INJURY TO THE AORTA OR GREAT VESSELS
Accidental trauma is the fifth leading cause of death overall and the leading cause of death in children and adults between the ages of 1 and 44 in the USA.1. and 2. Similar figures are reported in Europe. 3 The thorax is a frequent site of significant injury in trauma patients. 3 The overall mortality rate for chest trauma is approximately 15%, but approaches 80% when associated with shock and head injury. 4 Survival frequently depends upon rapid diagnosis and treatment of life-threatening thoracic injuries. Some of the more important thoracic injuries include aortic or great vessel injury, rupture of the trachea, esophagus and diaphragm, pulmonary laceration, and contusion and fractures of the ribs or spine.
The supine anteroposterior (AP) portable chest radiograph remains one of the first tests ordered for acutely traumatized patients. The chest radiograph provides much information about potential thoracic injuries and should be carefully scrutinized for signs of pneumothorax or pneumomediastinum, pleural fluid, pulmonary opacities suggesting contusion, rib and spine fractures, or diaphragm rupture. Identification of correct positioning of endotracheal or orogastric tubes or other cardiovascular support equipment is of equal importance. However, assessment of the mediastinum is perhaps the most critical aspect of interpretation of the chest radiograph in patients with blunt thoracic trauma. Widening of the mediastinum and abnormal mediastinal contours on the chest radiograph are important indicators of mediastinal hemorrhage and, when present, suggest the possibility of aortic or great vessel injury.
Multislice computed tomography (CT) has revolutionized the noninvasive evaluation of thoracic trauma.5. and 6. CT can demonstrate with far greater clarity many of the direct or indirect signs of injury. Tracheobronchial tears can be visualized, aortic injury can either be diagnosed or excluded with confidence, and most diaphragm injuries are well demonstrated by CT. Fractures, contusions, and pneumothoraces are also shown to better advantage. For these reasons, some authors have advocated very liberal or primary use of CT in patients with severe thoracic trauma.4.7.8.9. and 10.
In this chapter, many of the most important manifestations of thoracic injuries are reviewed, emphasizing in particular those due to blunt trauma.
INJURY TO THE AORTA OR GREAT VESSELS (Box 17.1)
Blunt aortic injury
Blunt aortic injury (BAI) is an uncommon, but potentially lethal, complication of thoracic trauma and accounts for 10–20% of deaths in high-speed motor vehicle accidents. 11 Up to 85% of affected patients die at the accident scene; only 10–20% survive the initial injury. 7 Rapid diagnosis of BAI in survivors is critical because, undiagnosed, 30% of affected patients die in the first 6 hours and 40–50% die in the first 24 hours. 7 A small percentage of patients with BAI survive undiagnosed and develop a chronic pseudoaneurysm (Fig. 17.1). 12 The most common causes of BAI are high-speed motor vehicle accidents, falls from significant heights, and airplane crashes. However, BAI may occur after less severe trauma.
Box 17.1
Mechanism of injury
• Rapid deceleration in motor vehicle accidents, plane crashes, and falls
Mechanics of injury
• Shearing and twisting forces concentrated at points of aortic fixation: root, isthmus, and diaphragmatic hiatus
• The ‘osseous pinch’ mechanism
Site of injury
• 95% at the aortic isthmus
• <5% in the ascending aorta
• 1% in the distal descending aorta
• Rarely in the abdominal aorta
Survival statistics
• 85% die at the accident site
• Of the survivors:
– 30% die within 6 hours
– 50% die within 24 hours
– 98% die within 4 months
– 2% long-term survivors
Box 17.2
• Mediastinal widening
– Width >8 cm above the aortic arch on a supine examination with a source-to-detector distance of 100 cm (40 in)
– Mediastinal width/thoracic width >0.25 at this level
• Obscuration of the aortic arch
• Shift of trachea to the right
• Shift of orogastric tube (esophagus) to the right
• Left apical pleural cap
• Widened left paraspinal reflection
• Widened right paraspinal reflection
• Opacification of the aortopulmonary window
• Widened right paratracheal stripe
• Displaced superior vena cava
• Depressed left main bronchus
• Evidence of significant chest trauma
– Multiple rib fractures (especially first rib)
– Lung contusion
– Hemothorax
– Pneumothorax
Box 17.3
Indirect signs of BAI
• Periaortic or perivascular hematoma
Direct signs of BAI
• Focal disruption of the wall
• Extravasation of contrast
• Intimal flap
• Pseudoaneurysm
Diagnosis
• Direct signs more sensitive and specific than indirect signs
• Direct signs have less intraobserver variability
• Negative predictive value >98% (100% for significant injury) when:
– No periaortic hematoma
– No direct signs of injury
Pitfalls in diagnosis
• Streak artifacts
• Thymic tissue simulating hematoma
• Atheromatous disease
• Ductus diverticulum or bump
• Difficulty in identifying injury to the great vessels
Box 17.4
Features on aortography
• Pseudoaneurysm
• Frank rupture with extravasation of contrast
• Intimal tear with intravasation of contrast into the wall
• Posttraumatic coarctation
• Concomitant great vessel injury
Pitfalls in diagnosis
• Ductus bump or diverticulum
• Ulcerated atheromatous plaque
• Preexistent aortic dissection
• Congenital abnormalities of the arch and the great vessels
Box 17.5
Advantages
• Bedside examination
• Rapidly performed
• Highly sensitive and specific with skilled, experienced operator
• Accurate in assessing myocardial and valve function and detecting pericardial effusions
Disadvantages
• Cannot be performed on all patients
• Operator dependent
• Difficult to provide the necessary continuous emergency department coverage
• Does not adequately assess the great vessels and the descending aorta
Box 17.6
Contusions
• Appear rapidly (within a few hours)
• Resolve within a few days
• Opacities do not respect anatomic boundaries (e.g. fissures)
• Peripheral clearing may be noted on CT
Hematoma
• May result from focal laceration
• Solitary or multiple nodules or masses
• Margins initially ill-defined but become well-defined with time
• May cavitate
Posttraumatic lung cysts
• Due to parenchymal laceration
• Solitary or multiple
• May be quite large
• Resolve slowly
• Residual pneumatocele may occur
Secondary injuries
• Pneumothorax
• Hemothorax
The severity of BAI varies from a minimal intimal tear to complete transection of the aorta, which is rarely encountered in living patients for obvious reasons. The three most common sites of BAI are the aortic root, aortic isthmus (site of the ligamentum arteriosum), and diaphragmatic hiatus. Great vessel avulsion is another potential, but uncommon, site of injury. The reported distribution of injuries between these sites varies. In autopsy series, BAI is equally distributed between aortic root and isthmus; injuries at the diaphragm are rare. 13 In surviving patients, however, 95% of BAIs are found at the isthmus (Fig. 17.1), 14 5% in the ascending aorta (Fig. 17.2), and less than 1% in the distal descending thoracic aorta (Fig. 17.3). 15 BAI is rarely reported in the abdomen. 16
BAI is believed to be due to shearing and twisting forces that result from rapid deceleration (Box 17.1) and are concentrated at aortic attachment sites: aortic root, isthmus, and diaphragmatic hiatus. Crass, Cohen and colleagues17. and 18. proposed an alternative mechanism for BAI at the isthmus. They suggested that compression of the anterior chest causes the manubrium to arc posteriorly and inferiorly, the axis of rotation being the spinal attachments of the first ribs. The aortic isthmus is then ‘pinched’ and injured between the manubrium and the spine. 19
Chest radiography
The chest radiograph is often the first imaging test performed in patients with suspected BAI. The chest radiograph is used to screen for mediastinal hemorrhage, an indirect marker for BAI. However, because there are many causes for mediastinal hematoma in seriously injured patients other than BAI (sternal fracture, venous bleeding, spine fractures, etc.) this finding is nonspecific. Most patients (>90%) with mediastinal hematoma on chest radiography will prove not to have BAI on further investigation by CT angiography or conventional aortography.
Mirvis et al. 20 found that an erect AP view of the chest obtained at full inspiration was more valuable for detecting a true-negative mediastinum than a supine view. While such a radiograph performed with pristine technique is clearly most desirable and useful, such views are often difficult to obtain in severely traumatized patients. Thus, many radiographs are obtained with suboptimal technique, so that confident exclusion of mediastinal hematoma, particularly if the patient is severely injured or obese, may be impossible. There is extensive literature documenting the various findings of mediastinal hematoma on chest radiographs, summarized below and in Box 17.2 (Fig. 17.3, Fig. 17.4, Fig. 17.5, Fig. 17.6, Fig. 17.7 and Fig. 17.8).21.22. and 23.
Widening of the mediastinum has been regarded as an important finding of mediastinal hemorrhage after BAI24 (Figs 17.4 and 17.5). However, Ho et al. 25 reported that ‘mediastinal widening’ had a pooled sensitivity of only 73% for BAI and was less accurate than the radiologist’s impression of ‘normal’ versus ‘abnormal’ mediastinum (90% sensitivity). They discouraged use of the term ‘mediastinal widening’, emphasizing its poor sensitivity and substantial interreader variability. 25 For these reasons, many authors emphasize more specific criteria for diagnosis of mediastinal hematoma in the setting of blunt chest trauma. More reproducible criteria for diagnosis of ‘mediastinal widening’ have been difficult to establish, however, due to variations in radiographic technique (source-to-detector distance), patient positioning, patient size, obesity, and age. Proposed criteria have included a mediastinal width exceeding 8 cm just above the aortic arch26 or a ratio of the width of the mediastinum to the width of the chest exceeding 0.25 at the same level. 27 However, BAI is occasionally found in patients with normal mediastinal measurements. 28
Obscuration of the normal contours of the aortic arch and descending aorta and opacification of the AP window are important findings that suggest perivascular mediastinal hematoma and BAI (Figs 17.4 and 17.5). Mirvis et al. 20 found these signs to be among the best radiographic discriminators for BAI. Furthermore, these signs may be more specifically applied to interpretation of trauma chest radiographs.
Displacement of normal structures such as the trachea, left main bronchus, and esophagus are also important radiographic findings of perivascular hematoma. Hematoma at the aortic isthmus can displace the left main bronchus downward (Fig. 17.4) and the trachea and esophagus to the right (Fig. 17.5). Marnocha and Maglinte29 found that depression of the left main bronchus to below 40° off horizontal was a specific finding of BAI. Tracheal deviation is more difficult to diagnose because the trachea normally deviates to the right at the level of the aortic arch, and minor degrees of patient rotation can either artificially accentuate or minimize the finding. Because the esophagus descends adjacent to the right lateral wall of the aorta at the isthmus, lateral deviation of the esophagus (as indicated by the position of an orogastric tube) is a good indicator of perivascular hematoma (Fig. 17.5).30. and 31.
Hemorrhage into anatomically contiguous regions such as the pleural space, paravertebral soft tissues, or apex of the thorax can result in further important radiographic findings that suggest BAI. Hemothorax, particularly on the left, should immediately suggest the possibility of BAI. Widening of the paravertebral reflections (Figs 17.3 and 17.6) 32 also suggests mediastinal hemorrhage. Care must be taken not to confuse paraspinal hematoma due to vertebral fractures with mediastinal hematoma due to BAI (Fig. 17.7). 33 Mirvis et al. 20 found that widening of the left paraspinal reflection (Fig. 17.3) without associated spine fracture was a discriminating radiographic finding for BAI. Mediastinal hematomas may dissect over the lung apices in the extrapleural space, forming so-called apical ‘caps’ which are more common on the left (Fig. 17.4). 34 This finding is particularly important when there are no associated fractures of the upper ribs.
Other radiographic findings such as upper rib or scapular fractures, pulmonary contusion, or pneumothorax can indicate significant chest trauma. 7 The importance of such findings in isolation, particularly upper rib and scapular fractures, and in the context of suspected BAI, is controversial. Most authors have concluded that isolated first rib fractures with an otherwise normal chest radiograph are unlikely to be indicative of BAI.35.36.37.38. and 39.
There is an extensive literature on the role of chest radiography for diagnosis of BAI. While a correctly performed chest radiograph can be a sensitive test for mediastinal hematoma, it is very nonspecific. 40 Mirvis et al., 20 for example, reported that a normal chest radiograph had a 98% negative predictive value for BAI. 20 There are many dissenting opinions, however. Woodring23 reviewed 52 published studies of patients with BAI and found that 7% of patients with proved BAI had normal chest radiographs, and concluded that a normal chest radiograph does not confidently exclude BAI. Fabian et al. 41 reported that 2% of patients with BAI had a normal chest radiograph. Dyer et al. 42 prospectively evaluated over 1500 patients with suspected BAI and found that 4/30 (13%) patients with BAI had normal chest radiographs. Cook et al. 43 and Ho et al. 25 also concluded that the chest radiograph was of limited value in excluding BAI.
There are thus contradictory results regarding the utility of chest radiographs for diagnosing BAI. Many of these results can be explained by inconsistent standards for diagnosis of an ‘abnormal’ mediastinum and a variable ‘gold standard’ for diagnosis of BAI. Many authors, however, conclude that the chest radiograph, properly performed, remains a useful screening test for BAI, but that negative results must be interpreted with caution in patients with a high risk of BAI. 40 Many such patients will, and should, be referred for CT. For example, Exadaktylos et al. 44 have recommended that all patients undergo CT if there is a history of motor vehicle accident at a speed of more than 16 km/h (unrestrained) or 48 km/h (restrained), or a fall from a height of 7 m or more.
Interpretation of a ‘positive’ chest radiograph result remains controversial as well. Algorithms that send all patients with a ‘positive’ chest radiograph to CT may result in considerable overutilization and overexposure of the population to ionizing radiation, with little added benefit. 45 Strategies to decrease the ‘overuse’ of CT in this setting, while maintaining a high sensitivity for diagnosis of BAI, have been proposed and have met with variable success.40.46.47. and 48.
Computed tomography
Many now consider multidetector CT the ‘gold standard’ for diagnosis of BAI42.49. and 50. (see Box 17.3). Current generation multidetector CT scanners allow rapid acquisition of very thin-slice axial images with isotropic voxel sampling through the thorax. These datasets can be reconstructed in multiple two-dimensional planes, and isotropic three-dimensional images that simulate those of conventional aortography can be produced. High-quality CT angiography of the thoracic aorta requires rapid-bolus administration of intravenous contrast (typically between 3 mL and 5 mL per second), thin slice collimation (2.5 mm or less) and overlapping reconstruction intervals. Cardiac-gating can improve visualization of the ascending aorta, 51 but increases acquisition time, a potential limitation for trauma imaging.
The signs of BAI on CT may be classified as indirect or direct. 52 The most important indirect sign of BAI is mediastinal hematoma. As noted previously, however, this finding is relatively nonspecific as it can also be caused by venous bleeding, rib, spine, or sternal fractures. Location of mediastinal hematoma is thus important and has been recently classified into four types depending on proximity to the aorta or other sites of injury such as the spine, preservation of periaortic fat planes, etc. 53 It is generally accepted that only hematoma that directly contacts the margins of the aorta or great vessels (perivascular or periaortic) is of importance for predicting the presence or absence of BAI (see discussion below). The direct signs of BAI include intimal flaps and/or intraluminal thrombus, aortic contour irregularities and/or caliber changes and contrast extravasation11(Figs 17.6, Fig. 17.8, Fig. 17.9 and Fig. 17.10).
Much data support the very high sensitivity of CT for diagnosis of BAI and the very high negative predictive value of a normal study7.42.52.54.55.56.57.58.59.60. and 61. (Figs 17.11 and 17.12). Dyer et al.7. and 42. reported a sensitivity of 95% for BAI and a 99.9% negative predictive value using only direct signs for diagnosis on CT. When they included periaortic hematoma as an additional positive finding, both their sensitivity and negative predictive value improved to 100%. Fabian et al. 56 prospectively evaluated 494 patients with suspected BAI and reported that the sensitivity and negative predictive value of CT was 100% and 100%, respectively. In the same group of patients, the sensitivity and negative predictive value of angiography was 92% and 97%, respectively. Parker et al. 58 prospectively evaluated 142 patients with both CT and angiography and reported 100% sensitivity and 100% negative predictive value for both methods. Ellis and Mayo62 studied 278 consecutive patients who received contrast-enhanced CT for blunt chest trauma and reported a negative predictive value for BAI of 100%.
Several authors have debated the merits of using direct versus indirect signs for diagnosis or exclusion of BAI.52.55. and 59. Fishman et al. 52 found that, when they used direct signs for diagnosis alone, not only did their accuracy of diagnosis improve (with no loss of sensitivity), but the intraobserver variability for diagnosis improved as well. Cleverley et al. 59 found that using direct signs enabled diagnosis of all surgically proved cases of BAI. Interestingly, 9% of patients with BAI in their series had no periaortic hematoma.
There is thus a large body of evidence that suggests that high-quality helical CT angiography has both a very high sensitivity (close to 100%) and a high negative predictive value (also close to 100%) for diagnosis or exclusion of BAI (Table 17.1). Furthermore, evidence suggests that if the criteria for diagnosis of BAI are restricted to the direct signs of aortic injury (intimal flaps, aortic contour irregularities, caliber changes, and pseudoaneurysm formation) or periaortic hematoma, specificity can be substantially improved without an adverse effect on either sensitivity or negative predictive value. In summary, current evidence suggests that patients with a normal-appearing aorta and no periaortic hematoma on CT need not undergo further evaluation. Furthermore, patients with a normal-appearing aorta and only isolated anterior mediastinal hematoma need not undergo further evaluation53 (Fig. 17.12). Dyer et al.,7. and 42. for example, found that no patient with an isolated anterior mediastinal hematoma had BAI.
Clinical suspicion based on severity of trauma and mechanism of injury | Findings on chest radiography | Recommended imaging | |
---|---|---|---|
To detect significant aortic injury | To detect all (including intimal) aortic injuries | ||
Medium–high | Suspect mediastinal hematoma | CT | CT |
Medium–high | Normal | None | CT |
Low | Suspect mediastinal hematoma | CT | CT |
Low | Normal | None | None |
There remains the problem of the equivocal or indeterminate CT. Equivocal results derive from either anatomic variants (‘ductus bump’, bronchial arteries), CT artifacts (motion, stairstep), or, most importantly, perivascular or periaortic hematoma without direct signs of injury. In such cases, aortography is frequently recommended.7.40.42. and 56. While anatomic variants or CT artifacts may exclude definitive interpretation and necessitate aortography, perivascular or periaortic hematoma in the absence of direct signs of injury remains a point of significant controversy. Many authorities40. and 63. continue to recommend aortography in this setting. Sammer et al. 53 reached a different conclusion by reviewing CT, aortography, and outcome data in 107 patients with mediastinal hematoma and no direct signs of BAI on CT, of whom over 50% had periaortic hematoma. In their series, no patients were found to have aortic or great vessel injury.
The further management of patients with evidence of BAI on CT angiography remains controversial. There are several important questions that await definitive answers. The first has to do with the management of a positive result. Bruckner et al. 63 reported a positive predictive value for CT of only 15%. However, CTs that were either interpreted as equivocal or nondiagnostic were included in the positive pool. Of those scans read as definitively positive, the positive predictive value (PPV) was 100%. Further, the majority of their scans were performed on a single slice scanner with 5–7 mm collimation. Nevertheless, as recently as 2006, 63 these authors recommended follow-on aortography to confirm all positive CT results (Fig. 17.8). Ng et al. 11 found that the number and type of direct signs present on CT was an important factor in regard to false positives; in their series, three patients with only a single positive direct sign were found not to have BAI at aortography or surgery. Intimal flaps or intraluminal thrombus had the highest specificity for diagnosis. On the other hand, patients with three or more direct signs, including extravasation, were shown to have a high risk of immediate rupture and death. They would thus recommend proceeding immediately to repair in patients with multiple direct signs or with an intimal flap evident on CT, but proceeding to aortography when only one sign such as luminal irregularity was present. While this decision ultimately rests on the experience of the operating surgeon, a number of other studies support proceeding directly to operative repair based on CT alone.7.42.54.56.58. and 64. Multiplanar reconstruction techniques can yield images that are quite similar to those obtained at conventional angiography; in particular, sagittal reconstructions are usually adequate for surgical planning (Fig. 17.13). 42 Downing et al. 64 reviewed their experience with treatment of 54 patients with BAI and found that operating on the basis of the CT alone was both safe and expeditious. Traditionally, BAI has been treated by open thoracotomy and direct aortic repair. More recently, endovascular stent grafting is being used to treat such injuries.65.66.67.68. and 69. While immediate and ‘mid-term’ results for endovascular repair are promising, the issue has not been definitively resolved.
A further interesting question is the concept of minimal aortic injury (MAI). With the increasing use of high-quality CT angiography (or endoscopic ultrasound), more and more subtle aortic intimal injuries are being diagnosed. Frequently, these injuries are detected in the absence of significant periaortic hematoma (Figs 17.14 and 17.15).7.42.59.70.71. and 72. Malhotra et al. 72 defined MAI as a small (less than 1 cm) intimal flap with no other aortic abnormality and no or minimal periaortic hematoma. In their series of 189 patients with BAI, 10% were found to have MAI by CT. Aortography was normal in half the patients with MAI; in those, the diagnosis was confirmed either by surgery or by endovascular ultrasound. Follow-up studies in six of the nine patients with MAI showed that the lesion resolved in half and that a small pseudoaneurysm developed in the other half. Malhotra et al., 72 and others, 70 have suggested that MAI, in hemodynamically stable patients, can be managed conservatively, but followed carefully until resolution of the lesion is documented. Furthermore, Malhotra et al. 72 found that intravascular ultrasound was useful for confirming such limited injuries.
Aortography
Aortography has long been considered the ‘gold standard’ for diagnosis of BAI (see Box 17.4, Fig. 17.2, Fig. 17.3 and Fig. 17.4, 17.6). Prior to the widespread application of multidetector CT, aortography was performed liberally for evaluation of patients with suspected BAI. Consequently, a large number of negative angiograms were performed; up to 90% of aortograms performed on the basis of an abnormal chest radiograph are negative.20.24.73.74.75. and 76. One of the great advantages of CT is to reduce the number of negative angiograms performed. In centers where high-quality helical CT is available, aortography is more often reserved for patients with either nondiagnostic scans or minimal periaortic hematoma without aortic contour abnormalities. As discussed above, some centers may also perform aortography in patients with clearly abnormal CT scans. With experience, the need for aortography should occur less and less.
The angiographic diagnosis of BAI is occasionally difficult and both false-positive and false-negative examinations (Fig. 17.16) are reported.77. and 78. Angiographic diagnosis depends on identifying a disruption of the aortic contour that ranges from a subtle contour irregularity to a focal pseudoaneurysm and even to evidence of extravasation. The two main pitfalls are ulcerated atheromatous plaque and the ductus diverticulum (ductus bump). Ulcerated plaque can simulate an intimal flap whereas the ductus diverticulum resembles a pseudoaneurysm. Particular care, therefore, needs to be taken in the older individual with other evidence of atheromatous disease. However, this is not a problem with the younger patient and it should be noted that in one large series the mean age of the patients with BAI was 40 years. 79 The appearance of a ductus diverticulum (bump) can be especially problematic. This outpouching of the aorta occurs at the attachment of the ligamentum arteriosum at the isthmus and may closely resemble a pseudoaneurysm. Other diagnostic difficulties include BAI in unusual locations, preexistent nontraumatic aortic dissection and congenital abnormalities of the aorta or great vessels. A detailed review of the problems in the angiographic diagnosis of BAI is beyond the scope of this text, but the subject is well reviewed with copious illustrations by Mirvis et al. 77 and Fisher et al. 80 One potential advantage of aortography over CT and transesophageal echocardiography (TEE) is that it clearly demonstrates injury to the great vessels (see Box 17.5).
Ultrasonography
TEE has shown promise in the diagnosis of BAI (see Box 17.5).81.82.83.84.85.86.87.88.89.90.91. and 92. Smith et al. 93 reported a sensitivity of 100% and a specificity of 98% for TEE in the examination of over 90 patients with suspected aortic injury. Buckmaster et al. 94 reported comparable results. In their series of 160 patients, TEE was 100% sensitive and specific, whereas aortography had a sensitivity of 73% and a specificity of 99%. On the other hand, Saletta et al. 95 and Minard and Lang96 reported more modest results with sensitivities of 63% and 57% and specificities of 84% and 91%, respectively. Vignon and Lang90 reviewed the literature to 1999 and concluded that the sensitivity and specificity of TEE for BAI was 88% (range 57–100%) and 96% (range 84–100%), respectively. In another study, Goarin et al. 87 reported that TEE was more sensitive than either angiography or CT for diagnosis of BAI, primarily because it detected more limited (MAI) intimal injuries. When they compared diagnostic sensitivity for ‘significant’ injuries (those requiring surgical repair), all three methods were equivalent.
There are certain disadvantages with TEE. It may not be possible to perform the examination because of lack of patient cooperation or maxillofacial trauma. 93 TEE is not suited to diagnosing injury to the arch vessels or distal descending aorta.90. and 97. TEE performance is quite dependent on the skill and experience of the operator and it can be difficult to provide continuous TEE coverage with immediate availability by skilled operators. Nevertheless, in experienced hands, TEE seems to be an excellent test for diagnosing BAI. Although it is not commonly used as first-line imaging, it may be quite useful for assessment of patients with indeterminate or inconclusive CT scans or aortograms.88. and 89.
While TEE is clearly an examination of great utility, the role of intravascular ultrasound is less clear. Some authors have reported success in diagnosing BAI with this method.72.88. and 98. This technique is probably too invasive and time consuming to be of value as a primary investigative test. However, like TEE, its greatest value may be in the assessment of patients with indeterminate or inconclusive CT scans or aortograms.
Magnetic resonance imaging
Magnetic resonance imaging (MRI) has not, to date, played a great role in the acute evaluation of suspected BAI.4.99. and 100. There have been, however, considerable recent advances in the quality and speed of high-resolution MRI of the aorta.101. and 102. It is now possible to image the aorta with the same clarity and nearly the same speed as helical CT. However, MRI of an acutely traumatized patient remains a daunting task. MRI is now currently used, and will likely continue to be used, as a problem-solving tool for diagnosis of BAI, once the patient has been stabilized.
Injury to the aortic branch vessels
As has already been noted, injuries to the aortic branch vessels (subclavian, brachiocephalic, and intrathoracic carotid arteries) are less common than injuries to the aorta itself in the setting of blunt thoracic trauma. 103 Branch vessel injury due to penetrating trauma is, for instance, far more common. Chen et al. 104 retrospectively reviewed 166 arteriograms performed in the setting of blunt trauma to exclude aortic or branch vessel injury. They identified 24 vascular injuries overall; 15 had isolated aortic injury, seven had isolated branch vessel injury, and two had both aortic and branch vessel injuries. They emphasized that the arteriographic features of the injuries were often quite subtle. The spectrum of branch vessel injuries reported ranges from limited intimal flaps to marked arterial dissection to complete transection with pseudoaneurysm formation.103.104.105.106.107.108.109.110.111. and 112.
Numerous authors have emphasized the difficulties in diagnosing aortic branch vessel injury.103.105.106.107.108.109.110.111. and 112. Chest radiographs in affected patients are frequently (usually) abnormal, although the sensitivity of the chest radiograph for branch vessel injury has not been studied in detail, perhaps due to the infrequency of these injuries. The role of CT in this regard is even less studied and concerns have been raised regarding the use of CT for diagnosis of branch vessel injury. Certainly, CT can diagnose such injuries (Figs 17.17 and 17.18), but its sensitivity and specificity in the absence of direct signs of vascular injury is unknown. In our practice, patients with significant mediastinal hematoma on CT but who have no direct signs of arterial injury frequently undergo arteriography in order to exclude great vessel injury. Furthermore, arteriography is performed if there are suggestive physical examination findings, such as diminished arm blood pressures or pulses or evidence of neurovascular compromise.
Injury to the pulmonary artery
Injuries to the pulmonary arteries in the setting of blunt trauma are quite rare.113.114.115.116. and 117. Penetrating trauma to the pulmonary arteries is much more common. Blunt injury to the pulmonary arteries is often fatal, as intrapericardial rupture may lead to cardiac tamponade. Chest radiographs in all reported cases have been abnormal. CT has been used successfully to diagnose pulmonary artery laceration in the setting of blunt trauma.113.114.116. and 117. However, given the rarity of the injury, the sensitivity of CT for pulmonary arterial injury in this setting is unknown. Avulsion of pulmonary veins is also a rare, sometimes lethal, complication of blunt thoracic trauma.118. and 119.
INJURY TO THE PULMONARY PARENCHYMA (Box 17.6)
Contusion of the lung parenchyma is quite common in patients with major blunt thoracic trauma and can occur at the site of injury (coup) or in other parts of the lung (contrecoup injury).120. and 121. Contusion results from laceration of the pulmonary parenchyma by sudden compression and shear forces. 122 Lung injury may be further compounded by fractured ribs or tearing of pleural adhesions. Alveolar hemorrhage and parenchymal destruction are usually greatest during the first 24 hours after injury.6.120. and 121. Nevertheless, radiographs obtained within the first few hours of injury may not show findings of contusion. Radiographs obtained shortly thereafter, however, will show either focal or diffuse homogeneous opacities (Figs 17.19 and 17.20). Because the interlobar fissures do not impede the shock wave, lung contusion does not usually localize in a lobar (or segmental) pattern. Although the opacities may appear to progress on chest radiographs for a day or 2, they tend to stabilize and then clear fairly rapidly, usually within 7 days (Fig. 17.19).120. and 121. Lung contusion can result in considerable respiratory distress leading to mechanical ventilation, acute respiratory distress syndrome (ARDS), and, in some cases, long-term respiratory disability.120. and 121.
CT is clearly more sensitive for lung contusion than chest radiography (Fig. 17.21).120.123.124.125. and 126. Schild et al. 127 imaged experimentally induced pulmonary contusions in an animal model with CT and chest radiographs. CT detected 100% of contusions immediately after the trauma, whereas radiographs failed to detect 20% of contusions even on sequential examinations. Miller et al. 126 used CT to quantify the volume of contusion and found a correlation between contusion volume and risk for ARDS. Donnelly et al. 124 reported that lung contusion in children very frequently (95% of cases) showed subpleural sparing on CT, a finding of potential utility for differentiating contusion from infection and aspiration.
Pulmonary laceration can also result in formation of posttraumatic lung cysts (pneumatoceles, Figs 17.21 and 17.22) or focal hematomas (Figs 17.23 and 17.24). 128 Such lesions may be solitary or multiple. Most range from 2 cm to 5 cm in diameter, but extremely large hematomas, up to 14 cm in diameter, are reported. Pneumatoceles or hematomas may be radiographically apparent within a few hours of injury. However, the lesions may not be apparent on initial chest radiographs because of associated parenchymal contusion. In such instances, the lesions will become more visible as the surrounding pulmonary parenchymal contusion clears. As is the case with lung contusion, CT is more sensitive than chest radiography for detection of posttraumatic lung cysts and parenchymal hematomas. Both lesions tend to resolve with time; pneumatoceles tend to resolve faster than hematomas. Hematomas may take many months to resolve completely and for a considerable period of time may be the only visible sequela of previous injury. Since by this stage a hematoma is a circumscribed lung mass, the lesion may be mistaken for a neoplasm (Fig. 17.23). In such a case, Takahashi et al. 129 were able to identify the lesion as a hematoma by its signal characteristics on MRI. During resolution, a hematoma may communicate with the airway and manifest as a cavitary mass (Fig. 17.24). Awareness of these features is important to avoid confusion with more serious pulmonary processes such as lung cancer. Cavitating hematomas resolve without treatment.
![]() |
Fig. 17.22 (Courtesy of Dr. P Goodman, Durham, NC, USA.) |
![]() |
Fig. 17.23 (Courtesy of Dr. P Goodman, Durham, NC, USA.) |
![]() |
Fig. 17.24 (Courtesy of Dr. P Goodman, Durham, NC, USA.) |
Pneumothoraces (Fig. 17.25) and pleural effusions (Fig. 17.19) commonly accompany lung parenchymal injury and may require prompt chest tube drainage. Severely injured patients are usually radiographed in the supine position, which can make detecting air and fluid in the pleural space difficult, unless the fluid or air is localized to the minor fissure, the subpulmonic spaces, or the mediastinal pleural space. In the supine position air tends to collect anteriorly and fluid tends to layer posteriorly. Anterior pneumothoraces can be detected on frontal projections taken with the patient supine, but the findings are subtle and easily overlooked.130. and 131. The mediastinal contours on the affected side may be seen with unusual clarity, and the anterior portion of the diaphragm may be depressed, revealing more of the base of the heart on that side.130. and 132. The lateral costophrenic sulcus may also be seen with unusual clarity and appear asymmetrically deep – the so-called ‘deep sulcus sign’. 132 Pleural effusions may cause a diffuse haze over the lungs resulting from the filtering effect of the posteriorly positioned fluid layer (Fig. 17.19B). If the effusion is large enough, it may extend around the lung and produce a band of density along the lateral chest wall and over the apex. Cross-table lateral views can be helpful for detecting both pneumothoraces and pleural effusions. CT is highly accurate for both, and the lung bases should always be covered during the abdominal CT examination for visceral injury. 133 One study showed that CT detected 100% of pneumothoraces, whereas supine frontal chest radiographs detected only 40%. 134 Two other studies found that supine chest radiographs detected only about 50% of pneumothoraces in trauma patients.135. and 136. However, Holmes et al. 136 also found that many of the pneumothoraces detected only by abdominal CT did not require tube thoracostomy. Wolfman et al. 137 used CT to grade the size of such incidental pneumothoraces and found that very small ones did not require tube thoracostomy.
INJURY TO THE CENTRAL AIRWAYS (Box 17.7)
Tracheal or bronchial rupture most frequently results from penetrating thoracic injuries or instrumentation. 138 Injury due to blunt trauma is rare and, because of the significant force required, is frequently associated with injuries to other structures such as the aorta and great vessels, thoracic cage, and lungs.139.140.141. and 142. The imaging findings of airway rupture are often subtle and may be overshadowed by other injuries. A considerable proportion of cases may go undetected until complications develop either at the site of rupture, such as bronchial stenosis, or in the lung distal to the rupture, such as septic complications or persistent atelectasis.143.144.145. and 146. Definitive diagnosis frequently requires fiberoptic bronchoscopy. 140
Box 17.7
Location
• 80% occur in main bronchi
• Right bronchus > left bronchus
• 15% occur in trachea
• Most occur within 2.5 cm of the carina
Radiographic findings
• Persistent pneumothorax despite adequate chest tube drainage
• Pneumomediastinum
• ‘Fallen lung’ sign (rare)
• Abnormal endotracheal tube position
• Endotracheal tube balloon overinflation
CT findings
• Mediastinal air adjacent to airways
• Airway disruption
• CT ‘fallen lung’ sign
There are two major indirect manifestations of tracheal or bronchial rupture – evidence of air leak and abnormal lung ventilation distal to the injury. Evidence of air leak is the more crucial finding and is seen in up to 90% of cases of tracheobronchial injury. 147 However, in up to 10% of cases, the adventitial sleeve remains intact and air leak may not occur, making the diagnosis of airway injury more difficult. The most common imaging finding of air leak is pneumothorax, seen in 60–100% of cases.148. and 149. Pneumothoraces due to major airway injury are frequently large and persistent despite insertion of multiple pleural tubes (Figs 17.26 and 17.27); they may also be under tension. Pneumomediastinum is another important indicator of airway injury (Figs 17.27 and 17.28)149. and 150. and is a more specific sign than pneumothorax. Pneumomediastinum may be the only sign of air leak in patients with tracheal or intramediastinal bronchial rupture (particularly the left main bronchus). 151 Pneumomediastinum due to airway injury typically manifests on chest radiographs with streaky lucencies around the carina and these extend superiorly as the air dissects in the tissue planes around the trachea, aorta, and great vessels. At the margins of the mediastinum, air dissects and elevates the mediastinal parietal pleura from the aorta and heart. On lateral radiographs, pneumomediastinum is best appreciated in the retrosternal space. Pneumothorax and pneumomediastinum seen on chest radiographs obtained after severe blunt thoracic trauma are highly suggestive of airway injury.
![]() |
Fig. 17.26 (Courtesy of Dr. Jud Gurney, Omaha, NE, USA.) |
The second major indirect manifestation of major airway injury is abnormal ventilation of the affected lung. 152 This can result in ventilation–perfusion mismatch with hypoxia and cyanosis. Loss of bronchial continuity combined with intraairway hemorrhage and edema can also result in significant atelectasis. The diagnostic problems in these cases are numerous. In severely traumatized patients, atelectasis may develop for reasons other than bronchial rupture. Furthermore, significant associated pulmonary abnormalities such as lung contusion or aspiration may also be present. Collapse of a lung is expected in the presence of a pneumothorax, particularly if the pneumothorax is large and under tension. Atelectasis in patients with bronchial rupture is usually persistent and unresponsive to normal therapeutic maneuvers. Bronchial stenosis or occlusion at the site of rupture can occur if the injury is not promptly diagnosed and repaired. Septic complications including pneumonia and abscess can also be encountered in the affected lung.
Further important, but infrequently observed, indirect findings of major airway injury include abnormally positioned endotracheal tubes or overdistended endotracheal tube balloons.153. and 154. Chen et al. 155 reported that balloon overinflation occurred in 71% and balloon herniation through the defect in 29% of cases of tracheal rupture. The overinflated balloon may also assume a more rounded configuration than is normally seen. Tracheal perforation due to traumatic intubation can result in the tip of the endotracheal tube projecting too far to the right of the tracheal air column on supine chest radiographs. 138
A rare but characteristic feature of bronchial rupture is the so-called ‘fallen lung’ sign, which can be seen on chest radiographs or CT139. and 156. (Fig. 17.26). This finding occurs in the setting of a complete bronchial transection and a large pneumothorax that allows the lung to sag away from the hilum inferiorly and laterally and, on supine CT, posteriorly. The vascular pedicle remains intact and the lung remains perfused but underventilated. 157 The consequent ventilation–perfusion mismatch can result in hypoxia and cyanosis.
CT has been used with success to diagnose airway injuries (Figs 17.27 and 17.28).139.155.156.158.159.160.161.162. and 163. CT is clearly more sensitive than chest radiography for detecting small air-leaks in the mediastinum that suggest the presence of airway injury. However, the sensitivity of CT for direct visualization of the site of injury is not 100%. Chen et al. 155 studied a group of patients with tracheal rupture and reported that CT identified the direct site of injury in 71%, whereas, in another study, direct CT evidence of tracheal rupture was seen in only 10% of patients. 160 Thus, the primary role of CT in diagnosis of airway injuries is probably to suggest the possibility of injury when extraluminal air is identified adjacent to major airways. Ultimately, the diagnosis depends on awareness of the possibility of airway injury in cases of severe thoracic trauma. In a considerable number of instances the diagnosis is missed in the acute phase and is detected only because of persistent lung or lobar atelectasis. Bronchoscopy should be performed in any case in which the radiographic or CT findings suggest airway rupture.
INJURY TO THE ESOPHAGUS OR THORACIC DUCT (Box 17.8)
Instrumentation is the most common cause of traumatic esophageal rupture.164. and 165. Most noniatrogenic traumatic esophageal injuries are due to gunshot wounds. 166 Esophageal rupture due to blunt thoracic trauma is quite rare, accounting for only 10% of all noniatrogenic cases.167. and 168. The radiographic features of esophageal rupture are more fully discussed elsewhere (see p. 921) and include pneumomediastinum, pneumothorax, or pleural effusion, most commonly on the left side, and evidence of mediastinitis, including abscess formation. Diagnosis of esophageal rupture in the trauma patient can be quite difficult because these findings may be attributed to other injuries and considerable delay in diagnosis is common.165. and 167. Unfortunately, this delay in diagnosis results in a higher incidence of infectious complications. The majority of esophageal ruptures due to blunt trauma occur in the cervical and upper thoracic region (Fig. 17.29) but distal esophageal rupture following blunt external trauma has been described.169. and 170. In many cases, particularly with gunshot wounds, the diagnosis is made by surgical exploration. Otherwise, an esophagram using water-soluble contrast will readily confirm the injury.
Box 17.8
Mechanism
• Penetrating ≫ blunt trauma
Imaging appearances
• Chylothorax
• Lymphocele
Location
• Left – injuries above T6
• Right – injuries below T6
Diagnosis
• Lymphangiography
• Lymphoscintigraphy
• Percutaneous aspiration
• Magnetic resonance lymphangiography
Injuries to the thoracic duct are usually due either to penetrating trauma or to surgical injury during thoracic exploration (Figs 17.30 and 17.31).171. and 172. Thoracic duct injury from blunt external trauma is exceedingly rare. 173 Rupture of the thoracic duct can result in chylothorax (Fig. 17.30) or, occasionally, a localized lymphocele (Fig. 17.31). 174 Fluid accumulation is characteristically slow and many days may pass before considerable quantities of fluid accumulate. Definitive diagnosis rests on determining that the fluid collection is chylous or that it communicates with the thoracic duct.174.175. and 176. The site of injury to the thoracic duct determines the side on which the fluid accumulates. The duct enters the thorax through the aortic hiatus in the diaphragm and ascends along the right anterolateral aspect of the spine. In the midthoracic region (about the level of the sixth thoracic vertebral body), the duct crosses the midline and ascends along the left anterolateral aspect of the spine. Finally, it arches forward to enter the venous system in the region of the left jugular and subclavian vein junction. As the duct arches into the left cervical region, it is particularly vulnerable to penetrating injury. Worthington et al. 177 described eight cases of rupture involving the upper portion of the thoracic duct above the level of the aortic arch, seven of which were caused by knife wounds. All seven were associated with left chylothorax. On the other hand, injuries to the duct below the level of the sixth thoracic vertebral body usually result in right-sided chylothorax or lymphocele formation. Because the duct lies in close proximity to the spine, there is a significant association between thoracic duct injury and fracture-dislocation injuries of the thoracic spine. 178 Large persistent pleural effusions in patients with such fractures suggest the possibility of thoracic duct injury.
![]() |
Fig. 17.31 (With permission from Perusse KR, McAdams HP, Earls JP, et al. General case of the day. Posttraumatic thoracic lymphocele. RadioGraphics 1994;14:192–195. Copyright Radiological Society of North America.) |
Lymphangiography has been used successfully to determine the site of leakage prior to surgical intervention. Sachs et al. 179 studied 12 patients with chylous ascites or chylothorax following surgery and found abnormal lymphangiograms in seven. Lymphoscintigraphy (Fig. 17.31), 174 CT-guided needle aspiration, 176 and magnetic resonance (MR) Lymphangiography have also been used to successfully diagnose chylothoraces and lymphoceles180. and 181. due to thoracic duct trauma.
INJURY TO THE DIAPHRAGM (Boxes 17.9 and 17.10)
Acute diaphragm rupture can result from either penetrating injury or blunt thoracoabdominal trauma182 (see Box 17.9). Rupture can result in herniation of abdominal contents into the chest, either acutely or in a delayed fashion. Herniated viscera can cause respiratory distress because of heart or lung compression. Herniated bowel can also strangulate, sometimes years after the initial injury. 183
Box 17.9
Mechanism of injury
• Penetrating injury
• Blunt trauma, usually high-speed motor vehicle accidents or fall from height
Incidence
• Seen in 3–5% of patients admitted to major trauma centers
Mortality
• Approximately 20–25%, mainly due to associated injuries
Associated injuries
• Over 90% have solid abdominal organ injury
• 10% have associated blunt aortic injury
• Pelvic fractures, spinal fractures, and closed head injuries common
Diagnostic peritoneal lavage
• Left-sided ruptures – a small number (15–30%) may have a negative lavage (lesser sac sequestration of blood)
• Right-sided ruptures – almost invariably positive
Box 17.10
Chest radiography
• 85–90% show abnormalities of some type
• Pleural fluid ± pulmonary contusion
• ‘Elevated’ hemidiaphragm
• Stomach and bowel loops high in the chest
• Diaphragm obscured by atelectasis and pleural fluid
• Abnormal course of the orogastric tube
CT
• High position of the liver or the stomach in the chest with contralateral shift of the heart
• Diaphragmatic defect may be directly shown – focal or complete discontinuity
• Diaphragm thickening
• Sagittal and coronal reconstructions – diaphragmatic defect.
• Collar or gathering effect on herniated liver or bowel (‘collar’ or ‘waist’ sign)
• ‘Dependent viscera’ sign
• ‘Hump’ and ‘band’ signs – specific to right diaphragm ruptures
Ultrasonography
• Disrupted diaphragm
• ‘Floating’ portion of diaphragm
• Demonstration of liver or bowel herniation
MRI
• Diaphragm shown with clarity – either intact or disrupted
• Herniation of liver, stomach, or bowel clearly shown
Penetrating injuries to the diaphragm are usually caused by knife or bullet wounds (Fig. 17.32). These injuries usually cause shorter diaphragmatic tears than those seen after blunt trauma. 184 Because the tear is usually so small, herniation of abdominal contents is uncommon. Furthermore, because adjacent lung is frequently injured, the contours of the diaphragm may be obscured on the frontal chest radiograph, making detection of small hernias (if present) difficult. Thus, definitive diagnosis may be delayed. For example, Demetriades et al. 185 found herniation of abdominal contents in only 15% of 150 patients with penetrating injuries of the diaphragm. Diagnosis was delayed in almost half of the affected patients. Because associated abdominal injuries that require exploratory laparotomy (e.g. liver, spleen, or bowel laceration) occur in at least 75% of affected patients, diaphragmatic injuries due to penetrating trauma are usually detected by direct inspection. Diaphragm rupture due to blunt thoracoabdominal trauma is usually the result of a high-speed motor vehicle accident or a fall from a considerable height. The high incidence of associated injury is reflected in reported mortality rates of up to 25%.186.187. and 188. Over 80% of patients with traumatic rupture of the diaphragm have concomitant intraabdominal injuries, typically liver and spleen lacerations. 189 Pelvic and spinal fractures are frequent, as is closed head injury. Further, up to 10% of patients with diaphragm rupture due to blunt trauma also have aortic injury190 (Fig. 17.33). Diaphragm rupture due to blunt trauma is more commonly seen on the left, possibly because the liver acts as a buffer on the right. In several large series, 67–88% of ruptures were left sided (Fig. 17.33), 12–28% were right sided (Fig. 17.34) and 5% were either bilateral or central in location.184.187. and 191. While this left-sided predominance may be due to the protective effect of the liver, it may also reflect difficulties in diagnosis of right-sided injuries and that patients with right-sided injuries may have more associated and severe injuries. Autopsy studies of trauma victims who died at the accident site or before hospitalization report an equal frequency of right and left-sided ruptures, 189 in marked contrast to the left-sided predominance noted in surgical series.184.187. and 191. This finding suggests that patients with right-sided ruptures are more likely to die acutely, due to other severe injuries. For example, Boulanger et al. 189 found that 100% of patients with right-sided ruptures had associated intraabdominal injuries whereas only 77% of patients with left-sided rupture had such injuries.
Preoperative diagnosis of diaphragmatic rupture after blunt trauma can be difficult. Diagnosis by chest radiography depends on demonstration of herniated abdominal contents within the thorax (see below). However, herniation of abdominal contents does not always occur in cases of traumatic rupture, particularly if positive-pressure ventilation is used (Fig. 17.34).192. and 193. Prior to widespread use of CT in the setting of trauma, a significant number of diaphragmatic injuries were discovered at exploratory laparotomy.186.187. and 192. Shah et al. 194 reviewed 980 reported cases of traumatic rupture and found that only 44% were diagnosed prior to exploratory surgery. Over 40% of the ruptures were diagnosed during surgery or at autopsy and, in 15% of cases, the injury was not diagnosed during the initial hospitalization. Beal and McKennan, 195
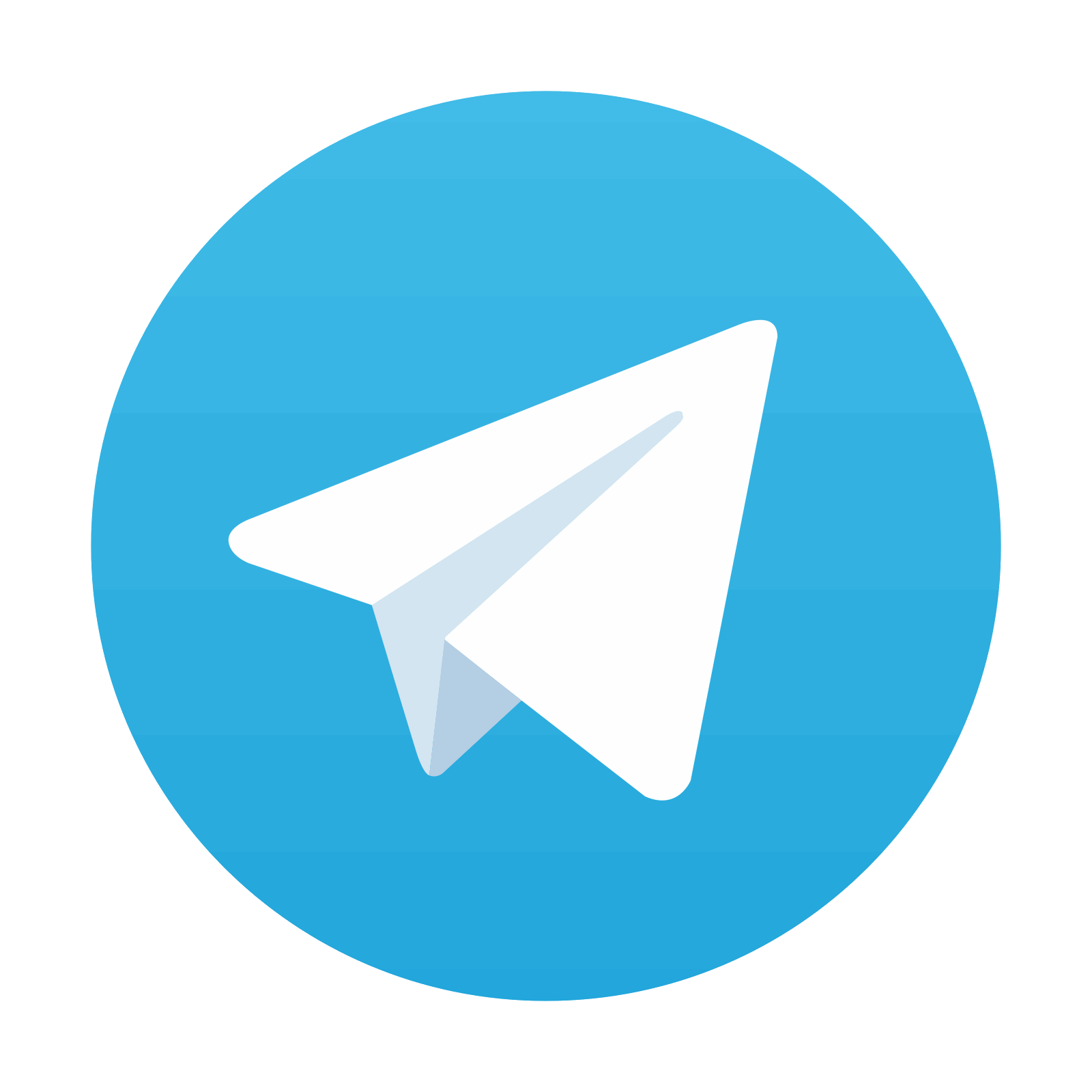
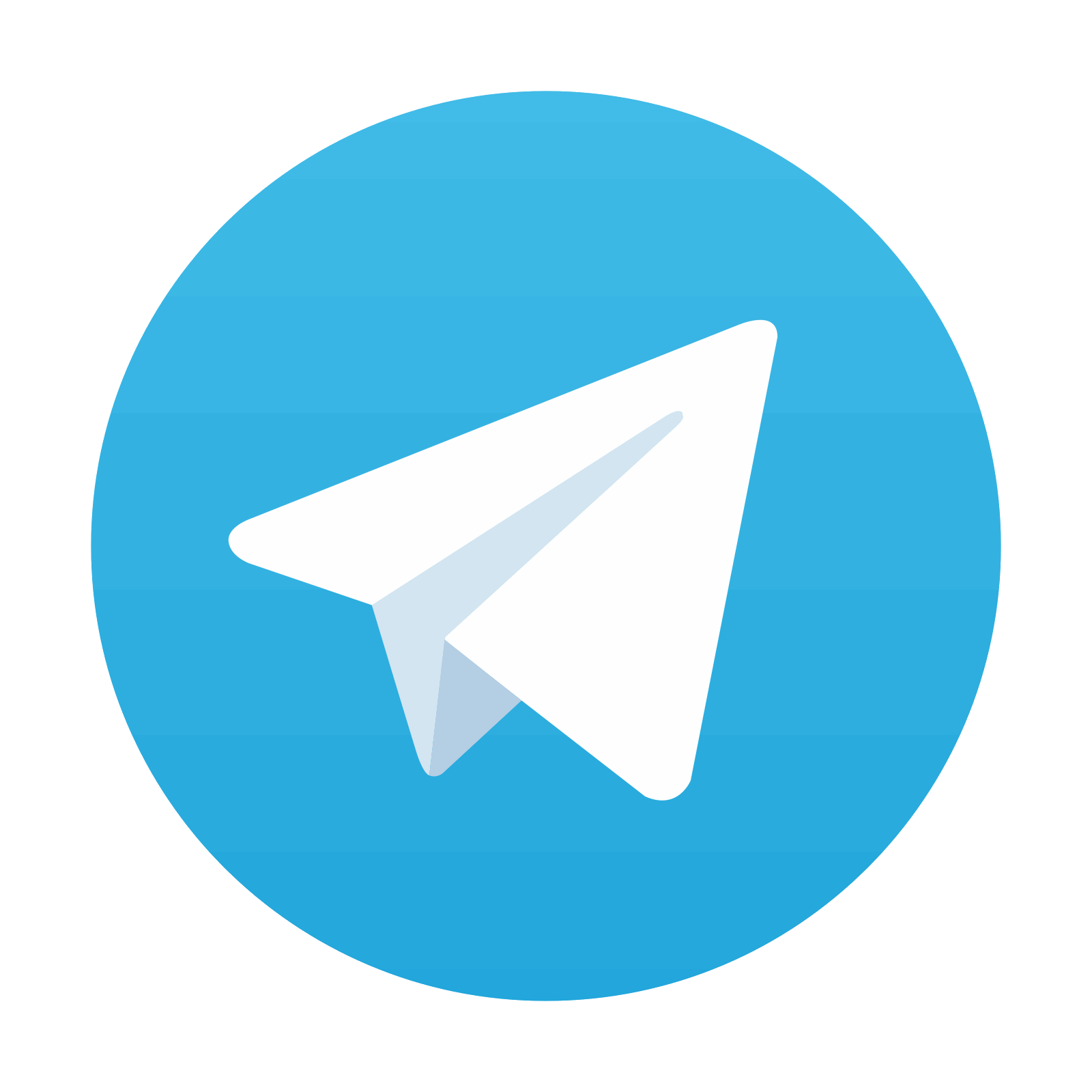
Stay updated, free articles. Join our Telegram channel

Full access? Get Clinical Tree
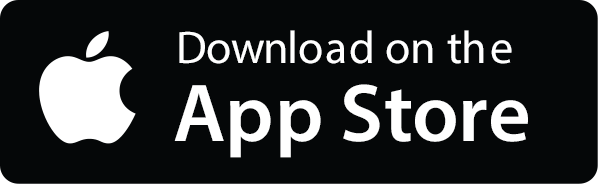
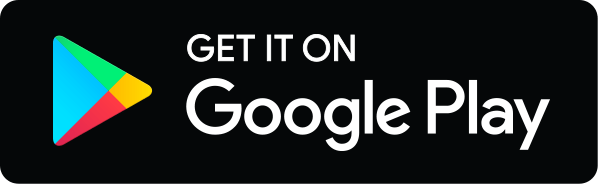