Xe-Enhanced CT Perfusion Measurements
Andrew P. Carlson
Howard Yonas
Introduction and Rationale for Ongoing Relevance of Xe/CT
Xenon-enhanced computed tomography (Xe/CT) is one of the most accurate methods for obtaining quantitative brain cerebral blood flow (CBF) (perfusion) imaging. It is regarded as the gold-standard technique and used to validate other perfusion measurements. Lack of availability of xenon gas in the United States owing to U.S. Food and Drug Administration (FDA) restrictions has inhibited widespread incorporation into clinical practice. With the resurgence of interest in vascular physiology, as well as advancing medical, surgical, and endovascular therapies for ischemia, Xe/CT is poised to re-emerge to fill a much needed gap in providing safe, quantitative data for clinical decision making.
Technique, Acquisition, and Validation
The use of xenon in CBF measurement relates to several intrinsic factors of xenon. Xenon is a noble (inert) gas, which is highly lipophilic, and rapidly crosses into (and out of) brain tissue. This property was initially explored using the (unstable and thus radioactive) xenon-133 isotope and quantitative hemispheric or superficial regional CBF with external scintillation counters. Although this was a powerful technique, it lacked spatial resolution into the deeper drain structures.
The discovery that stable xenon (in nonisotope form) was radio-opaque in the 1970s allowed for the first applications with CT imaging. The molecular similarity of xenon (atomic number 54) to iodine (atomic number 53) accounts for this similarity in radio-opacity; however, the lipophilicity of xenon gas means that it is rapidly absorbed into tissue in a directly flow-dependent manner (rather than staying in the vasculature or regions of blood–brain barrier breakdown, as with iodinated contrast). The use of the technique was initially demonstrated in vivo in defining infarcted territory in baboon,1 which led quickly to a description of the technique in the journal Science.2

Xenon is a rapidly diffusible noble gas with atomic number 54, right next to iodine (atomic number 53) on the periodic table of elements. Iodinated contrast agents are standard for CT imaging and are nondiffusible.
The technique for obtaining quantitative flow information is based on solving the Kety-Schmidt equation, developed in the 1940s.3 This equation was based on the Fick principle—that the amount of a marker substance taken into an organ per unit time is related to the concentration in the arterial and venous systems. Kety and Schmidt demonstrated that by using an inert gas (nitrous oxide initially) and measuring the arterial concentration, the venous concentration, and the blood–brain partition coefficient, global CBF could be calculated. The ability to measure a tracer, in this case xenon, directly in the brain (by serial CT imaging and measuring the change in Hounsfield units) allowed one to substitute for the difference in arterial and venous concentration.
With this technique, baseline CT scans are obtained, and as the patient remains motionless, low-concentration (28% currently) xenon gas is introduced into either a sealed mask or an endotracheal tube circuit during a 4.5-minute inhalation period. As the gas is inhaled, serial CT imaging is performed at the same slices as baseline at around 1-minute intervals. The number of slices is limited only by the number of parallel detectors in the scanner, but usually is four to eight. In addition, the end-tidal xenon concentration is measured by the respiratory circuit, and because of very fast alveolar-arterial equilibration, is a very accurate measure of arterial xenon concentration. This makes arterial catheterization, which is required for other quantitative techniques, unnecessary. Using these values, the modified Kety-Schmidt equation is then solved for each CT pixel in each slice:

Arterial cannulation is not needed for xenon/CT imaging because the end-tidal xenon concentration measured by a mask is a very accurate reflection of the arterial concentration owing to the rapid equilibration of xenon across the alveoli.

Here, Cxebr(t) is the time-dependent brain xenon concentration, λ is the brain–blood partition coefficient, k is the brain uptake flow rate constant, and CxeArt(μ) is the time-dependent arterial xenon concentration.
This process can be performed very quickly with modern computing and leads to multilevel, quantitative CBF data with values calculated for each of the nearly 24,000
pixels in each CT slice. Figure 10.1 shows examples of Hounsfield unit enhancement over time in different brain regions at a single pixel. To overcome the inherent error in single-pixel analysis, the pixels are grouped for analysis into a minimum of 10 × 10 pixels, where the error has been shown to approximate 10%,4 which is comparable to any quantitative physiologic study. The data can then be displayed and manipulated many ways. A standard four-level study is shown in Figure 10.2. The baseline anatomic images are shown with the corresponding CBF map for each level just below. The bottom row displays the confidence based on motion artifact for each level. The scale on the right is a quantitative scale of values for the CBF map in the middle row. The quality of the registration of each series of scans can be assessed for motion, and if a scan falls above a preset tolerance level, that scan can be manually removed for the calculation. See Figure 10.3 for examples. These uniquely rigorous internal validation strategies are performed for every study done, helping the clinician understand the validity of that particular study.
pixels in each CT slice. Figure 10.1 shows examples of Hounsfield unit enhancement over time in different brain regions at a single pixel. To overcome the inherent error in single-pixel analysis, the pixels are grouped for analysis into a minimum of 10 × 10 pixels, where the error has been shown to approximate 10%,4 which is comparable to any quantitative physiologic study. The data can then be displayed and manipulated many ways. A standard four-level study is shown in Figure 10.2. The baseline anatomic images are shown with the corresponding CBF map for each level just below. The bottom row displays the confidence based on motion artifact for each level. The scale on the right is a quantitative scale of values for the CBF map in the middle row. The quality of the registration of each series of scans can be assessed for motion, and if a scan falls above a preset tolerance level, that scan can be manually removed for the calculation. See Figure 10.3 for examples. These uniquely rigorous internal validation strategies are performed for every study done, helping the clinician understand the validity of that particular study.
The data can then be grouped into regions of interest (ROIs), usually 6 or 20 ROIs of the outer 2 cm of cortex, representing mixed cortical CBF. Figure 10.4 shows the baseline anatomic scan on the left for one of the levels
and the CBF map on the right, again with the quantitative scale on the far right. The table below the scan displays the mean mixed cortical CBF for each ROI, as well as the number of individual CBF pixels this is averaged from (area). Inherent differences in acquisition and processing between different scanner manufacturers have been overcome with the use of a CBF phantom, which allows for the xenon system to be used interchangeably with any CT scanner retaining comparable quantitative data.
and the CBF map on the right, again with the quantitative scale on the far right. The table below the scan displays the mean mixed cortical CBF for each ROI, as well as the number of individual CBF pixels this is averaged from (area). Inherent differences in acquisition and processing between different scanner manufacturers have been overcome with the use of a CBF phantom, which allows for the xenon system to be used interchangeably with any CT scanner retaining comparable quantitative data.
Another unique capability of Xe/CT is that the xenon is rapidly cleared (see Fig. 10.5 for an example of the wash-in and wash-out curve), allowing for repeating the test at a very short interval. This allows one to assess hemodynamic reserve with a CO2 or acetazolamide vasodilatory challenge or to assess the effect of an intervention such as blood pressure or CO2 manipulation. The two scans can then be compared side by side and a subtracted image can be used to determine the regions of change between the two studies (see Fig. 10.6).

Xenon is completely cleared within minutes, allowing for early repeated studies to assess response to a vasodilatory challenge or other physiologic manipulation.
Concerns have been raised about potential side effects of xenon inhalation, particularly because at very high concentrations, xenon can be used as an anesthetic agent. At the very low concentrations currently used for physiologic imaging, brief, self-limited respiratory pauses occur in 1.9% of patients.5 None of these required any intervention and
rarely even required termination of the study. Various sensorial side effects have also been reported, which may be distressing to some patients but cease with termination of the study. Severe adverse reactions are very rare (0.35%), and in a carefully monitored series of over 2000 studies, xenon did not result in any permanent sequelae. Furthermore, in the many studies that have been conducted across the world, to our knowledge, no permanent morbidity or mortality has been reported from xenon inhalation for CBF imaging.5,6 That said, because the accuracy of the study critically depends on patient stillness during acquisition, even the minor sensorial side effects can be detrimental to accuracy of the study. For this reason, awake patients should be carefully coached before and during the study to avoid movement.
rarely even required termination of the study. Various sensorial side effects have also been reported, which may be distressing to some patients but cease with termination of the study. Severe adverse reactions are very rare (0.35%), and in a carefully monitored series of over 2000 studies, xenon did not result in any permanent sequelae. Furthermore, in the many studies that have been conducted across the world, to our knowledge, no permanent morbidity or mortality has been reported from xenon inhalation for CBF imaging.5,6 That said, because the accuracy of the study critically depends on patient stillness during acquisition, even the minor sensorial side effects can be detrimental to accuracy of the study. For this reason, awake patients should be carefully coached before and during the study to avoid movement.
The accuracy of the Xe/CT method is unarguable, and it is the most extensively validated CBF/perfusion modality. Various techniques have been used by different centers to confirm the accuracy of the technique. Acute radiolabeled microsphere injection is a destructive CBF technique and has been closely correlated to Xe/CT in baboon across a wide range of CBF rates.7,8 [14C]Iodoantipyrine is another established, destructive CBF method and has also been correlated with Xe/CT in normal and low-flow states in baboon.9 The original Kety-Schmidt method using nitrous oxide has also been compared to the Xe/CT method and was found to be linearly correlated.10 Other noninvasive methods including xenon-13311 and positron emission tomography (PET)12,13 have also been used to further validate the Xe/CT method in humans; however, they are plagued with the lack of an equally accurate gold-standard measure for tomographic CBF. PET is limited to the low-flow CBF range because the H2O15 is not a diffusible tracer. Thermal dilution CBF probes have been shown to have tight linear correlation with regional Xe/CT measurements in several centers around the world.14,15,16 As a further testament to the perceived status of Xe/CT as the gold-standard technique, multiple studies have used it to attempt to validate other techniques. Xe/CT has been used to validate implanted brain tissue oxygenation probes17 or magnetic resonance imaging (MRI) perfusion techniques.18,19,20
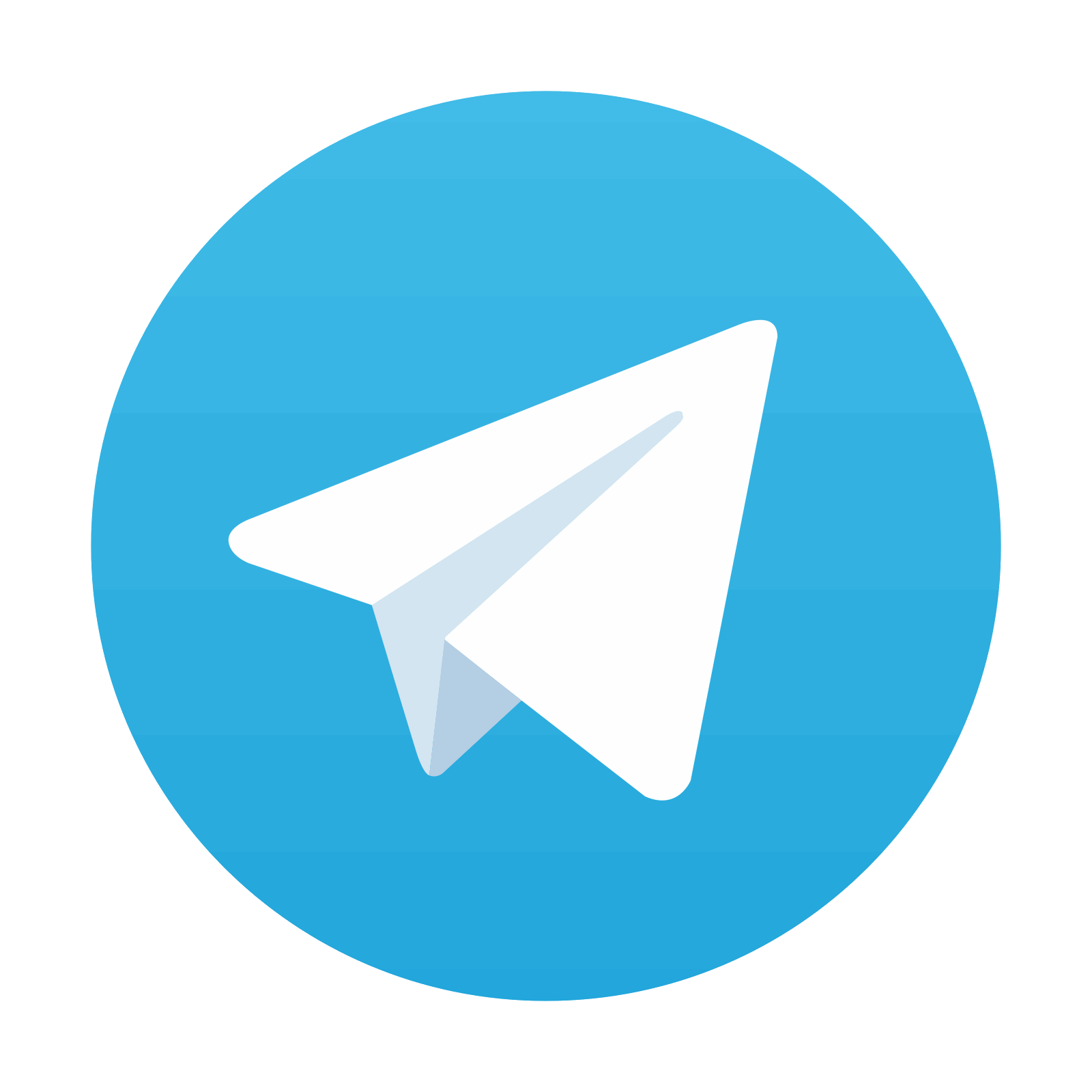
Stay updated, free articles. Join our Telegram channel

Full access? Get Clinical Tree
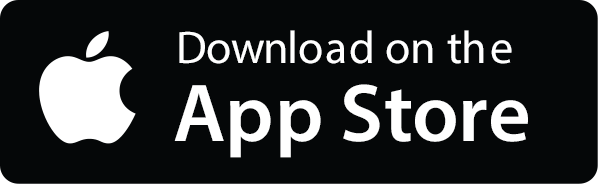
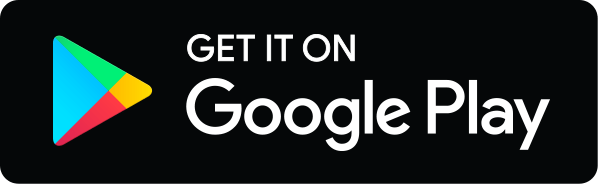