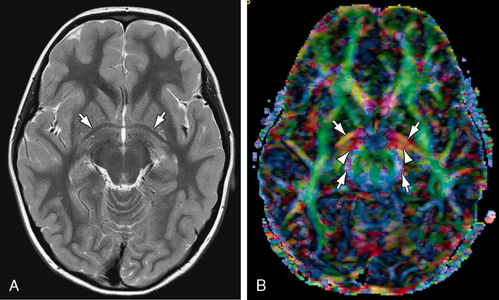
FIGURE 12-10 Anterior commissure. A, Axial T2W MR image. The anterior commissure (white arrows) has the shape of bicycle handlebars (see also Fig. 12-8A). B, Axial DTI. The transversely oriented (predominantly red) fibers of the anterior commissure (white arrows) pass posterolaterally through the lenticular nuclei bilaterally. Part of their course parallels the proximal portions of the optic tracts (also red) (white arrowheads) and anterior contours of the cerebral peduncles (blue) (white arrows).
Histology
The anterior commissure contains approximately 3 million fibers (see Table 12-2).17,18 The single macroscopic tract contains two histologically distinguishable fiber bundles separated by a definite glial plane: (1) a small allocortical basal telencephalic bundle courses along the anterior edge of the gross tract and contains small, mostly unmyelinated fibers; and (2) a large, predominant neocortical bundle courses immediately behind the first and contains mostly small myelinated fibers.18
Function
The fibers of the thin anterior bundle originate from the anterior olfactory nuclei of one olfactory tract and pass across the midline to the contralateral olfactory bulb.17 These fibers may act in reflex control of activity in the olfactory bulb.1 The function of the fibers of the large posterior bundle is not well known.
Imaging
The gross anatomy and standard imaging of the anterior commissure are displayed in Figures 11-14 and 11-15. The greatest diameter of the normal anterior commissure never exceeds 6 mm.18 DTT displays the anterior commissure as arching fibers that pass through the anterior midline toward the inferior frontal regions and toward the temporo-occipital regions (see Figs. 12-10 and 12-11).
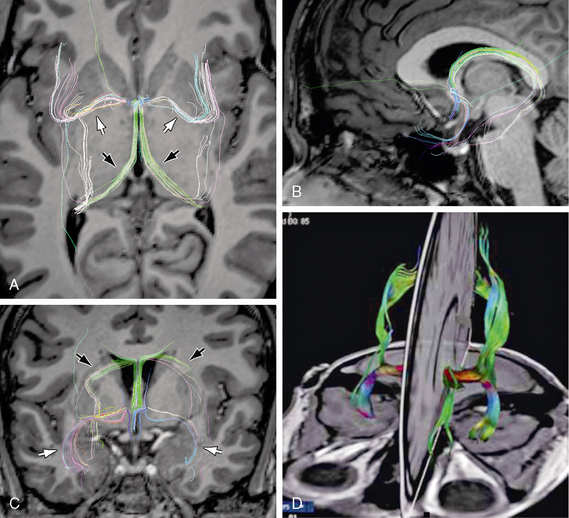
FIGURE 12-11 Anterior commissure. Diffusion tensor MR tractography at 3T. A to C, DT tractograms projected onto the axial plane (A), midsagittal plane (B), and coronal plane (C) show the characteristic shapes and positions of the fibers coursing through the anterior commissure (white arrows) and the fornices (black arrows). D, 3D display of the olfactory and nonolfactory components of the anterior commissure.
(D, Reprinted from Kollias S. Parcellation of the white matter using DTI: insights into the functional connectivity of the brain. Neuroradiol J 2009; 22[Suppl I]:45-57.)
Hippocampal Commissure (Commissure of the Fornices, Psalterium)
Approximately 20% of forniceal fibers cross the midline between the two crura of the fornices to form the hippocampal commissure. Grossly, the hippocampal commissure is a thin triangular sheet of fibers situated between the paired crura of the fornices just dorsal to the posterior third ventricle and just ventral to the posterior body of the corpus callosum (Fig. 12-12). The hippocampal commissure contains fibers that interconnect the two medial temporal lobes in both directions. According to Gloor and associates, the human hippocampal commissure interconnects the presubiculum, entorhinal, and parahippocampal cortices of the two sides but not the hippocampi themselves.18,27
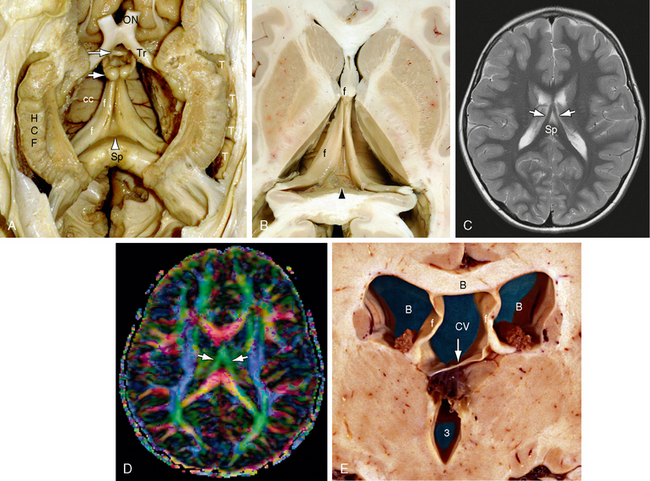
FIGURE 12-12 Fornices and forniceal commissure (hippocampal commissure, psalterium) (dissection by Dr. N. Krayenbühl, Zurich). A and B, Gross dissection of the paired hippocampal formations (HCF) and fornices (f) seen from below. In the triangular region between the two fornices, just anterior to the splenium (Sp) and inferior to the corpus callosum (cc), the commissural fibers of the fornix form a thin sheet of tissue designated the forniceal commissure (hippocampal commissure, psalterium [arrowhead]). Anteriorly, the paired anterior columns of the fornices curve downward toward the anterior commissure (larger white arrow). Most of the forniceal fibers pass behind the anterior commissure to reach the mammillary bodies (small white arrow). A small precommissural contingent passes to the septal region. Also labeled are the optic nerve (ON) and tract (Tr) and the temporal horn (T). C and D, Axial T2W (C) and axial diffusion tensor color map (D) at 1.5 T. The fornices (white arrows) converge anteriorly (green) just inferior to the corpus callosum. The hippocampal commissure lies within the triangle between the fornices and anterior to the splenium (Sp) but is not identifiable individually. E, Cavum vergae. Coronal plane gross anatomic section through the bodies of the lateral ventricles (white B) and third ventricle (3). The walls of the cavum vergae (CV) are formed by the body of the corpus callosum (black B) above, the paired fornices (f, f) laterally, and the commissure of the fornices (arrow) inferiorly. At present, routine anatomic imaging resolves the commissure of the fornices only when it is separated from the undersurface of the corpus callosum.
Histology
In rhesus monkeys, the hippocampal commissure consists mostly of medium-sized myelinated associative fibers and is clearly separated from the splenium of the corpus callosum by a glial plane (see Table 12-2).17,18
Imaging
The hippocampal commissure is difficult to resolve by any standard imaging technique. In most cases it merges indistinguishably into the undersurface of the corpus callosum. When a cavum vergae is present, the cavum separates the hippocampal commissure from the corpus callosum, so the hippocampal commissure may be seen as the lower margin of the cavum, overlying the cistern of the velum interpositum (see Fig. 12-12E). In some cases of schizencephaly with absent septum pellucidum, the hippocampal commissure may also be seen free of superimposition by adjoining structures.
Projection Fibers
Projection fibers convey impulses from the cortex to distant sites or from distant sites to the cortex. The afferent and efferent projection fibers are arranged as radiating bundles of fibers that converge to or diverge from the brain stem. Together they form the corona radiata. The most compact portion of the corona radiata situated just cephalic to the brain stem is the internal capsule.
Corona Radiata
The corona radiata is not a specific tract. Instead it is the name given to the broad fan-shaped array of white matter fibers that appear to converge inferiorly into the internal capsule. It is composed of multiple different fiber tracts (see Fig. 12-2J). The fibers of the corona radiata cross between the transversely oriented commissural fibers that converge to the corpus callosum. The coronal fibers lie between the longitudinal fibers of the cingulum bundle and superior fronto-occipital fasciculus medially and the superior longitudinal fasciculus laterally.3
Function
The fibers of the corona radiata interconnect the cerebral cortex with the thalamus and brainstem in both directions (Figs. 12-13 and 12-14). From anterior to posterior, they include (1) the thalamic connections to the frontal lobes and the frontopontine motor fibers that pass through the anterior limb of the internal capsule; (2) the thalamic connections to the anterior parietal lobe and the corticonuclear motor projections that pass through the genu; and (3) the thalamic connections to the central parietal and occipitotemporal lobes and corticospinal, corticopontine, and corticotegmental motor fibers that pass through the posterior limb of the internal capsule2 (see Internal Capsule, later). The thalamic radiations to and from the cortex are grouped into four thalamic peduncles (Fig. 14-D) (Table 12-3).1
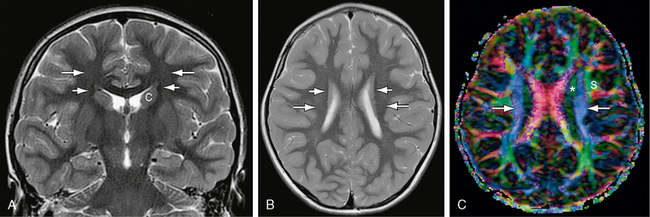
FIGURE 12-13 Corona radiata and superior occipitofrontal fasciculus. A and B, Coronal (A) and axial (B) T2W MR images. The small, compact, longitudinally oriented superior occipitofrontal fasciculus (short white arrows) appears as a thin, low signal ovoid (coronal plane) or line (axial plane) situated just lateral to the superior portion of the caudate nucleus (c) (see Fig. 12-26). The fibers of the corona radiata (long white arrows) lie lateral to this but are difficult to resolve individually on routine sectional imaging. C, Axial plane DT color map. The blue craniocaudally oriented fibers (white arrows) of the coronal radiata are now identifiable medial to the green longitudinally oriented fibers of the superior longitudinal fasciculus (s). The most medial red fibers are the corpus callosum (see Fig. 12-8). The green longitudinally oriented fibers identified by the asterisk could represent a segment of the superior occipitofrontal fasciculus, the more anteriorly directed fibers of the corona radiata (see Fig. 12-14), or a portion of the cingulum bundle sectioned obliquely.
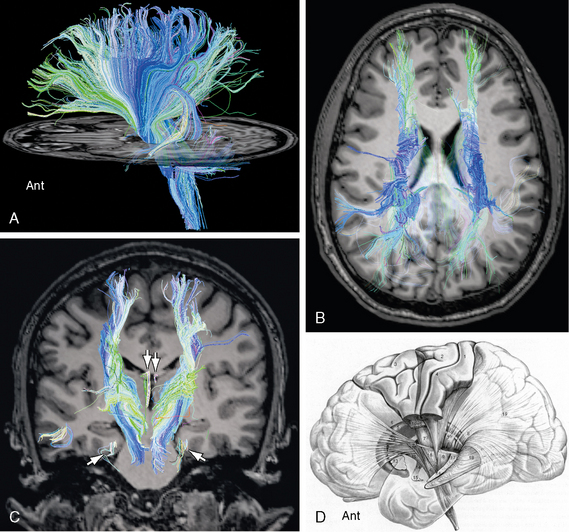
FIGURE 12-14 Diffusion tensor tractography of the corona radiata. Sagittal (A), axial (B) and coronal (C) images display the radiating pattern of fibers for which the corona radiata is named. These fibers converge inferiorly and traverse the internal capsule to reach the thalamus and the brain stem. A few medial forniceal fibers (arrows) are seen to pass to the white matter just deep to the entorhinal cortex of the parahippocampal gyrus bilaterally. D, Thalamic peduncles. Lateral diagram of the thalamic peduncles and fibers within the internal capsule. The corticospinal and corticopontine fibers have been “resected” to expose the thalamic peduncles (see Table 12-3). The anterior thalamic peduncle (12) runs in the anterior limb of the internal capsule. The superior (7) and posterior (8) thalamic peduncles run in the posterior limb of the internal capsule. The inferior thalamic peduncle (14) passes into the ansa peduncularis (15). Other labeled structures include the following: 1, postcentral gyrus (Brodmann areas 2, 1, 3); 2, precentral gyrus (BA 4); 3, frontal gyri (BA 6, 8); 5, caudate nucleus; 6, pyramidal tract; 9, parietopontine tract; 10, corticotegmental fibers; 11, frontopontine tract; 13, putamen; 16, temporopontine tract in the sublenticular portion of the internal capsule; 17, occipitopontine tract in the retrolenticular portion of the internal capsule; 18, optic radiations; and 19, stratum sagittale.
(D, Modified from Nieuwenhuys R, Voogd J, van Huijzen C. The Human Central Nervous System, 4th ed. Berlin, Springer, 2008.)
Imaging
On axial images the corona radiata appears as a nearly uniform region of myelinated white matter (see Figs. 12-13 and 12-14). On diffusion tensor imaging (DTI), the fibers of the corona radiata appear as an open fan of greenish blue mostly craniocaudal fibers that course between the red transversely oriented fibers of the corpus callosum and the green anteroposteriorly oriented long association bundles of the cingulum bundle medially and the superior longitudinal fasciculus laterally.
Internal Capsule
The majority of connections between the cerebral cortex and subcortical structures travel through the internal capsule (Figs. 12-14 and 12-15). Afferent fibers in the internal capsule largely arise from the thalamus and radiate to all parts of the cortex as the thalamocortical radiations.3 Efferent fibers in the internal capsule arise from cortical neurons and extend widely to specific portions of the thalamus, brain stem, and spinal cord as the corticothalamic, corticopontine, corticobulbar (for medulla oblongata), and corticospinal fibers. The fiber tracts passing through the corona radiata and the internal capsule to interconnect the cortex and the thalamus are designated the thalamic peduncles (see Fig. 12-14D and Table 12-3). The anterior, superior, and posterior thalamic peduncles detach from the internal capsule to enter the thalamus dorsally at its rostral and caudal poles.2 The inferior thalamic peduncle enters the thalamus ventromedially, medial to the posterior limb of the internal capsule.2 It forms part of the ansa peduncularis.2
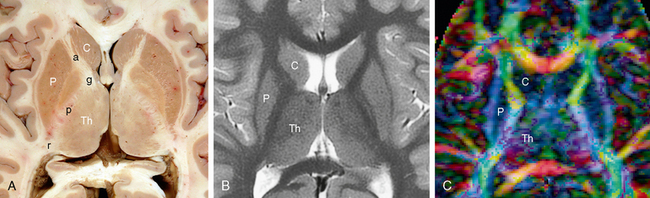
FIGURE 12-15 Internal capsule. Axial anatomic section (A), T2W MRI (B) and DT color map (C). The anterior limb (a), genu (g), posterior limb (p) and retrolenticular (r) portion of the internal capsule course between the caudate nucleus (C) and thalamus (Th) medially and the lenticular nucleus laterally. P, putamen. The more dominant blue coloration in the posterior limb indicates that the fibers passing there are oriented more vertically, while the fibers in the anterior limb, genu and retrolenticular portion of the internal capsule course more obliquely, both vertically and longitudinally.
Function
The topographic organization of tracts within the internal capsule is determined by the anteroposterior position of their cortical connections, regardless of whether they are afferent or efferent fibers (see Figs. 12-2J and 12-14A). The anterior limb carries ascending thalamic projections from the anterior and medial thalamic nuclei to the frontal lobes and descending frontopontine motor fibers (Fig. 12-16).3 The genu contains anterior portions of the ascending superior thalamic (somaesthetic) radiation to the postcentral gyrus and descending corticobulbar motor projections. The posterior limb contains most of the ascending superior thalamic (somaesthetic) radiation to the postcentral gyrus and the descending corticospinal, corticopontine, and corticotegmental motor fibers.3 The retrolenticular portion of the internal capsule conveys the optic radiations from the lateral geniculate nucleus to the calcarine cortex, some of the auditory radiations, and descending parietopontine, occipitopontine, and occipitotectal projections.2,3 The sublenticular portion conveys limited ascending thalamic fibers to the temporal lobe and insula, the auditory radiations from the medial geniculate nucleus to the transverse temporal gyrus of Heschl and the superior temporal gyrus, and descending temporopontine and posterior parietal pontine projections.3
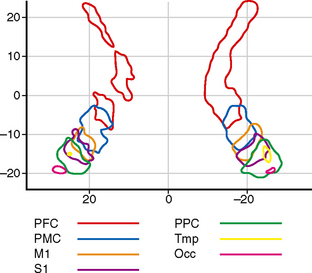
FIGURE 12-16 Internal capsule. Topographical distribution of the cortical tracts within the internal capsule. Two-dimensional probabilistic population map from 11 right-handed subjects 23 to 57 years of age. Grouped data. This diagram is color coded to display the fibers related to the prefrontal cortex (PFC), premotor cortex (PMC), primary motor strip of the precentral gyrus (M1), primary sensory strip of the postcentral gyrus (S1), posterior parietal cortex (PPC), temporal cortex (Tmp), and occipital cortex (Occ). The anterior limb and genu of the internal capsule are occupied by prefrontal fibers. The sensorimotor tracts traverse the posterior half of the posterior limb.
(From Zarei M, Johansen-Berg H, Jenkinson M, et al. Two-dimensional population map of cortical connections in the human internal capsule. J Magn Reson Imaging 2007; 25:48-54.)
Imaging
The gross anatomy and standard imaging of the internal capsule are displayed in Figures 11-3 to 11-12 Figure 11-4 Figure 11-5 Figure 11-6 Figure 11-7 Figure 11-8 Figure 11-9 Figure 11-10 Figure 11-11 Figure 11-12. DTT displays the internal capsule as multiple sets of fibers that course predominantly craniocaudally between the caudate nuclei and thalami medially and the lenticular nuclei laterally (see Figs. 12-14 and 12-15).
Corticospinal Tract
The fibers of the corticospinal tract arise from a broad area of the cortical surface.28 The precise contributions of each cortical area to the tract remain uncertain. According to A. R. Crossman, only 20% to 30% of corticospinal fibers arise from the primary motor cortex (Brodmann area 4).3 These “arise from pyramidal cells in layer V and give rise to the largest diameter corticospinal axons.”3 Forty to 60 percent of pyramidal axons arise from the parietal lobe, including Brodmann areas 3 and 5 of the superior operculum and dorsal insula.3,29 However, according to Ebeling and Reulen, 40% to 60% of corticospinal fibers arise from layer 5 neurons of the primary motor cortex (M1) in the precentral gyrus (Brodmann area 4). About 20% of fibers arise from the somaesthetic cortex of the postcentral gyrus and paracentral lobule (Brodmann areas 3, 2, and 1).28,30 The remainder arise from the dorsal and ventral premotor areas (Brodmann areas 6 lateral and 8), the supplementary motor area (Brodmann area 6 medial), and the parietal lobe (Brodmann areas 5 and 7).28,30 Gender and handedness are not associated with consistent difference in the makeup of the left versus right corticospinal tract.31 A slight asymmetry between left and right corticospinal tracts has been noted in healthy individuals but was not found to be significantly different from zero across individuals.31
The corticospinal tract descends obliquely through the posterior limb of the internal capsule.32–34 If the posterior limb of the internal capsule is divided into four equal portions, then the rostral portion of the corticospinal tract crosses through the first or second quarter of the posterior limb while the caudal portion of the corticospinal tract traverses the third quarter or rarely the fourth quarter of the posterior limb (Figs. 12-17 and 12-18).33 Within the posterior limb of the internal capsule, the fibers of the corticospinal tract are arrayed somatotopically, with individual variation.35,36 Holodny35 and Ino36 found three different arrangements of the corticospinal fibers: (1) the fibers may be arrayed longitudinally from anterior to posterior along the long axis of the posterior limb35,36; (2) they may be arrayed transversely across the short axis of the posterior limb; or (3) they may be intermixed without specific localization. Holodny and associates reported that the fibers were arrayed predominantly transversely, from lateral to medial, with the hand fibers lateral and slightly anterior to the leg fibers in 17 of 20 tracts (85%).35 In 3 of 20 tracts (15%) the fibers were intermixed.35 Ino and colleagues36 confirmed that the corticospinal fibers were arrayed transversely as reported by Holodny and associates35 in four of seven cases (57%). However, the fibers were arrayed longitudinally in the other three cases (43%).36 In one of these three (14%), the hand fibers lay anteromedial to the foot fibers. In two of the three, the hand fibers lay posterolateral to the foot fibers (29%).36
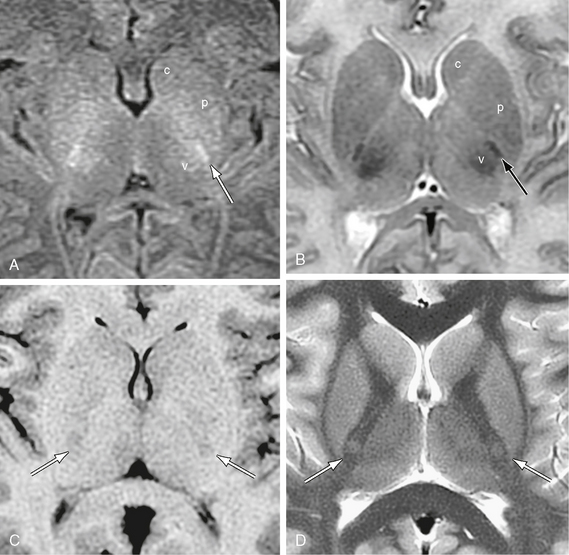
FIGURE 12-17 Corticospinal tracts. Axial MR sections at two ages. The corticospinal tract (arrow) descends through the posterior half of the posterior limb of the internal capsule. A and B, MR images in a newborn girl. Because the corticospinal tract is already myelinated at term, whereas other fiber tracts are not, the corticospinal tract appears as a sharply defined “white” fiber bundle on T1W images (A) and sharply defined “black” bundle on T2W images (B). It characteristically lies just anterolateral to the also-myelinated ventral posterolateral nucleus of the thalamus (v). c, caudate nucleus; p, putamen. C and D, MR images from a 9-year-old girl. With maturation and signal change of the adjacent structures, the corticospinal tract comes to show relatively low signal on T1W images (C) and relatively high signal on T2W (D) in older individuals.
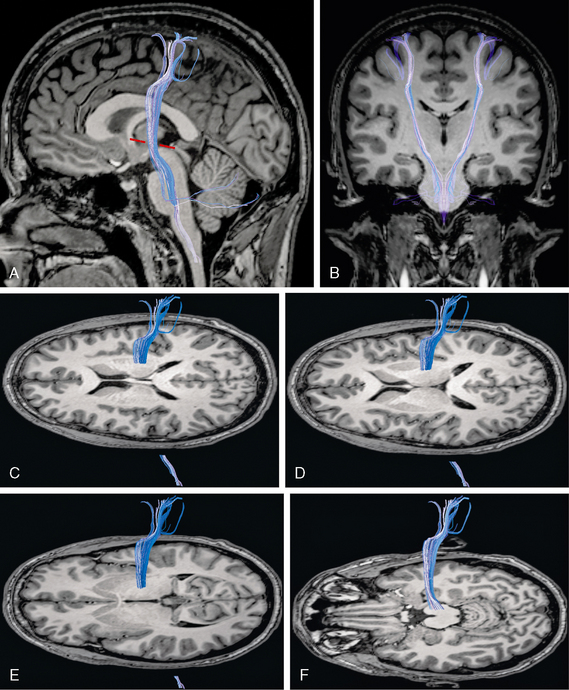
FIGURE 12-18 Diffusion tensor tractography of the corticospinal tracts. A and B, Sagittal (A) and coronal (B) images show the origin of the corticospinal tract (blue) within the precentral and postcentral gyri and its course through the posterior limb of the internal capsule, the cerebral peduncle, and the brain stem. The corticospinal tract characteristically describes an anterior genu within 3 mm of the Talaraich-Tournoux (anterior commissure/posterior commissure) baseline (red dashes). C to F, Projection of the corticospinal tract (blue) onto serial axial MR sections displayed from superior (C) to inferior (F). The corticospinal tract angles from anterosuperior to posteroinferior through the posterior limb of the internal capsule and descends through the midportion of the cerebral peduncle (F) into the lower brain stem.
Newton and colleagues analyzed the intracerebral courses of the contributions to the corticospinal tracts from each of the dorsal premotor area (PMd), the ventral premotor area (PMv), and the supplementary motor area (SMA).37
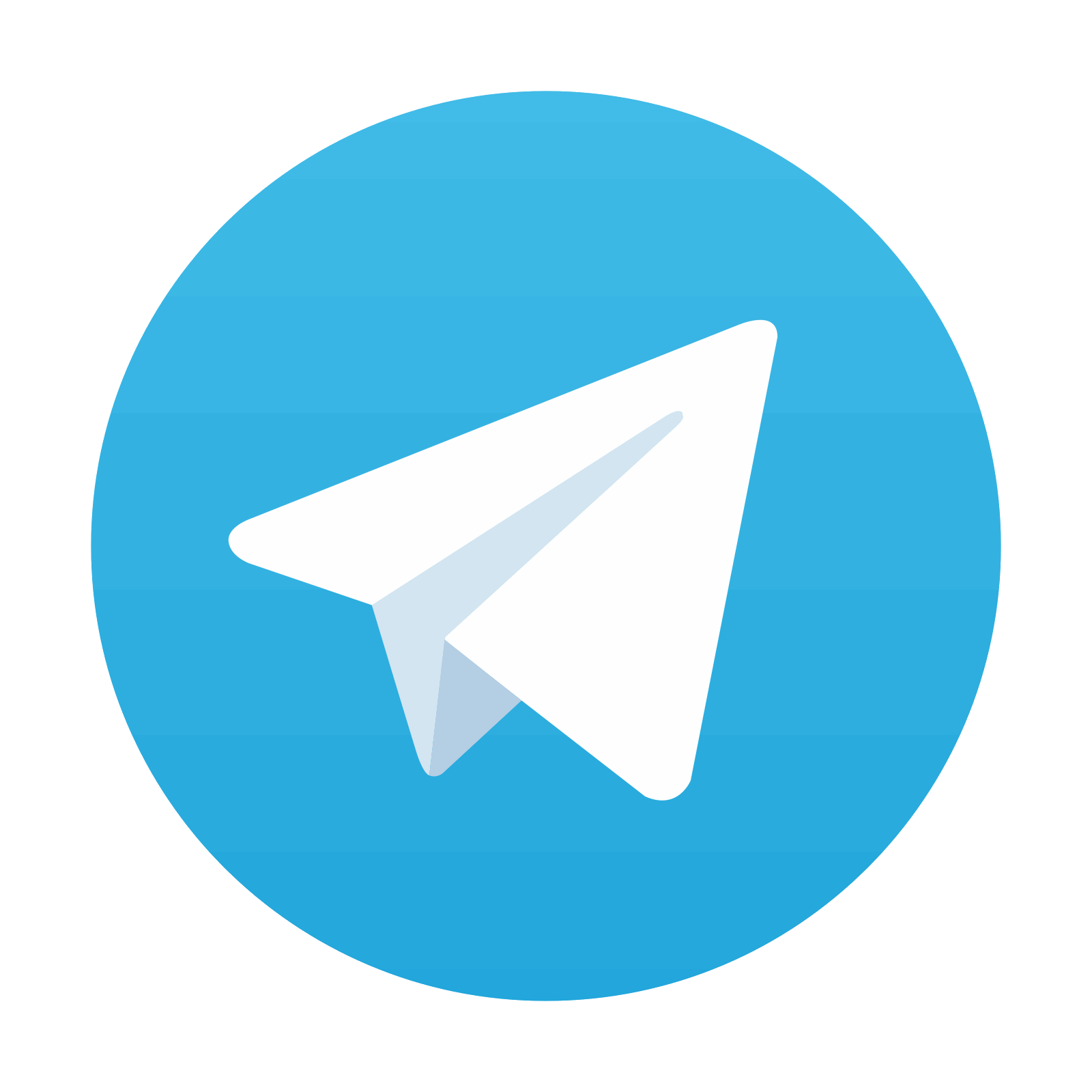
Stay updated, free articles. Join our Telegram channel

Full access? Get Clinical Tree
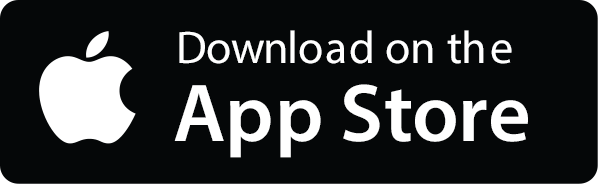
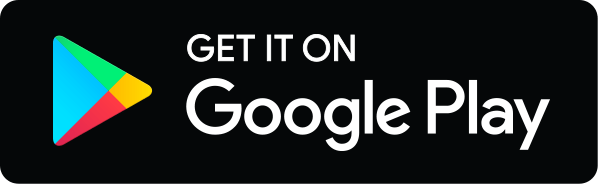