Fig. 22.1
The path from genes to behavior. fMRI phenotypes are closer to genes effect compared to behavioral correlates
In this chapter, we will first focus on how high-field fMRI is helping in revealing pathophysiological aspects of a prototypical brain disorder, i.e., schizophrenia. In particular, we will first describe key concepts related to this brain disorder and to its genetics. Then, we will describe intermediate phenotypes for schizophrenia as identified with fMRI. Furthermore, we will focus on studies addressing the relationship between such intermediate phenotypes and genetic variations of relevance to schizophrenia. Finally, we will highlight recent applications of high-field functional neuroimaging as a tool for delineating trajectories of risk for this brain disorder using the intermediate phenotype approach.
22.2 Schizophrenia: Key Concepts
Schizophrenia has a lifetime prevalence of approximately 0.3–0.7 % (Diagnostic and Statistical Manual of Mental Disorders, version V (DSM V) [3]) and is considered the most severe and disabling mental disorder for several reasons. First, schizophrenia symptoms including delusions, hallucinations, and flattened affect lead to a significant decline in personal and social functioning of the patients [136]. Moreover, it is characterized by a chronic course with frequent relapses [59], and the rehabilitation of patients suffering from this disorder is hard because of the frequent insufficient response to the pharmacological treatment, which often fails in improving personal and social functioning of patients.
Heritability plays a crucial role in schizophrenia: about 80 % of the susceptibility for this disorder is explained by genetic factors [94]. Nonetheless, the likely complex, heterogeneous, and non-Mendelian genetic architecture [69] of schizophrenia makes it extremely difficult to discover causative genes. There is not a single gene explaining genetic risk for schizophrenia [12]. In this regard, genome-wide association studies (GWAS) using very large sample sizes suggest that risk for this brain disorder is associated with several genetic variants, each with a small effect [113]. In particular, the Schizophrenia Working Group of the Psychiatric Genomics Consortium analyzed genome-wide data from more than 36,000 patients with schizophrenia as well as more than 113,000 healthy subjects and identified 108 genetic loci associated with diagnosis for this brain disorder [113]. These results are giving an outstanding contribution and impulse to further studies investigating the biological pathways implicated in mechanisms of risk for this devastating disease, which are not fully understood to date. Importantly, gene-environment interactions may be relevant in increasing such risk [12, 36]. This scenario, together with the uncertainty and variability of the clinical phenotype [12], makes it crucial for the investigation of intermediate phenotypes characterized by fMRI in order to link small and complex gene effects to measurable biological correlates, such that new light could be shed on key pathophysiological aspects of schizophrenia.
22.2.1 Schizophrenia and fMRI Intermediate Phenotypes
A starting point of fMRI studies in schizophrenia is that patients exhibit deficits while performing tasks eliciting high-order cognitive functions as working memory and attention [47, 77, 145]. Moreover, evidence revealed that such cognitive deficits are also present in their unaffected siblings [47, 137]. On this basis, past research devoted to the investigating of neurobiological correlates of these cognitive impairments identified abnormal activation of the dorsolateral prefrontal cortex (DLPFC) as a reliable and robust intermediate phenotype for schizophrenia (Bertolino and Blasi in Pancheri, [11]). Indeed, this brain region is key for working memory [62] and attentional control [17] processing. More in detail, a large body of literature suggested decreased prefrontal activation in patients with schizophrenia when compared to healthy controls during working memory (hypofrontality) [6, 103, 104, 112]. On the other hand, further studies proposed a more elaborated interpretation of the relationship between schizophrenia and dorsolateral prefrontal abnormalities, positing that prefrontal capacity in patients with schizophrenia reaches saturation at a lower level of working memory demand when patients are compared to normal controls [13, 32, 91, 118, 130, 132]. Thus, patients may have greater DLPFC activity than controls at low working memory demands because of the need to recruit a large amount of prefrontal resources. At a greater load of working memory, patients may have lower DLPFC response, because of the failure of performing the task when the load is too high for their working memory capacity [91].
Similarly, studies focusing on attentional control and selective attention suggest a decrease in activation in patients with schizophrenia in DLPFC [6, 22, 34, 35, 78, 80, 87, 107, 108, 119, 149] and in cingulate cortex [17, 18]. On the other hand, there is evidence of hyperfrontality in patients with schizophrenia when compared to controls while performing attentional control tasks [78, 92, 148]. Again, an earlier saturation of capacity also during attentional processing may reconcile these apparently discrepant findings [22]. Apart of these interpretations, these studies testify the strong involvement of DLPFC in the pathophysiology of schizophrenia, suggesting that abnormalities in functional activation during both working memory and attentional control tasks are core features of this disorder.
As stated above, one of the key features of intermediate phenotypes is that they must be present also in healthy relatives of patients suffering from the disease. In this line of reasoning, further studies have investigated whether healthy siblings of patients with schizophrenia share with their affected relatives patterns of functional abnormalities during brain processing. For example, Callicott et al. [30] demonstrated that, despite the absence of significant behavioral differences between groups, siblings had greater DLPFC activation than normal controls while performing the N-Back task, suggesting that siblings require the engagement of a greater amount of neuronal resources compared to controls in order to perform the working memory task at the same level of proficiency. This pattern is similar to those found in several previous studies ([24, 29, 45, 67, 78, 90]; Manoach et al. 1999; [92, 122, 126, 134, 148]) and consistent with the model of decreased prefrontal capacity during cognition in schizophrenia described above [91].
Recent studies on schizophrenia have also focused on another key brain function, i.e., the processing of reward. This is a form of action-outcome learning about an organized behavior that is performed in order to obtain a positive experience [154]. Indeed, previous models have posited that reward processing may play a key role in the pathophysiology of schizophrenia [61], possibly because of the inability of patients in integrating feedback over extended learning. In this context, several fMRI studies have demonstrated abnormal lower activation of the ventral striatum in patients with schizophrenia during reward anticipation compared to healthy controls, as well as a negative correlation between such striatal response and negative symptoms scores [41, 50, 75, 76, 97, 124, 156]. Consistent with a role of the neural network subtending reward in the pathophysiology of schizophrenia, a recent study using a multivariate approach [84] found that diagnosis of schizophrenia is predicted by the activation pattern of several key nodes of the reward system (frontal, temporal, and midbrain regions) (93 % accuracy rate). Activation in ventral striatum was associated with 88 % accuracy rate in the prediction analysis. Moreover, activity of ventral striatum also predicted negative symptoms scores. These results suggest that investigation of the brain network subserving reward processing is a promising target for the identification of novel neurobiological markers of schizophrenia. Accordingly, few recent studies comparing the brain activation of normal controls and relatives of patients with schizophrenia revealed that relatives have the same pattern of reduced ventral striatum activation characterizing patients [44, 66] and that such ventral striatal activation negatively correlates with subclinical negative symptoms [44].
Overall, these findings suggest that region-specific fMRI activity during different brain processes is crucially associated with schizophrenia. In particular, DLPFC fMRI response during high-order cognitive processing is consistently altered in patients as well as in their healthy siblings. Consistent findings have also highlighted the putative relevance of ventral striatal activity during reward processing for schizophrenia. This evidence strongly suggests that these fMRI correlates could be useful intermediate phenotypes for this brain disorder.
A growing body of evidence obtained using high-field MRI is also highlighting the crucial relevance for schizophrenia of anomalies in functional connectivity between key regions for working memory and attention. In this context, evidence indicated lower cortical (prefrontal) and subcortical (especially thalamic) connectivity during cognitive processing in patients with schizophrenia and in subjects with genetic liability for this disorder [42, 105, 140, 141, 155], although evidence of increased prefrontal connectivity has also been found [152]. Moreover, other studies have revealed that connectivity abnormalities related to schizophrenia are also present in regions not directly participating to cognitive processing, such as nodes of the default mode network (DMN) [109, 127], which is composed of a set of brain areas including the medial prefrontal cortex (mPFC), the posterior cingulate cortex, the inferior parietal lobule, the precuneus, and the medial temporal lobe [117]. This network has been implicated in self-reference [68] and introspection [110]. In particular, abnormalities in DMN connectivity have been found in both patients with schizophrenia and people at genetic risk for the disease, even if patterns of anomalies are not always consistent [38, 58, 82, 99, 101, 116, 133, 152, 155]. A recent study [5] investigated with Independent Component Analysis the relationship between genetic risk for schizophrenia and connectivity strength in both DMN and nodes of cognition during attentional control using the Variable Attentional Control (VAC) task [18] in patients with schizophrenia, unaffected siblings of patients, and normal controls. Results revealed that patients with schizophrenia and healthy siblings, compared to healthy controls, had attenuated connectivity strength in left thalamus within an attentional control network (Fig. 22.2) as well as greater connectivity in right medial prefrontal cortex (PFC) within the DMN.
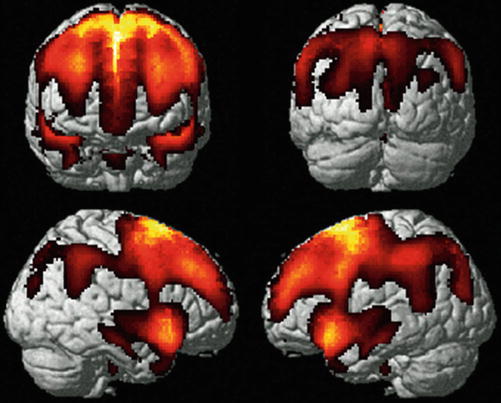
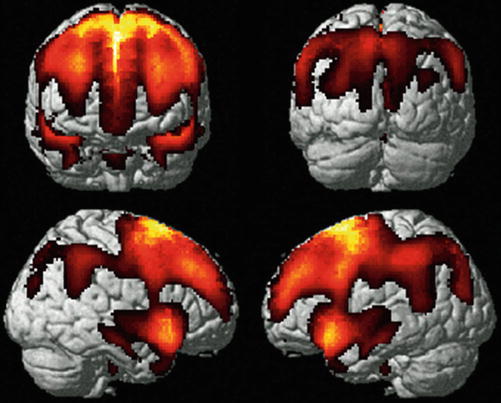
Fig. 22.2
Render depicting an independent component of brain functional connectivity correlated with attentional control processing, as found in Antonucci et al. [5]
These results are in line with studies demonstrating the key role of the thalamus in cognition [71, 139, 163] as well as with those indicating its abnormal activation in patients with schizophrenia and in their healthy siblings during cognitive processing (Braus et al. 2002; [70, 88, 90]; Salgado-Pineda et al. 2004). Furthermore, they are also consistent with the hypothesis that connectivity dysfunctions are a core feature of schizophrenia, especially in the thalamic-prefrontal network [4, 102, 123, 150], such that they could be considered a reliable intermediate phenotype for schizophrenia.
22.3 The Relationship Between fMRI Intermediate Phenotypes and Genetics of Schizophrenia
It is crucial to link intermediate phenotypes with biological pathogenic mechanisms of the disease. Shaping this relationship is relevant in order to shed light on the effects of such mechanisms on the physiology of the brain, as well as to unveil how pharmacological treatments modulating specific biological targets affect system-level phenotypes key for brain disorders. With respect to schizophrenia, a large body of evidence suggests that risk for this brain disorder is predicted by multiple genetic variants [113], that dopamine plays a key role in its pathophysiology ([14, 16, 26, 33, 65]; Weinberger 1987), and that genetic variability of an important determinant of dopamine signaling as the D2 dopamine receptor (DRD2) is associated with diagnosis of schizophrenia [113]. Importantly, D2 is target of all the antipsychotics to date and modulates prefrontal cognition (Seamans 2004 [121]). Its relevance in psychotic disorders is further testified by evidence of increased D2 density in striatum in patients [85]. Furthermore, both clinical and cognitive symptoms in patients with schizophrenia may be associated with abnormalities in D2 signaling [46, 81, 144]. Thus, it is crucial to investigate the relationship between genetic variations affecting D2 signaling and intermediate phenotypes as identified with high-field fMRI in order to add knowledge to the relationship between genes effect and brain correlates of relevance to schizophrenia.
In this regard, it is important to note that there are two alternatively spliced isoforms of the D2 receptor [138]: the D2 long (D2L) isoform is primarily postsynaptic, while the D2 short (D2S) isoform is mainly presynaptic and acts as an autoreceptor. Previous evidence has indicated that an intronic DRD2 single-nucleotide polymorphism (SNP) (rs1076560) affects the D2S/D2L ratio in both prefrontal and striatal regions, with GG subjects having higher D2S density than subjects carrying the T allele [162]. Importantly, this SNP also predicts in healthy subjects behavioral performance as well as cortical and subcortical activity during working memory and attention, with subjects homozygous for the guanine (G) allele being more efficient than subjects carrying the timine (T) allele (i.e., GG subjects exhibited lower prefrontal and striatal activation and greater performance during working memory when compared to T carriers). Other findings indicate that this genetic variant interacts with diagnosis of schizophrenia in modulating cognitive and neurobiological phenotypes. In particular, Bertolino et al. [15] replicated the association of rs1076560 with D2S/D2L ratio of expression in healthy subjects and found similar results in patients with schizophrenia. Furthermore, they reported an interaction between genotype and diagnosis on prefrontal activity and behavior during working memory. In particular, T carrier healthy subjects had lower working memory performance in the face of greater prefronto-striatal activation when compared to healthy GG subjects. Differently, patients carrying the T allele had lower working memory accuracy but also lower prefronto-striatal activity relative to GG patients. The authors interpreted these results on the basis of a differential dopamine level in patients with schizophrenia and healthy subjects, such that suboptimal prefrontal processing during working memory might be differentially elicited in this group of individuals. Apart of possible interpretations, overall these findings strongly suggest a key relevance of functional variation in the D2 receptor gene in the modulation of crucial system-level phenotypes of schizophrenia, as measured with high-field fMRI.
D2 signaling is transducted in the neuron by different signaling pathways, which allow D2 related molecular adjustments possibly relevant for pathophysiological aspects of schizophrenia. In one of these molecular cascades, D2 receptors interact with the serine/threonine kinase AKT1, which phosphorylates to inhibit the protein kinase glycogen synthase kinase (GSK-3β) in a cAMP-independent pathway [51]. In particular, D2 stimulation by dopamine inhibits AKT1 through dephosphorylation [8, 9]. This mechanism in turn modulates activity of another serine/threonine kinase, GSK-3β, which has beta-catenin as a crucial substrate involved in gene expression [19, 20, 51]. Importantly, both AKT1 and GSK-3β have been involved in schizophrenia. For example, AKT1 levels in peripheral lymphocytes and in the prefrontal cortex are reduced in schizophrenia patients [49]. Furthermore, the AKT1 coding gene (14q32.32) has been associated with diagnosis of schizophrenia [73, 98, 120, 131, 135]. Moreover, imaging genetic results revealed that the A allele of a synonymous SNP in the gene coding for AKT1 (rs1130233, G>A) has been associated with less efficient prefrontal activity during working memory. With regard to GSK-3β, evidence indicated lower phosphorylation of this kinase in postmortem prefrontal cortex of patients with schizophrenia [2, 49] as well as genetic association of GSK-3β with diagnosis [86, 128]. Other studies have also indicated that GSK-3β genetic variation affects temporal lobe volumes in schizophrenia patients [10]. Moreover, a recent fMRI study [19] tested the association of a polymorphism (rs12630592) in GSK-3β coding gene with prefrontal activity during cognitive processing and prefrontal cortical thickness in healthy humans. Results demonstrated that the TT genotype for rs12630592 was associated with attenuated fMRI prefrontal activity during working memory and attentional control processing. Furthermore, TT genotype was associated with reduced prefrontal cortical thickness and with diagnosis of schizophrenia.
Given the complex genetic architecture of schizophrenia, other studies have also tested if the interaction between genetic variants relevant to D2 signaling affect brain phenotypes crucially associated with this brain disorder. In this context, a recent study [20] investigated the interaction between DRD2 rs1076560 and AKT1 rs1130233 on multilevel correlates in healthy subjects and patients with schizophrenia. Results indicated that the interaction between the T allele of DRD2 rs1076560 and the A allele of AKT1 rs1130233 was associated with reduced AKT1 levels, reduced GSK-3β phosphorylation and altered cingulate cortex activation during attentional control. Furthermore, in a sample of patients with schizophrenia, the authors found that the interaction between the two alleles was associated with better response after 8 weeks of treatment with a second-generation antipsychotic. Moreover, Blasi et al. [21] investigated the interaction between functional genetic variations in genes coding for D2 (rs1076560) and 5HT2A (rs6314) [19] coding genes, whose signaling likely converges on common molecular pathways [43]. Here [21] the authors demonstrated that healthy subjects carrying the T allele for both genetic variants have greater prefrontal activity during working memory and attentional control tasks, as well as lower behavioral accuracy during N-Back. Furthermore, patients carrying the T allele for both DRD2 rs1076560 and HTR2A rs6314 had lower clinical improvements after antipsychotic treatment, relative to the other genotypic groups. Other results add evidence to the complex interaction between genetic variants in modulating intermediate phenotypes for schizophrenia. In particular, Tan et al. [131] found an epistatic interaction between AKT1 rs1130233 and COMT rs4680, a functional polymorphism located in the gene coding for the cathecol-O-methyltransferase, which acts as the main enzyme for the catabolism of dopamine in prefrontal cortex. Specifically, healthy individuals carrying both the AKT1 rs1130233 A allele (associated with decreased AKT1 expression) and the COMT rs4680 valine allele – which increases COMT activity compared to the met allele [39], possibly lowering synaptic dopamine tone – had lower prefrontal efficiency relative to all other AKT1-COMT genotypic configurations while performing a working memory task during fMRI.
Altogether, these results highlight the relevance of high-field fMRI in elucidating how functional genetic variation of relevance for schizophrenia affects brain phenotypes crucially associated with this brain disorder. Furthermore, they are consistent with the notion that the genetic architecture of schizophrenia is heterogeneous and more likely associated with interactive mechanisms among several genetic variants. Therefore, the investigation of association between single genetic variants and brain intermediate phenotypes of schizophrenia would probably have only a limited amount of information on the pathophysiological mechanisms of the disease. Accordingly, recent advances in this field are starting to address the relationship between polygenic effects of multiple genetic variants and brain intermediate phenotypes. For example, Walton et al. [142] derived a polygenic risk score, based on more than 600 genetic variants associated with schizophrenia in a separate discovery sample, and demonstrated that increased polygenic risk for schizophrenia predicted DLPFC inefficiency during a working memory task, coherently with other reports [143, 151]. These results strengthen the notion that a polygenic approach is able to detect complex patterns of schizophrenia-related neural dysfunctions, which is in line with the complex genetic architecture underlying the disease. Consistent with this notion, novel and intriguing methodological concepts are arising, which are pushing this field of research toward the investigation of the relationship between genetic networks and brain functional activity. This approach integrates to the analysis of a large amount of genetic and molecular information in order to identify biological ensembles implicated in brain disorders [57]. Using this perspective, Richiardi et al. [111] report findings suggesting that physiological resting state brain functional connectivity is linked with the coordinated activity of several genes possibly modulating ion channels and synaptic function. A logical following step of this investigation will be the study of the relationship between gene networks and intermediate phenotypes of relevance to schizophrenia.
22.4 fMRI as a Prevention Tool: The At-Risk Mental State for Psychosis and Brain Functional Imaging-Associated Features
High-field fMRI allows the detection of intermediate phenotypes for schizophrenia as well as the investigation of their association with individual genetic variability of relevance for this brain disorder. However, if risk for schizophrenia is genetically mediated and affects neurodevelopmental mechanisms [147], it is likely that schizophrenia-specific brain functional features are present before the clinical onset of the disorder. Extending knowledge on brain functional anomalies during the period of life preceding full-blown schizophrenia would be useful for clinical research and practice in order to early identify people at risk for the illness and might allow the implementation of prevention and/or intervention strategies targeting these individuals [159, 160].
In this regard, schizophrenia is often preceded at the clinical level by a “prodromal phase,” defined as a critical period which could last from few days to around 5 years, characterized by a marked behavioral alteration and by a significant personal and social decline. Moreover, during the prodromal period, some psychotic-like symptoms may appear, which are usually different from those characterizing the acute phase of schizophrenia in terms of attenuated frequency, duration, and/or intensity. Because of this attenuated symptomatology, none of these symptoms could fall into any diagnostic category [159–161]. Current evidence has highlighted that some clinical variables could be predictive of the transition to psychosis. For example, high levels of unusual content of thoughts, high level of paranoid thoughts, low personal, occupational and social functioning, history of drug addiction, and exposure to stressful life events seem to increase risk for conversion to acute psychosis [74]. Previous studies have used specific criteria in order to identify the subclinical population of “at-risk mental state” (ARMS), i.e., individuals between 15 and 30 years [40] who suffer from attenuated psychotic symptoms but cannot be diagnosed with psychosis yet [161]. Meta-analytic [52, 53] and longitudinal studies [31, 83, 96, 160] on ARMS subjects suggest that the risk of transition to full-blown psychosis progressively increases during the subsequent 3 years after their first evaluation.
A wide body of literature demonstrated that cognitive deficits in the domains of working memory, attention, executive functioning, verbal fluency, and social cognition are present in at-risk subjects [52], in whom they could be considered as primary symptoms [23] and independent from drug treatment and course of the disease [48]. Previous literature suggests that they could be also considered as an index of functional outcome and response to treatment [79]. Specifically, results in literature demonstrated that people at risk who subsequently convert to psychosis, compared with those who do not convert, have more severe cognitive impairment in several cognitive domains [158], especially in verbal fluency and memory [60, 106]. However, the neural substrates of the cognitive deficits exhibited by ARMS subjects have not been fully investigated. Furthermore, results published in this context are often weak, also due to the small sample sizes used.
fMRI studies performed to date have indicated that the brain functional abnormalities found in ARMS subjects are qualitatively similar, but less severe, than those exhibited by subjects at their first episode of psychosis (FEP, [56]) and that ARMS have patterns of brain activation which are intermediate between those displayed by full-blown psychotic patients and normal controls [27, 95]. For example, Morey et al. [95] found that the behavioral performances of ARMS subjects during a cognitive control task were lower than those of normal controls, but greater than those of FEP and chronic schizophrenia patients. Furthermore, fMRI activity during the task revealed that ARMS subjects, when compared to normal controls, had lower BOLD response in the middle and the inferior frontal gyrus, while they had greater activation of these loci when compared to FEP and schizophrenia patients. Overall, these results suggest that both behavioral and imaging abnormalities are associated with the progression of the disease. Furthermore, they also suggest that brain functional anomalies predate the onset of the illness and are associated with vulnerability to psychosis, rather than with the diagnosis itself. On the other hand, other studies have indicated that ARMS subjects exhibit greater activation than normal controls in medial and inferior frontal regions [28, 54, 100]. Moreover, further work found patterns of both increased and decreased activation in ARMS subjects during cognitive processing in basal ganglia, precuneus, occipital, parietal, postcentral, and supramarginal gyrus compared to controls [1, 54, 55, 114, 157]. Also, other findings indicate that prefrontal, cingulate, and parietal cortex hypoactivation is associated with transition from ARM state to full psychosis [125, 153]. All together, these results suggest that ARMS are characterized by different brain functional anomalies compared to healthy controls and FEP on one hand. On the other hand, the lack of consistency of the results makes it difficult to clearly identify an unambiguous pathophysiological pattern characterizing ARMS.
An emerging approach for the characterization of potential markers of risk for psychosis is the analysis of brain functional networks. In this regard, Allen et al. [1] used dynamic causal modeling (DCM) techniques in order to investigate effective frontotemporal connectivity during a sentence completion task. Results of this study indicate an increase in activation of the cingulate cortex in ARMS subjects when compared to healthy controls. Furthermore, there was a relationship between cingulate activity and frontotemporal connectivity, such that the more the activity of cingulate cortex in ARMS, the more the frontotemporal connectivity was similar between these subjects and controls. These results could suggest that ARMS subjects need compensatory activation of the cingulate cortex in order to maintain optimal levels of frontotemporal connectivity, thus revealing connectivity anomalies associated with the prodromal phase of psychosis. Similarly, Lord et al. [89] used a graph theory approach to investigate patterns of abnormal connectivity in ARMS during a verbal fluency task. The anterior cingulate cortex (ACC) was the network hub of this analysis. Results revealed that ARMS and normal controls did not differ in terms of global connectivity and efficiency of networks. However, ACC was less involved in contributing to task relevant functional connections in high symptomatic compared to low symptomatic ARMS subjects and to normal controls, as suggested by the analysis of topological centrality. These results suggest that connectivity abnormalities predate the onset of full-blown psychosis and could be associated with psychosis risk. Furthermore, they also suggest that advanced neuroimaging techniques could have a predictive value in order to detect anomalies associated with the risk for psychosis. In this regard, the strong and groundbreaking clinical potential of high-field fMRI for the early identification of at risk individuals should be well explored in the next future.
Conclusions
All studies here reported support the notion that high-field fMRI is capable to detect neurobiological anomalies that are associated with schizophrenia, which has heterogeneous clinical correlates. Indeed, fMRI, more than behavioral and clinical tools, is providing brain phenotypes closer to the effect of the complex genetic architecture of this disease and, as such, is likely to characterize markers of its risk. These markers might in future provide clinical practice with useful information about potential predictors of psychosis, such that they might be used as targets for strategies of prevention in order to mitigate trajectories of development of schizophrenia. However, the heterogeneity of findings and the lack of robust longitudinal studies comparing individuals who subsequently develop psychosis with subjects who do not call for stronger efforts in order to shed more light on the brain correlates of schizophrenia and of risk for this brain disorder. Such efforts might also help in softening the social burden associated with this devastating brain illness, which is paradigmatic of the relevant impact of psychiatric diseases for the entire community.
References
1.
Allen P, Stephan KE, Mechelli A, Day F, Ward N, Dalton J, Williams SC, McGuire P (2010) Cingulate activity and fronto-temporal connectivity in people with prodromal signs of psychosis. NeuroImage 49:947–955PubMedPubMedCentral
2.
Amar S, Shaltiel G, Mann L, Shamir A, Dean B, Scarr E, Bersudsky Y, Belmaker RH, Agam G (2008) Possible involvement of post-dopamine D2 receptor signalling components in the pathophysiology of schizophrenia. Int J Neuropsychopharmacol 11:197–205PubMed
3.
American Psychiatric Association (2013) Diagnostic and statistical manual of mental disorders, 5th edn. American Psychiatric Publishing, Arlington
4.
Anticevic A, Cole MW, Repovs G, Murray JD, Brumbaugh MS, Winkler AM, Savic A, Krystal JH, Pearlson GD, Glahn DC (2014) Characterizing thalamo-cortical disturbances in schizophrenia and bipolar illness. Cereb Cortex 24:3116–3130PubMed
5.
Antonucci LA, Taurisano P, Fazio L, Gelao B, Romano R, Quarto T, Porcelli A, Mancini M, Di Giorgio A, Caforio G, Pergola G, Popolizio T, Bertolino A, Blasi G (2016) Association of familial risk for schizophrenia with thalamic and medial prefrontal functional connectivity during attentional control. Schizophr Res 173:23–29PubMed
6.
Barch DM, Carter CS, Braver TS, Sabb FW, MacDonald A 3rd, Noll DC, Cohen JD (2001) Selective deficits in prefrontal cortex function in medication-naive patients with schizophrenia. Arch Gen Psychiatry 58:280–288PubMed
7.
Bearden CE, Freimer NB (2006) Endophenotypes for psychiatric disorders: ready for primetime? Trends Genet 22:306–313PubMed
8.
Beaulieu JM, Sotnikova TD, Marion S, Lefkowitz RJ, Gainetdinov RR, Caron MG (2005) An Akt/beta-arrestin 2/PP2A signaling complex mediates dopaminergic neurotransmission and behavior. Cell 122:261–273PubMed
9.
Beaulieu JM, Tirotta E, Sotnikova TD, Masri B, Salahpour A, Gainetdinov RR, Borrelli E, Caron MG (2007) Regulation of Akt signaling by D2 and D3 dopamine receptors in vivo. J Neurosci 27:881–885PubMed
10.
Benedetti F, Poletti S, Radaelli D, Bernasconi A, Cavallaro R, Falini A, Lorenzi C, Pirovano A, Dallaspezia S, Locatelli C, Scotti G, Smeraldi E (2010) Temporal lobe grey matter volume in schizophrenia is associated with a genetic polymorphism influencing glycogen synthase kinase 3-beta activity. Genes Brain Behav 9:365–371PubMed
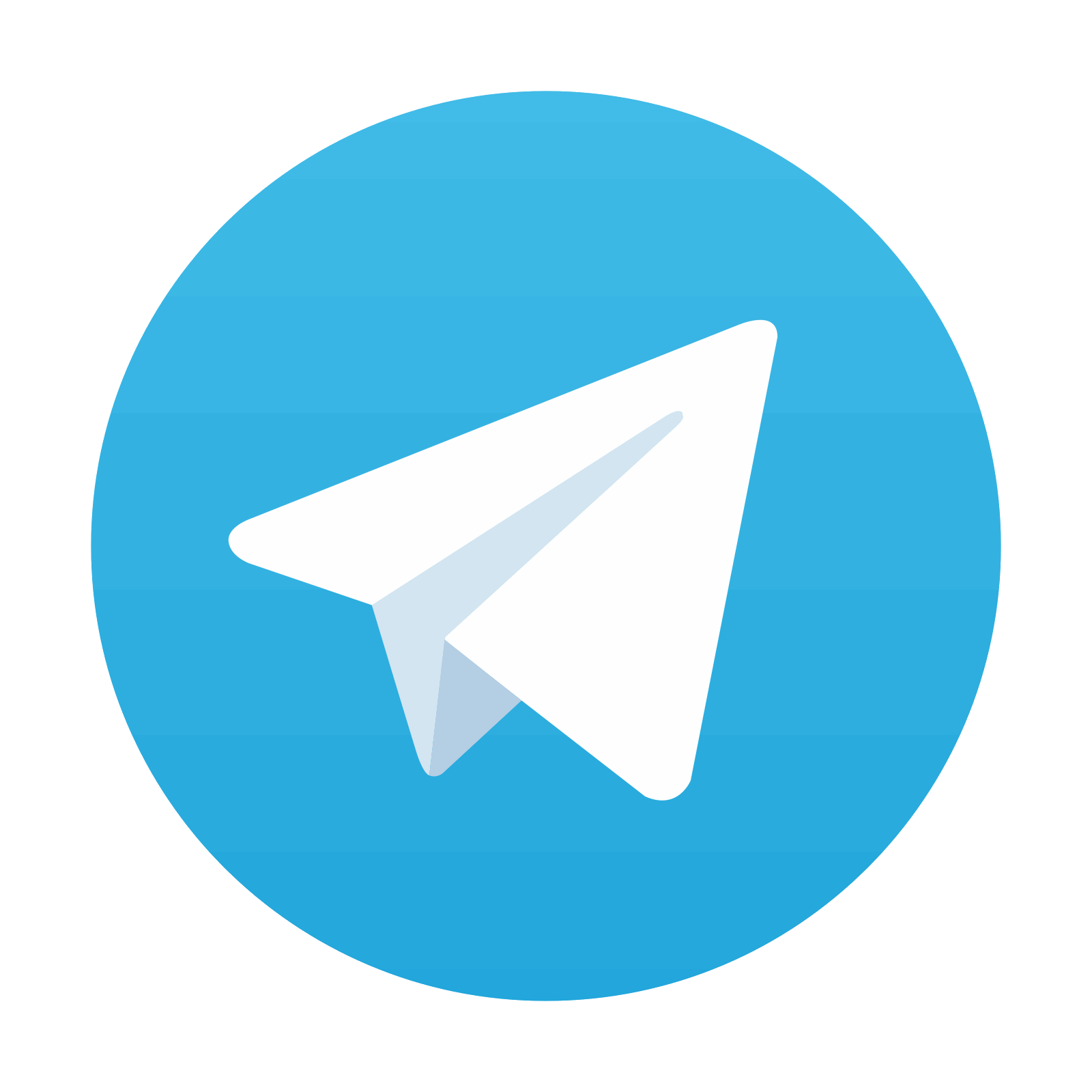
Stay updated, free articles. Join our Telegram channel

Full access? Get Clinical Tree
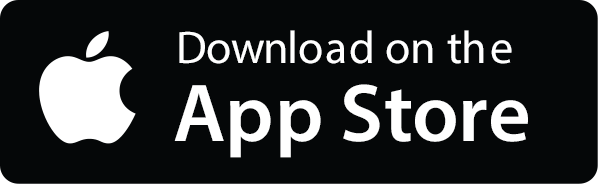
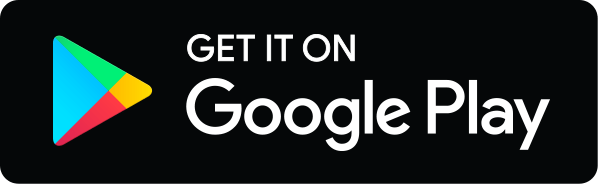