Fig. 5.1
Normal arterial circulation: high-definition 3D TOF image at 3.0 T (matrix 1024×512, FOV 240 mm, slice thickness 1 mm) (a); detail of the circle of Willis, especially of the posterior circle (b); also note detail of the right middle cerebral artery branches (c)
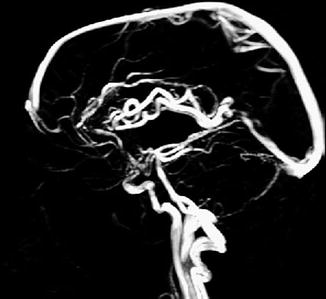
Fig. 5.2
Normal venous cerebral circulation: 3D PC study at 3.0 T
Drawbacks with respect to conventional angiography are lower spatial and, above all, temporal resolution. The quality of MR angiographic images has recently been improved even in lower field systems by the advent of surface or phased array coils with a higher signal/noise ratio (SNR) and shorter echo times (TE) made possible by stronger gradients, new pulse sequences with optimized SNR and longer acquisition times (given that a fourfold increase in examination time doubles the SNR).
5.1 MRA Techniques
Although there are several approaches to visualizing the intracranial vessels with MR, the two most widely used techniques are time-of-flight (TOF) and phase-contrast (PC) MRA.
In TOF MRA, which is usually performed using a flow-compensated gradient-refocused sequence, stationary tissues are saturated and thus have low signal intensity. However, blood upstream of the imaging volume is unsaturated. When this blood flows into the imaging volume, it is bright compared with the stationary background tissue [1].
For vessels coursing within the acquired section, the inflow effect becomes less effective, reducing intravascular signal to the level of the surrounding stationary spins. Potential difficulties in TOF MRA may therefore arise in situations where larger sections of vessels lie within a section and also in situations where turbulent flow is present, as this also suppresses bright intravascular signal.
In TOF MRA, vessels appear bright independent of the flow direction. Hence, arteries and veins cannot be differentiated. Flow in a particular direction can, however, be saturated using spatial flow presaturation bands. Spins being washed into the slice from the presaturation area will not carry any magnetization, and no inflow enhancement occurs [2]. These saturation bands can thus be used to obtain selective TOF angiograms or venograms.
Unlike TOF MRA, PC MRA utilizes phase shifts as blood flows in the presence of flow-encoding bipolar gradients. On phase-difference images, the signal phase intensity is proportional to velocity, resulting in suppression of the stationary background tissue. The flow-encoding gradients can be applied in any one or multiple directions depending on the desired flow sensitivity [1]. This technique is thus a direct velocity map, where the voxel intensity values are proportional to the actual flow velocity in a particular flow direction.
Because the typical imaging time of these conventional MRA techniques is between 2 and 8 min, neither technique is able to demonstrate the dynamics of cerebral blood flow. Moreover, both techniques are prone to artefacts resulting from slow, turbulent or complex blood flow.
Most of the problems associated with TOF MRA and PC MRA can be overcome with contrast-enhanced MRA (CE-MRA) [1, 3]. Three-dimensional CE-MRA fundamentally differs from other vascular MR imaging strategies in that it is not flow dependent. Blood signal is derived from the T1-shortening effect of the dynamically infused paramagnetic contrast. Hence, arterial contrast is based on the difference in T1 relaxation between blood and surrounding tissue [4]. As a result, problems associated with slow flow and turbulence-induced signal voids are overcome. The technique allows a small number of slices oriented in the plane of the vessels of interest to image an extensive region of vascular anatomy in a short period of time [5]. With this technique, a temporal resolution of about one image in 20 s has become possible, enabling selective demonstration of the early arterial and late venous phase [5–7].
MRA images are best interpreted on an independent workstation with 3D reconstruction capabilities. In addition to perusal of the original sections, diagnoses should be based on a combination of maximum intensity projection (MIP) images and interactive 3D multiplanar reformations (MPR). The MPR technique permits cross-sectional visualization of the vessels in any plane. Venous overlap can effectively be compensated, and the course of tortuous vessels can easily be reconstructed. This represents an advantage even over conventional catheter angiography. Surface-rendering algorithms as well as virtual angioscopic reconstructions are useful mainly for demonstration purpose.
5.2 3.0 T MRA
3.0 T MRA offers significant advantages (Figs. 5.3, 5.4, 5.5 and 5.6) [8–11].
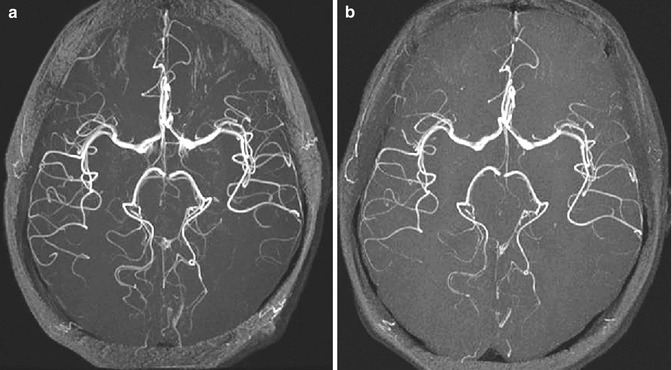
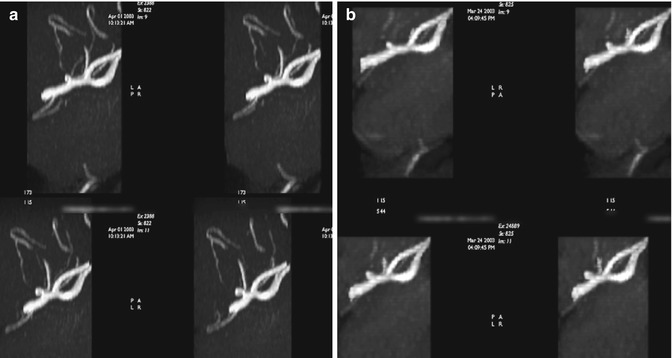
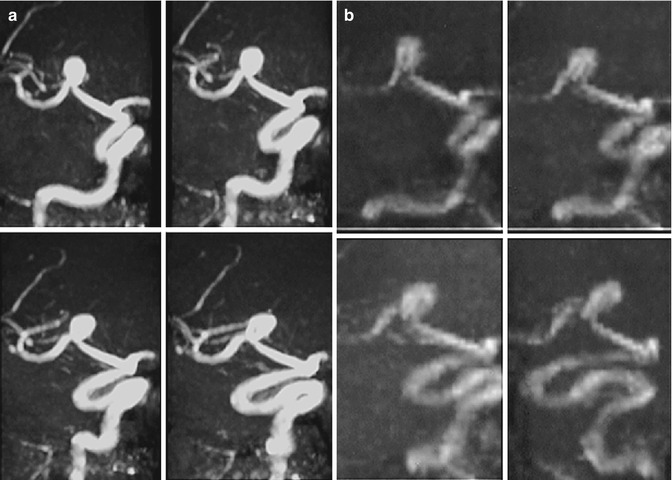
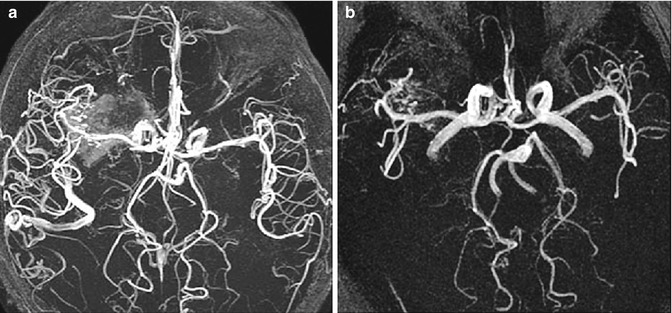
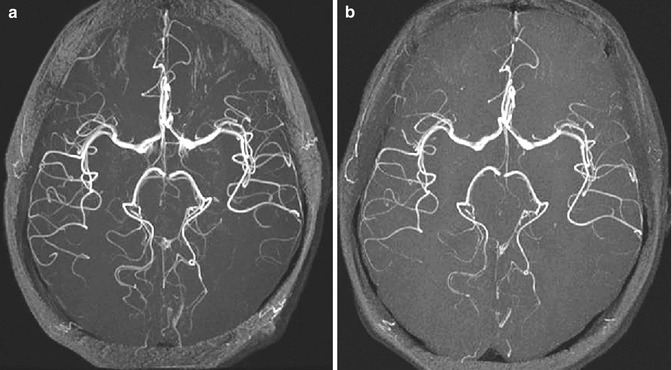
Fig. 5.3
Normal arterial cerebral circulation imaged using the same 3D TOF sequence at 3.0 T (a) and1.5 T (b). In (a), vessel conspicuity is greater than in (b), yielding better and more detailed depiction of superficial, smaller calibre vessels
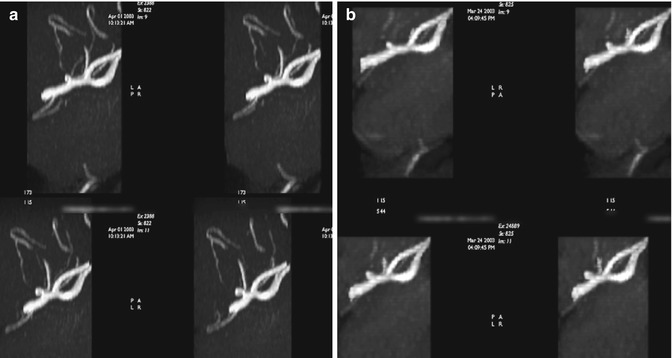
Fig. 5.4
Coiling of a branch of the right middle cerebral artery studied with a 3D TOF sequence at 3.0 T (a) and 1.5 T(b). The coil is better depicted in (a) than in (b), where the image mimics a small aneurysm
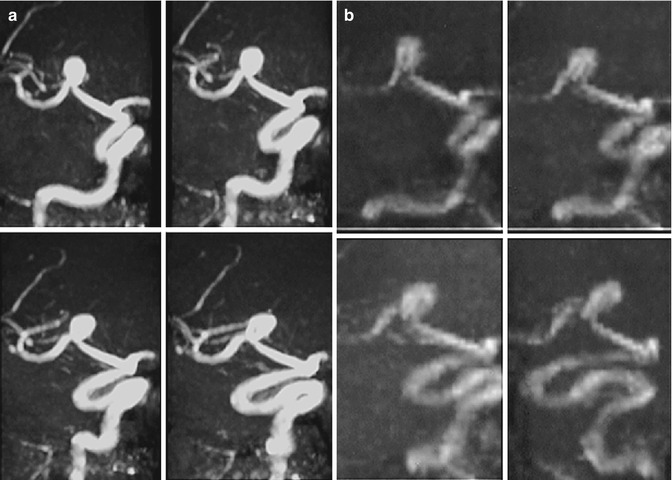
Fig. 5.5
Sacciform aneurysm of middle cerebral artery imaged using the same 3D TOF sequence performed at 3.0 T (a) and 1.5 T (b). The sacciform vascular dilatation is depicted in greater detail and exhibits a more intense signal in (a) than in (b)
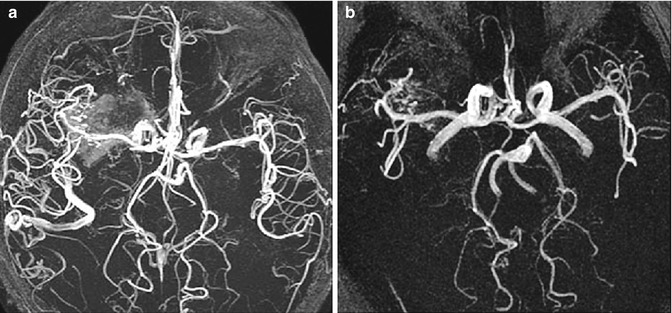
Fig. 5.6
Large right arteriovenous malformation: unenhanced 3D TOF images acquired at 3.0 T (a) and 1.5 T (b). Greater background suppression and flow enhancement afford better spatial depiction of the malformation at 3.0 T
First of all, it allows to perform all the angiographic sequences applied routinely in clinical practice with lower field systems, such as 2D and 3D TOF and PC, as well as ultrafast dynamic sequences after administration of a bolus of contrast agent (CE-MRA).
Similarly to standard brain MRI, the technical parameters of MRA sequences also need to be optimized at higher magnetic fields (Table 5.1).
Table 5.1
Optimization of technical parameters of MRA sequences at higher magnetic fields
Sequence | TR (ms) | TE (ms) | Other parameters (TI, FA, ZIP) | Slice thickness (mm) | No. of slices | FOV | Matrix | NEX | Examination time (min:s) |
---|---|---|---|---|---|---|---|---|---|
![]() | 30 | 6.9 | FA 30 | 1.2 | 32 | 24 | 352×224 | 1 | 3:08 |
![]() | 26 | Min | FA 20 ZIP 512 ZIP 2 | 1.4 | 60 | 16 | 288×224 | 1 | 5:53 |
![]() | 30 | Min | FA 20 ZIP 1024 ZIP 2 | 1.4 | 48 | 19 | 384×320 | 1 | 6:18 |
![]() | Min | Min | 70 | 1.5 | 23 | 256×224 | 1 | Variable in relation to number of slices | |
![]() | Min | Min | 50 | 1.4 | 22 | 256×192 | 1 | Variable in relation to number of slices | |
![]() ![]() Stay updated, free articles. Join our Telegram channel![]() Full access? Get Clinical Tree![]() ![]() ![]() |