Fig. 6.1
Schematic representation of the different strategies developed to enhance SC migratory potential in the CNS. Different approaches improved SC remyelination capacity whether directly by modifying SC properties, decreasing inhibitory molecules present in the environment, or by adjusting axonal signals
6.2.2.1 Modifying SC Intrinsic Properties
Transduction of SC has been used as a mean to act as gene delivery vehicles. Boosting SC expression of neurotrophins such as BDNF and NT3 has profound effects on neural protection, plasticity, and regeneration after spinal cord injury in the developing and adult CNS (Kromer and Cornbrooks 1985; Martin et al. 1991; Montero-Menei et al. 1992; Levi and Bunge 1994). Since NT3 also promotes oligodendrocyte proliferation, survival and differentiation (McTigue et al. 1998), and SC migration (Yamauchi et al. 2005) in vitro, we explored the benefit of this strategy on remyelination after transplantation into the demyelinated spinal cord. In doing so, we demonstrated that forced expression of BDNF and NT3 in macaque SC promoted functional motor recovery of the demyelinated mouse spinal cord (Girard et al. 2005). This beneficial effect resulted from multiple events including enhanced remyelination by endogenous oligodendrocytes and SC, enhanced neuroprotection, and reduced scar formation, thus highlighting the issue of ex vivo neurotrophin delivery based on SC therapy to enhance global repair of demyelinated lesions. A similar strategy combining overexpression of NT3 and BDNF was also used in spinal cord trauma and found to improve efficiently axonal length and the number of myelinated axons in the lesion (Golden et al. 2007). However this strategy did not distinguish a direct or indirect effect of neurotrophins on myelination, through either creating an increase in axon number and/or acceleration of SC differentiation.
An alternative strategy to enhance their intra-CNS integration/migration has been to modify SC membrane properties. As mentioned above, one major limitation of SC-based therapy is the poor potential of their integration/migration within white and gray matter of the CNS. By contrast, neural precursors and OEC interact more efficiently with astrocytes. Interestingly, both of these cells express the polysialylated form of NCAM (PSA-NCAM), a cell adhesion molecule highly expressed during development and involved in CNS plasticity, remodeling, and repair (reviewed in Rutishauser 2008). Since SC express NCAM but not PSA, it was speculated that forcing NCAM polysialylation in SC would improve their integration/migration in the CNS. Indeed, ectopic expression of PSA by rodent SC enhanced their migratory properties in vitro and functional repair in a trauma model in vivo without altering their myelination potential (Lavdas et al. 2006; Papastefanaki et al. 2007). This strategy was further explored with adult macaque SC grafted in the demyelinated rodent spinal cord. Forced expression of PSA-NCAM by the grafted SC enhanced their recruitment by distant lesions and promoted their ability to remyelinate the demyelinated axons while wild-type SC were unable to do so (Bachelin et al. 2010). This pro-migratory effect resulted in increased SC fluidity and exit from the graft most likely by reduced adhesion among sister SC and SC–astrocytes. Alternatively, it may also have decreased SC sensitivity to inhibitory molecules and/or increased their response to chemotactic cues as demonstrated for PSA-NCAM+ embryonic-stem-cell-derived neural precursors (Glaser et al. 2007).
6.2.2.2 Modifying the Environment
Other strategies have targeted specific molecular cues of the glial scar. Indeed, reducing levels of chondroitin sulfate proteoglycans (CSPG) (Grimpe and Silver 2004; Santos-Silva et al. 2007) and N-cadherin–N-cadherin (Fairless et al. 2005) interaction promoted SC–astrocyte mixing in vitro and in vivo. Interestingly, another inhibitor of axonal regeneration, semaphorin 3A (Sema 3A), was found to be repulsive for SC in vitro and in vivo in a model of spinal trauma. Treatment with a Sema 3A inhibitor was able to reverse this effect and increase SC-mediated myelination and axonal regeneration (Kaneko et al. 2006). Data also indicate that ephrins may play a role in SC–astrocyte non-mixing (Afshari et al. 2010). However, whether neutralizing these axonal growth inhibitors would also improve exogenous SC migration through CNS parenchyma has not been reported so far. Several inhibitors of axonal regeneration are also contained in myelin. However, their role in Schwann cell migration inhibition remains to be elucidated. Interestingly, SC, unlike OEC and OPC, do not secrete metalloendoproteases, enzymes known to degrade inhibitors as extracellular matrix proteoglycans and myelin axonal inhibitors Nogo (Amberger et al. 1997; Gueye et al. 2011).
Another issue with SC-based therapy concerns the control of SC myelination by axon diameter. Injections of SC into areas of small-diameter axons do not result in remyelination (Brook et al. 1993). However, using lentiviral-driven overexpression of NRG 1 type III, Taveggia et al. (2005) induced SC to myelinate small-diameter axons. Furthermore, overexpression of NRG1 type III in transgenic mice increased the thickness of endogenous newly formed PNS but not CNS myelin in response to demyelination (Brinkmann et al. 2008). Interestingly, several members of the ADAM (a disintegrin and metalloprotease) secretase have been implicated in the regulation of NRG 1 type III activity notably by regulating its processing. While some secretases such as BACE (beta secretase) positively regulate the myelination process (Shirakabe et al. 2001; Hu et al. 2006), recent data showed that another secretase TACE (tumor necrosis factor alpha-converting enzyme) is a negative regulator of PNS myelination (La Marca et al. 2011). These last observations underline the therapeutic potential of TACE modulation since several inhibitors of TACE are already under investigation for other disease treatment. The possibility to direct selectively exogenous SC remyelination towards CNS axons irrespectively of their size may thus be useful in enhancing their contribution to CNS remyelination.
6.2.3 OEC
OEC (initially named olfactory SC) are differentiated glial cells very similar to SC that belong to the peripheral olfactory system where they ensheath but do not myelinate the axons belonging to the first cranial nerve (Franklin and Barnett 2000; Vincent et al. 2005).
They share also features with astrocytes expressing “fibrillar” GFAP and forming part of the glial limitans of the olfactory bulb (Doucette 1991). The origin of OEC has long been controversial. During early development, they originate from the nasal placodes and provide essential growth and guidance to sensory axons which they do not myelinate. Although they were believed to be ectodermal derivatives, recent evidences based on the Wnt1Cre fate mapping technology show that at early embryonic stages OEC within the placode derive from neural crest cells (NCC) (Barraud et al. 2010; Forni et al. 2011). These findings could explain the high level of similarities existing between OEC and SC. In view of these recent data, we will consider OEC as an alternative source of neural crest-derived cells rather than cells deriving from another lineage.
In vitro, OEC and SC have remarkable similarities. Both cell types express the low-affinity NGF receptor (p75ntr), S100, GFAP, and cell adhesion molecules such as L1 and NCAM. They also express extracellular matrix proteins such as laminin and fibronectin (Wewetzer et al. 2002) and secrete neurotrophins including NGF, BDNF, FGF, and vascular endothelial growth factor (VEGF) (reviewed in Sasaki et al. 2011). Although OEC do not normally myelinate the very small axons of the first cranial nerve, studies have shown that OEC can (re)myelinate larger axons with myelin that is indistinguishable morphologically and biochemically from that made by SC when transplanted in the CNS. This was first observed in cocultures of OEC with DRG neurons (Devon and Doucette 1992) and further confirmed in vivo with an OEC cell line (Franceschini and Barnett 1996) and primary OEC (Franklin et al. 1996; Smith et al. 2001; Lakatos et al. 2003b). Cell suspensions of acutely dissociated OEC from neonatal rats remyelinated and enhanced axonal conduction, when focally injected into the ethidium-bromide-demyelinated areas of the dorsal columns of the spinal cord (Imaizumi et al. 1998). Moreover, xenotransplanted canine, human, or porcine OEC isolated from the adult olfactory bulb are capable of extensive functional remyelination following transplantation into demyelinated rat CNS (Kato et al. 2000; Smith et al. 2001; Deng et al. 2006). The myelin formed contains the peripheral myelin protein P0, and the cell’s association with axons resembles that of SC. Furthermore, when faced with demyelinated axons, transplanted OEC express SC transcription factors Krox-20 and SCIP mRNA, suggesting that similar mechanisms are involved in the manner OEC and SC form myelin (Smith et al. 2001). In addition to these observations, OEC transplantation in trauma models have suggested that OEC have multiple beneficial effects, reducing glial scarring and cavitation, increasing angiogenesis, and providing a cellular scaffold for regenerating axons (reviewed in Toft et al. 2011). Improved regeneration and reduced scarring was also observed after autologous transplantation in a canine model of spinal cord injury (Jeffery et al. 2005). However, the repair capacities of OEC have been challenged given that their ability to support axonal growth was not fully confirmed (Tetzlaff et al. 2011). It was also reported that OEC are less efficient than SC at forming myelin in vitro and in vivo (Plant et al. 2003; Boyd et al. 2004). In addition, it was proposed that a SC contamination could be responsible for all the observed effects (Boyd et al. 2004). However, transplantation of eGFP-OEC in a contusive SCI lesion (Sasaki et al. 2004), in the injured nerve (Dombrowski et al. 2006; Radtke et al. 2009), or in the X-EB lesions (Sasaki et al. 2011) provided proof that OEC have the ability to remyelinate axons with, in some circumstances, recovery of locomotor functions. Moreover, co-transplantation of purified OEC and meningeal cells indicated that this potential can be enhanced by meningeal cells which contaminate OEC preparations (Lakatos et al. 2003a). So far, the behavior of OEC in a context of immune inflammation comparable to MS has not been addressed.
Several studies suggested that OEC migrate better than SC when confronted with CNS components. Indeed, OEC mix better when cocultured with astrocytes (Lakatos et al. 2000), and transplanted OEC elicit a diminished expression of GFAP and CSPG expression in astrocytes compared to SC (Lakatos et al. 2003a; Garcia-Alias et al. 2004; Andrews and Stelzner 2007). This is explained via decreased N-cadherin-mediated adhesion with astrocytes (Fairless et al. 2005) and induction of a weaker astrocyte hypertrophy (Lakatos et al. 2000). Moreover, as mentioned before, OEC secrete matrix metalloproteinases (MMPs), which modulate their migration in vitro (Gueye et al. 2011). Since MMPs also induce changes in astrocyte morphology (Ogier et al. 2006), these enzymes could also play an important role in OEC mixing with astrocytes.
However, the greater migration potential of OEC in the injured spinal cord remains a subject of debate (Deng et al. 2006; Lu et al. 2006; Pearse et al. 2007). While several studies show that OEC, SC, and OPC survive and migrate poorly after transplantation in normal white matter (Iwashita et al. 2000; Hinks et al. 2001; Lankford et al. 2008), only one report highlights that transplanted OEC (and OPC), but not SC, migrate extensively in gray and white matter of the X-irradiated spinal cord (Lankford et al. 2008). Although these data stress potential differences between transplanted SC and OEC with respect to their sensitivity to CNS inhibitory cues. However the specific environment of the X-irradiated spinal cord besides NG2+OPC a depletion in NG2+ OPC remains to be identified.
6.2.4 Advantages of OEC over SC
Since OEC myelinate in a manner very similar to SC but mix better with astrocytes in vitro and in vivo, it has been proposed that from a therapeutic point of view, OEC transplantation would have advantages over SC. However, immature stages of the SC lineage migrated profusely on transplantation into the CNS (Fig. 6.2 and 6.3) and elicited similar reduced changes in astrocyte reactivity including CSPG production (Fig. 6.4) (Woodhoo and Sommer 2008; Zujovic et al. 2010). This suggests that OEC represent a stage of the neural crest lineage with plasticity more similar to SC precursors (SCp) and boundary cap cells (BC, see further) than mature SC.
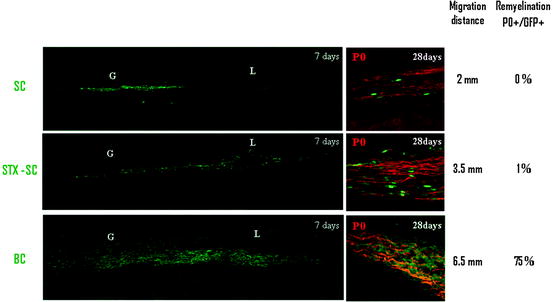
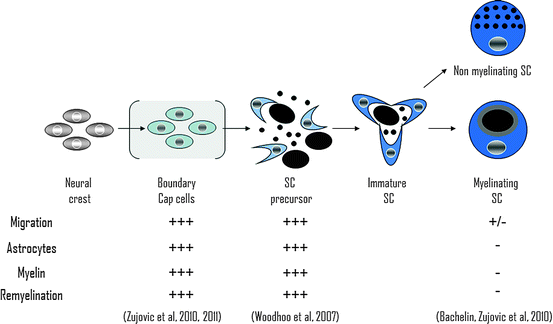
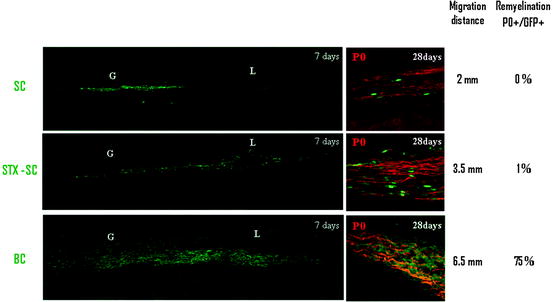
Fig. 6.2
Repair potential of different stages of the SC lineage of mature myelinating SC. The migratory and remyelination properties of mature myelinating SC in the CNS are challenged by their poor interaction with astrocytes and myelin inhibition. In contrast, earlier stages of SC lineages such as BC and SCp present higher repair potential due to their smoother interaction with CNS environment
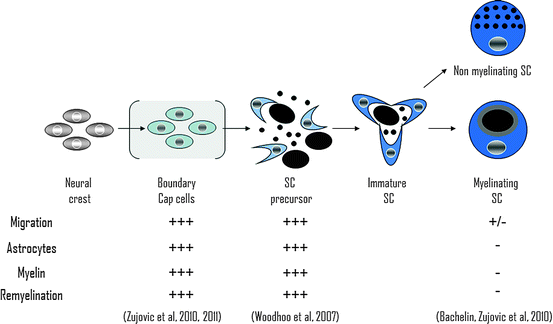
Fig. 6.3
Comparison of BC, STX-SC, and SC interaction with CNS environment. One week post grafting, BC and STX-SC progeny intermingle with astrocyte (blue, left panel) while adult SC graft induces the formation of an astrocytic barrier (blue, left panel). CSPG (red, middle panel) production was lower in BC and STX-SC graft than in SC graft. Only BC were able to migrate in MOG+ areas (red, right panel). The table summarizes the behavior of SC, STX-SC, and BC in CNS environment
Whether OEC and SC display species differences in terms of growth factor requirements for expansion has also been investigated. Similar to rodent OEC, OEC from large animal species share many shared features with SC. In fact a recent review by Wewetzer et al. (2011) concluded that rodent, but not canine, human, and monkey, OEC and SC require mitogens for expansion and undergo spontaneous immortalization after prolonged growth factor treatment. These observations highlight that both canine and monkey OEC and SC may serve as valuable translational models for addressing their regenerative capacities at a preclinical stage.
Autologous SC grafts in monkeys and autologous OEC grafts in dogs have been proven feasible and successful in terms of repair. Whether human OEC and SC have equivalent regenerative/remyelinating potentials remain to be defined. Considering autologous grafts studies in humans, lack of adverse effects but no proof of benefit were reported for autologous SC grafts in the injured spinal cord injury 1 year after injury (Saberi et al. 2008). Likewise no conclusions except for safety could be drawn from the suspended MS trial. Autologous olfactory mucosa engraftment (Lima et al. 2006) and autologous olfactory ensheathing cell transplantation (Feron et al. 2005) appeared to be safe and/or provide potential benefit. However, their application to promote remyelination/regeneration in MS patients has not been reported. Clearly more studies on MS-like preclinical models are necessary to evaluate the outcome of grafted cells in an inflammatory demyelinating milieu.
6.3 PNS Progenitors
An alternative to the use of committed SC or OEC for cell therapy would be the use of more immature stages of the neural crest (NC) lineage. While embryonic and adult precursors have been identified, few reports have addressed the repair potential of these PNS stem/precursor cells in the CNS. These include SC precursors (SCp), boundary cap cells (BC), and olfactory epithelial progenitors (OEp).
6.3.1 SCp
Schwann cell precursors are NC derivatives, which undergo various transitional stages before becoming myelin-competent cells (reviewed in Zujovic et al. 2007). Neural crest cells (NCC) differentiate into SC precursors (SCp) before becoming immature SC and mature myelinating or nonmyelinating SC. Woodhoo et al. highlighted the greater capacity of SCp to survive, integrate, and differentiate into myelin-forming cells when transplanted in the normal or demyelinated CNS (Fig. 6.2) (Woodhoo et al. 2007). When grafted into the injured spinal cord, these cells supported axonal growth and sprouting though significant functional improvements were not observed (Agudo et al. 2008).
6.3.2 Boundary Cap Cells
BC are potential stem cells (Hjerling-Leffler et al. 2005) of the embryonic mouse spinal roots (Maro et al. 2004; Aquino et al. 2006) closely related to NCC. When grafted in the demyelinated sciatic nerve, acute BC preparations differentiated into myelin-forming SC only if in vitro priming to committed SC was performed prior engraftment (Aquino et al. 2006). Using cell fate mapping and microdissection, we provided evidence that acutely dissociated BC migrate profusely in the demyelinated CNS and compete aggressively with host myelin-forming cells to remyelinate axons of far distant lesions of the spinal cord while SC are unable to do so (Zujovic et al. 2010) (Fig. 6.4). Although it is not clear why BC and SCp migrate more efficiently through normal white matter, expression of PSA-NCAM may be involved. However, BC, but not SCp, express PSA-NCAM, suggesting that mechanisms other than PSA-NCAM might intervene in efficient intra-CNS migration/integration of the BC/SCp. Moreover while SCp give rise exclusively to peripheral myelin, BC differentiate also into central myelin-forming cells in vitro and in vivo (Zujovic et al. 2011) (Fig. 6.5). While these observations highlight the remarkable therapeutic potential of these new cellular candidates, the use of BC rather than SCp to promote central remyelination might be more promising in view of their self-renewal ability and multipotency (Fig. 6.2). However, their potential for axonal regeneration is unknown.
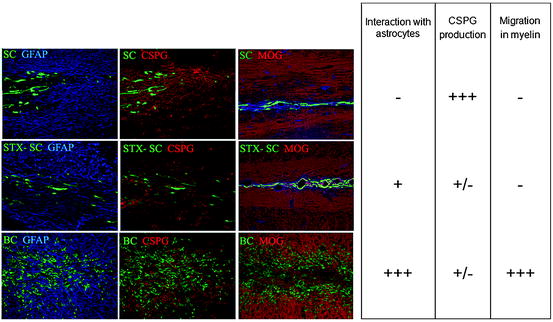
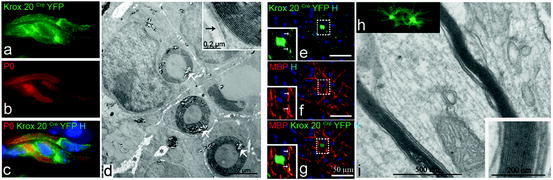
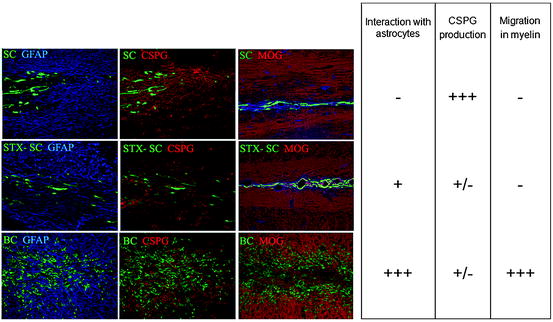
Fig. 6.4
Comparison of the migratory and remyelination potential of mature SC, STX-expressing SC, and BC. In order to increase mature SC migratory potential, two different strategies were developed: by overexpressing STX, a sialyltransferase inducing the polysialylation of NCAM, and by using more immature stages of the SC lineage such as BC. Since mature SC migrate poorly in the CNS (upper panel), they do not produce new peripheral myelin (P0, red). STX-SC migrate further than mature SC and participate more efficiently in the remyelination process. BC (lower panel) demonstrate impressive migratory capacities and participate massively in the remyelination of the lesion
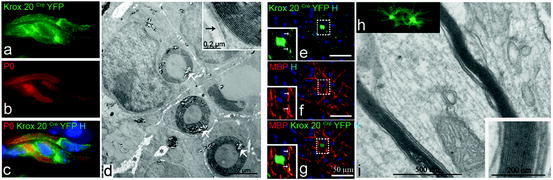
Fig. 6.5
BC directed differentiation towards a peripheral or central myelin-forming cell. When grafted in the demyelinated lesion of the spinal cord, the majority of BC give rise to SC (a–d). Four weeks post grafting, there is a clear colocalization of P0 positive myelin (red b, c) with YFP+ cells (green a, c). Bluo-gal precipitates reveal YFP+ BC (white arrows, d). BC progeny presents a classical morphology of PNS myelin with SC associated in a 1:1 relationship with the axon (d). Higher magnification illustrates the compaction of the newly formed PNS myelin and its basal membrane (black arrow, insert, d). When grafted in the newborn shiverer brain, BC adopt an oligodendroglial fate (e–i). YFP+ cells (green, e, g) expressing MBP (red, f, g) and have features of myelin-forming oligodendrocytes. Insets (e, g) are enlarged views of dotted square illustrating discrete co-expression of MBP and YFP in processes. Ultrastructural analysis confirms the presence of compact donor-derived central myelin (i, inset). Illustration of GFP+ oligodendrocytes in corresponding floating sections prior to processing for electron microscopy (h)
6.3.3 Adult PNS Stem/Precursor Cells
The persistence of NC-derived stem cells (NCSC) in the adult has been found, most notably in the adult DRG (Li et al. 2007). Li et al. suggested that the NCSC probably originate from satellite cells. Using lineage tracing with P0 and Wnt1-Cre/Floxed EGFP mice, Nagoshi et al. (2008) targeted the NCSC niches in the whisker pad, bone marrow, and the DRG and compared their multipotency and self-renewal ability. Although these various stem cells share the same NC origin and all of them give rise to neurons, glia, and myofibroblasts, the DRG-derived NCSC showed greater multipotency and an enhanced ability to form secondary spheres. Despite the observation that several tissues give rise to stem cells sharing the same origin and similar self-renewing and multipotential capacities, these cells seem to retain tissue-dependent characteristics. Whether they will have similar repair and remyelinating potential has to be addressed.
Recently, Takagi et al. (2011) were able to isolate SCp/immature SC from adult injured peripheral nerve. Based on the capacity of adult SC to differentiate after nerve injury, they collected cell suspensions from injured peripheral nerve at different time point after injury. They noted that while no sphere formation was observed in the intact sciatic nerve, Schwann spheres were obtained 3–10 days after injury. Although spheres differentiated into the SC lineage only, they contained SCp/immature SC with self-renewal capacity and greater neurite outgrowth and myelination potentials when cocultured with neurons, than mature SC.
6.3.4 Olfactory Epithelium Progenitors
Obtaining sufficient quantities of OEC from olfactory bulbs is difficult to achieve and is highly invasive, leading to total loss of olfaction. Another approach consisting in endoscopic biopsy of the olfactory epithelium was found to be less invasive and without adverse effect on olfactory function (Winstead et al. 2005). Interestingly, it is in this structure that OEp were first identified both in rodents and humans. When pieces of olfactory lamina propria containing OEp were placed into injured rat spinal cord, they promoted partial functional recovery in paraplegic rats (Lu et al. 2002). Later OEp were generated from olfactory epithelium from rodent (Tomé et al. 2009), from fresh necropsied tissue, or endoscopic biopsy from patients undergoing sinus surgery. The latter were characterized in vitro and shown to remain equivalent despite the age and sex of donor (Marshall et al. 2005, 2006). Upon transplantation into demyelinated spinal cord lesion, grafted rat OEp provided extensive peripheral remyelination (Markakis et al. 2009). Moreover, human OEp, transplanted in the rodent traumatized spinal cord, rescue axotomized rubrospinal neurons and promote functional recovery (Xiao et al. 2005).Yet, their use in disease models of trauma or demyelination deserves further attention.
6.4 Ectopic Sources
6.4.1 Mesenchymal Stem Cells
Mesenchymal stem cells (MSC) are stromal stem cells that can be isolated from various tissues and differentiate into cells of mesodermal (bone, fat, cartilage) and neuro-ectodermic lineages (neurons, glia). The most commonly studied MSC were isolated from bone marrow (Dezawa et al. 2001; Keilhoff et al. 2006; Krampera et al. 2007) but also from adipose tissue (Krampera et al. 2007; Xu et al. 2008), spleen, and thymus (Krampera et al. 2007). MSC originating from various tissues have the capacity to trans-differentiate into a SC-like phenotype in vitro. Adipocyte- or bone marrow MSC-derived SC were shown to myelinate PC12 neurites in vitro (Keilhoff et al. 2006; Xu et al. 2008) and sustain axonal regeneration when implanted into a biogenic muscle graft to bridge a sciatic nerve gap (Keilhoff et al. 2006). Freshly isolated, purified, or CD133+ subpopulations of bone marrow cells were transplanted in various demyelinating conditions and reported to be associated with either SC or oligodendrocyte remyelination (Sasaki et al. 2001; Akiyama et al. 2002a, b). However, so far, there has not been a clear indication as to whether MSC contribute directly to the genesis of myelin-forming cells, or act simply via a by-standard effect on the host environment-promoting remyelination by host cells.
6.4.2 Skin-Derived Precursors
A number of studies have demonstrated the presence of multipotent cells in the skin that are capable of generating cells of neural, mesenchymal lineage and NC-derived cell types (Toma et al. 2001, 2005; Fernandes et al. 2004; Sieber-Blum et al. 2004; Amoh et al. 2005). The anatomical location and origin of these skin-derived precursors (SKP) was thoroughly investigated, and several “niches” have been identified mainly in the hair follicle. While some studies demonstrated that SKP were present in the hair follicle bulge (Sieber-Blum et al. 2004; Amoh et al. 2005; Wong et al. 2006), others identified the dermal papilla (Fernandes et al. 2004; Biernaskie et al. 2006; Wong et al. 2006) as the main source of SKP. However, the presence of multipotent cells in the human foreskin, a region devoid of hair follicles, highlighted the presence of an extra-follicular niche in the human skin (Toma et al. 2005). Of particular interest is the capacity of SKP to differentiate in vitro into neural crest-derived cell types such as SC (Fernandes et al. 2004, 2006). When rodent and human SKP were cultured with peripheral nerve explants, SKP proliferated and expressed myelin proteins (Fernandes et al. 2004). When grafted into the injured peripheral nerve or into the dysmyelinated neonatal shiverer brain, SKP differentiated into functional SC that associated and remyelinated CNS axons (McKenzie et al. 2006). Biernaskie et al. (2007) demonstrated convincingly that SC remyelination derived from an SKP graft resulted in functional recovery in a spinal cord contusion model.
Since one of the major issues of autologous cell transplantation therapy is the accessibility of the cell sources, the discovery, isolation, and characterization of SKP offer a large exploratory field of investigation for cell replacement. The recent finding that human bulge stem/precursor cells from adult skin can be isolated reproducibly and can undergo robust ex vivo expansion and directed differentiation raises hope for future cell-based therapies in regenerative medicine (Clewes et al. 2011).
6.4.3 Oligodendrocyte Precursor Cells
Until recently the possibility that SC can be generated from CNS neural precursors was not foreseen. However, this possibility has been raised by both in vitro and in vivo studies. In vitro, cloned CNS neural precursors generated oligodendrocytes and SC (Mujtaba et al. 1998). Moreover transplantation of neural precursors, glial-restricted precursors (Keirstead et al. 1999), and MOG-expressing oligodendrocytes precursors (Crang et al. 2004) in X-irradiated ethidium bromide lesions resulted in both PNS and CNS remyelination. Furthermore, transplantation of cloned human neural precursors, isolated from the adult human brain, into the same type of lesion led also to SC remyelination (Akiyama et al. 2001). These studies underlined an important role for the lesion environment and in particular for astrocytes in regulating the fate of CNS precursors since those that enter areas of demyelination differentiated into oligodendrocytes in the presence of astrocytes but in SC in their absence. More recent data highlighted a role for bone morphogenic protein (BMP) in repressing oligodendrocyte development (Ara et al. 2008) and directing CNS precursor into differentiation SC in vitro (Sailer et al. 2005). Grafted OPC differentiated preferentially into SC in presence of BMP (Talbott et al. 2006), thus suggesting a role for this morphogen in orienting the fate of immature populations when grafted in pathological conditions. While these data indicate that environmental cues can direct the differentiation of CNS neural stem cells into PNS cells, our recent findings suggest that the fate of PNS neural stem cells can also be redirected when forced by transplantation in the CNS. Our fate mapping studies indicate that when BC are grafted in the demyelinated spinal cord, some of these cells differentiate in remyelinating oligodendrocytes (Fig. 6.5) in addition to SC (Zujovic et al. 2010). Furthermore, when transplanted in the developing shiverer brain, BC acquire exclusively a CNS phenotype, giving rise to astrocytes, neurons, and oligodendrocytes (Zujovic et al. 2011). We demonstrated in vitro that this reprogramming was a multistep process depending on a sequential combination of ventralizing morphogens such as noggin, an antagonizer of BMP and Shh, and pro-oligodendroglial growth factors. Taken together, these studies indicate that CNS–PNS boundaries are not as clear-cut and CNS–PNS plasticity prevails in extreme pathological conditions.
6.5 Embryonic Stem Cells and Induced Pluripotent Stem Cells
While the main asset of embryonic stem cells is their capacity to give rise to all the cells of the body, their foremost limitation is the source of these cells. However, one of the major issues of autologous cell transplantation therapy resides in the accessibility of the cell sources. This is the reason why many studies focused on skin as the most accessible organ. Based on the modification of adult fibroblasts transduced into induced pluripotent stem cells (iPS) or directly reprogrammed into differentiated cells, the skin offers great future opportunities for cell replacement and regenerative medicine.
6.5.1 Embryonic Stem Cells
Since embryonic stem cells (ES) are totipotent cells, they can give rise to the myriad of cells that compose the body. While most research on ES differentiation has focused on CNS phenotypes, fewer studies have considered ES as potential source of PNS cells. Using diverse protocols and biological reagents, several research groups have succeeded in differentiating them in a wide range of NC lineage cells. Indeed, the production of SC from ES is a multistep process that begins with the induction of NC-like cells. The first group to successfully obtain NC-like cells used the stromal-derived inducing activity of PA6 fibroblast coculture (Pomp et al. 2005). When Lee et al. (2007) analyzed cells derived from ES neural rosettes more closely, they showed that NC precursors emerged spontaneously in culture of human ES cell-derived neural rosettes and that treatment with extrinsic patterning factors such as FGF2 and BMP 2 increased significantly the hES-NC number. Later, Studer and colleagues established a more defined protocol for neural induction based on the inhibition of SMAD signaling with the dual action of Noggin and SB431542 (Chambers et al. 2009). These protocols were later improved with different strategies using a neurosphere intermediate stage (Pomp et al. 2008), enriching hES-NC by fluorescence-activating cell sorting based on specific markers of the NC lineage cell expression (Lee et al. 2007, 2010; Zhou and Snead 2008; Jiang et al. 2009) treating the cells with small molecule inhibitor of Rho effectors (Hotta et al. 2009). However most of these strategies generated a wide range of NC-derived progeny including sensory and autonomic neurons, myofibroblast, adipocytes, cartilage, and osteogenic cells, but few demonstrated robust and efficient SC differentiation. Many studies report the presence of GFAP+, MBP+, and S100+ cells in the hES-derived culture (Lee et al. 2007; Hotta et al. 2009; Jiang et al. 2009) but only Ziegler et al. (2011) provided evidence of S100, MBP, and PMP 22 positive cells with apparent wrapping of the axons. Nonetheless the process of obtaining SC takes much longer than neuronal differentiation (Lee et al. 2007, 2010; Ziegler et al. 2011), corresponding to the developmental sequence of neurogenesis preceding gliogenesis. Indeed, the capacity of hES-NC to differentiate in SC increases with time in culture, likely uncovering a cell-intrinsic temporal change in their epigenetic state.
6.5.2 Induced Pluripotent Stem Cells
The clue of possible reprogramming of mature differentiated cells into totipotent cells came from the original experiment of Gurdon in 1960 (Gurdon 2006) when he transferred the nucleus of an adult cell into an enucleated egg and obtained totipotent cells capable of giving rise to a whole new frog. More than 40 years later, Takahashi and Yamanaka (2006) published their revolutionary data on transcription factor-based reprogramming of adult mouse fibroblasts. They isolated and identified a cocktail of four transcriptions factors Oct 4, Sox2, Klf4, and Myc representing the minimal set of factors required for reprogramming fibroblasts into pluripotent stem cells.
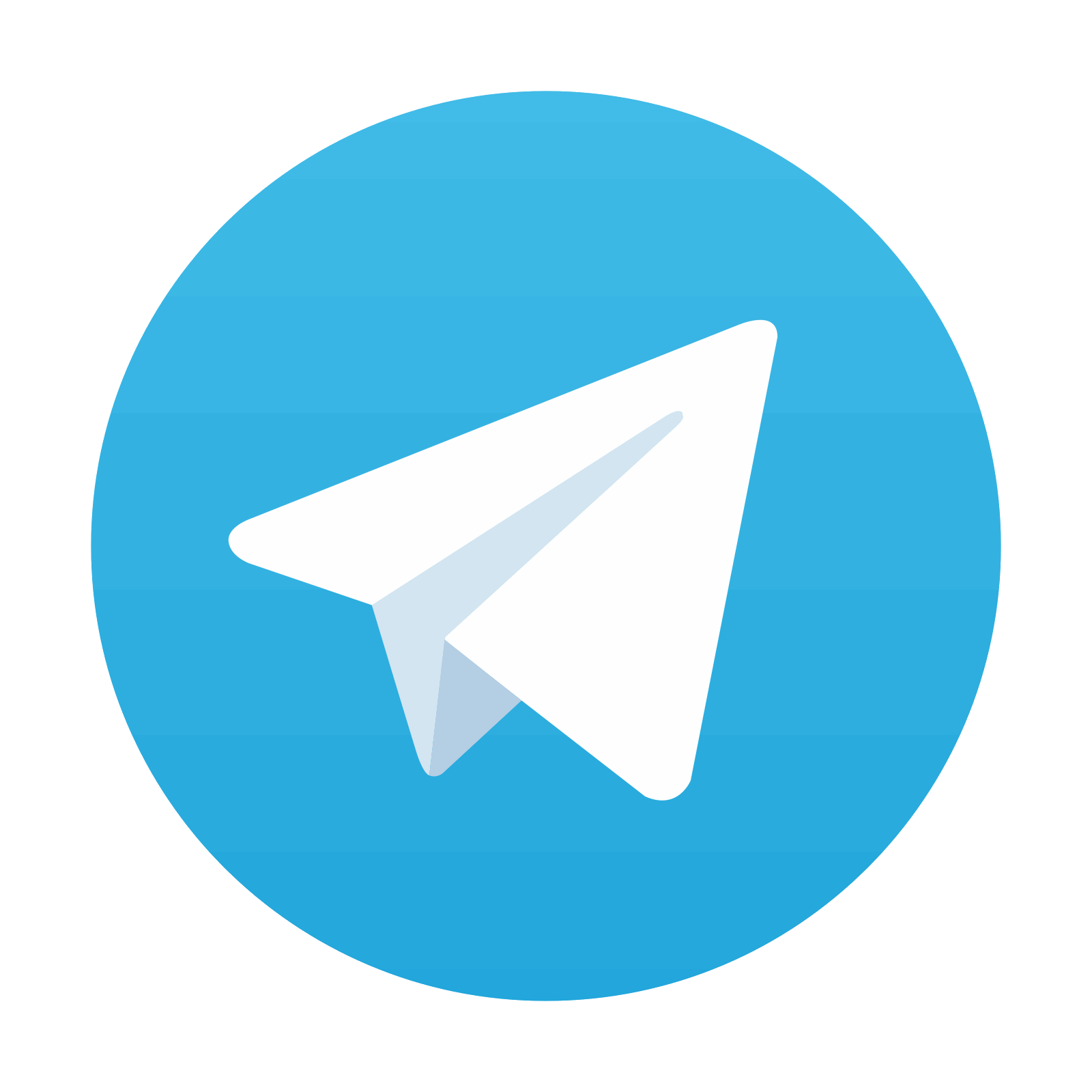
Stay updated, free articles. Join our Telegram channel

Full access? Get Clinical Tree
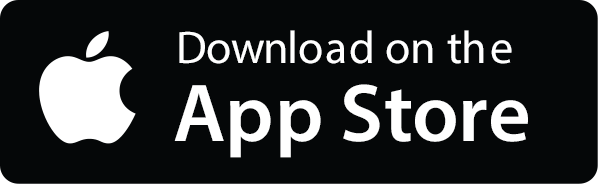
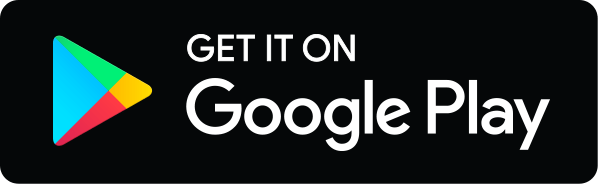