Reyhaneh Manafi‐Farid1, GhasemAli Divband2,3, HamidReza Amini3, Thomas G. Clifford4, Ali Gholamrezanezhad4, Mykol Larvie5, and Majid Assadi6 1 Research Center for Nuclear Medicine, Shariati Hospital, Tehran University of Medical Sciences, Tehran, Iran 2 Nuclear Medicine Center, Jam Hospital, Tehran, Iran 3 Khatam PET‐CT Center, Khatam Hospital, Tehran, Iran 4 Department of Radiology, Keck School of Medicine, University of Southern California, Los Angeles, CA, USA 5 Department of Radiology, Cleveland Clinic, Cleveland, OH, USA 6 Department of Radiology, School of Medicine, Nuclear Medicine and Molecular Imaging Research Center, Bushehr University of Medical Sciences, Bushehr, Iran The rising incidence and prevalence of cancer has led to a search within the field of nuclear medicine for new ways to control and treat the diseases. Various positron emission tomography (PET)‐tracers have been employed for functional imaging [1]. Currently, 2‐18F‐fluoro‐2‐deoxy‐D‐glucose positron emission tomography/computed tomography (18F‐FDG PET/CT) is the standard molecular imaging modality for many cancers. Nevertheless, FDG is a nonspecific metabolic marker with some limitations in certain malignancies, such as well‐differentiated neuroendocrine tumors, prostate adenocarcinoma, and mucin‐producing tumors. Therefore, targeted molecular imaging is pursuing new tracers for imaging and therapy that may overcome the drawbacks of earlier agents [2]. In nuclear oncology, the theragnostic concept is an established method for specific molecular targeting, in both diagnosis and therapy. The scintigraphic detection of targets can be considered as an indirect sign that the patient may benefit from specific treatments [3]. Modern precision oncology is based on tumor biology and thus relies on accurate analysis of the relationship between tumor cells and their microenvironment to understand the underlying tumor pathophysiology [4]. In addition to cancer cells, the tumor microenvironment consists of multiple different cell types, including inflammatory cells, lymphocytes, blood vessels, fibroblasts, and the extracellular matrix [5]. Therefore, this tumor microenvironment offers numerous potential targets for novel cancer drugs. One such target is cancer‐associated fibroblasts (CAFs), which are different from ordinary fibroblasts and abundant in the tumor microenvironment with potent effects on tumor development [6]. CAFs play a role in tumor growth and progression. A distinguishing feature of CAFs is their expression of fibroblast activation protein (FAP), a type II membrane‐bound glycoprotein [7]. FAP expression in healthy adult tissues is negligible but is highly upregulated with tissue remodeling, fibrosis, wound healing, inflammation, and cancers [8, 9]. FAP can be considered as a key co‐conspirator of cancer cells in tumor growth and metastasis [10]. Expression of FAP and its role in the majority of epithelial tumors encouraged scientists to employ it in oncologic imaging. However, early imaging with nonselective FAP antibodies was suboptimal, mainly due to their large size and long circulation time [11]. In recent years, several small‐molecule inhibitors of FAP (FAPIs) have been investigated for the diagnosis and therapy of various malignancies. A radiolabeled FAPI, recently introduced by Loktev et al. revealed acceptable characteristics for imaging, including rapid, specific, and stable uptake [12]. Also, imaging with radiolabeled FAPIs reportedly provides high contrast in tumors with rich stromal content, and they may be considered highly tumor‐specific [7]. FAP has been a target for imaging and treatment with different cold and radioactive FAP antibodies or inhibitors [13]. Here, we briefly describe the different subtypes of FAP inhibitors and their biodistribution. Moreover, we review the application of the novel promising imaging modality, radiolabeled‐FAPI PET, in nuclear oncology. Finally, we also discuss its potential role in nonmalignant conditions. Various radiolabeled FAPI subtypes have been used for PET imaging in preclinical and clinical settings, including, but not limited to, 68Ga‐labeled FAPIs. FAPI‐01 and FAPI‐02 have shown specific binding to human FAP with a rapid and almost complete internalization [7]. FAPI‐01 proved to be suboptimal due to enzymatic deiodination with efflux of the free iodine and consequently lower intracellular radioactivity after longer incubation intervals. FAPI‐02, a DOTA‐linked compound, demonstrated better pharmacokinetic and biochemical properties. FAPI‐02 eliminated more slowly than FAPI‐01 with approximately 10‐fold higher retention after 24 hours. Furthermore, a rapid internalization into FAP‐expressing cells has been reported with a high tumor uptake in patients with metastatic epithelial carcinomas [7, 14]. For optimization of tumor radiotracer uptake and retention, several FAPI‐02‐based compounds were investigated. Of synthesized FAPIs (FAPI‐02 to FAPI‐15), FAPI‐04 was the most promising radiotracer as a potential theragnostic agent [15]. Similar to FAPI‐02, FAPI‐04 showed rapid internalization into FAP‐positive tumors and fast clearance from the body, resulting in fast retention in lesions (in about 10 minutes after radiotracer injection). Furthermore, when compared to FAPI‐02, FAPI‐04 revealed 100% higher effective tumor uptake after 24 hours, which is more desirable for theragnostic application [15]. Several new compounds have been developed based on the lead compound FAPI‐04, which is characterized by rapid radiotracer uptake in FAP‐positive tumors followed by considerable background washout. Of these compounds, 68Ga‐FAPI‐46 displayed the highest tumor‐to‐blood, tumor‐to‐muscle, and tumor‐to‐liver ratios [16]. Biodistribution has been studied on the following 177lutetium‐labeled radiotracers: FAPI‐21, ‐35, ‐46, and ‐55. All of these compounds showed robust tumor accumulation with low radiotracer uptake by normal tissues. FAPI‐21 and ‐46 demonstrated higher tumor accumulation at 1 and 4 hours after injection in comparison to FAPI‐04 [16]. Depending on the tumor type, tumor accumulation can be significantly prolonged using FAPI‐46 [16]. Whereas earlier DOTA‐based tracers (FAPI‐02, ‐04, and ‐46) were developed with a focus on potential therapeutic applications, the NOTA‐derivate FAPI‐74 was developed as an exclusively diagnostic ligand, with slightly shorter tumor retention [17]. Recent analysis of biodistribution and dosimetry of 18F‐FAPI‐74 PET/CT and 68Ga‐FAPI‐74 PET/CT (by NOTA chelator ligand for 18F and 68Ga) in 10 lung cancer patients revealed high image contrast and low radiation burden. All currently available 68Ga‐labeled FAPI subtypes have renal excretion with activity in the urinary bladder and otherwise minimal background activity in normal tissues. Usually, the radiotracer is seen in the renal collecting system and urinary bladder without accumulation in the renal parenchyma [14]. Some researchers have reported dosimetry results of FAPI PET tracers. Giesel et al. performed the preliminary dosimetry for 68Ga‐FAPI‐02 and 68Ga‐FAPI‐04 in two metastasized breast cancer patients. PET/CT acquisition was performed at 10 minutes, 1 hour, and 3 hours after radiotracer injection. The effective total body dose was 1.80E‐22 mSv/MBq for 68Ga‐FAPI‐02 and 1.64E‐22 mSv/MBq for 68Ga‐FAPI‐04. The authors predicted that by injection of a dose of about 200 MBq, the effective dose from 68Ga‐FAPI PET/CT would be 3–4 mSv. The urinary bladder received the highest effective dose for both 68Ga‐FAPI‐02 and ‐04 [14]. The dosimetry of 68Ga‐FAPI‐46 was investigated by Meyer et al. in six different cancer patients. Serial imaging was performed at 10 minutes, 1 hour, and 3 hours after injection of 214–246 MBq of 68Ga‐FAPI‐46. The average effective total body dose was 7.80E‐03 mSv/MBq. Organs with the highest effective doses were the bladder wall (2.41E‐03 mSv/MBq), ovaries (1.15E‐03 mSv/MBq), and red marrow (8.49E‐04 mSv/MBq). The effective total body dose from 200 MBq of 68Ga‐FAPI‐46 was 1.56 ± 0.26 mSv. This, along with 3.7 mSv from low‐dose CT, leads to approximately 5.3 mSv total effective dose. They concluded that 68Ga‐FAPI‐46 PET/CT has a favorable dosimetry profile for imaging [18]. In summary, of the various FAPI tracers that have been developed, many of them have shown optimal imaging characteristics with favorable dosimetry. In particular, FAPI‐21 and FAPI‐46 seem to be the most appropriate agents for theragnostics. At present there are no special patient preparation recommendations prior to performing 68Ga‐FAPI PET/CT, such as fasting or discontinuing medications. However, adequate hydration is necessary for all agents with urinary excretion. Due to high and rapid renal excretion of most FAPI‐based PET radiotracers, intravenous hydration with 500 mL of normal saline and injection of 20 mg of furosemide from 15 minutes before to 30 minutes after radiotracer administration have been implemented [19]. 68Ga‐FAPI is injected intravenously but a definite injection activity has not been established. Authors have used the range of 100–370 MBq [14, 18, 19]. The appropriate injection activity is yet to be established for different scanners. In several clinical studies, especially for dosimetry purposes, different acquisition times for whole‐body 68Ga‐FAPI PET/CT scans were evaluated, mainly at three time points after radiotracer injection: 10 minutes, 1 hour, and 3 hours [14, 15, 18]. Early time‐point imaging at 10 minutes with 68Ga‐FAPI is possible, but it should be noted that the tumor‐to‐background contrast ratio improves with time [18]. Based on our experience, we orally hydrate patients and begin acquisition 30 minutes after injection of 200 MBq 68Ga‐FAPI. The overexpression of FAP in the microenvironment of many epithelial tumors is associated with a worse prognosis. Therefore, targeting this enzyme for molecular imaging and endoradiotherapy may be a novel strategy in cancer management [7]. 68Ga‐FAPI PET/CT with various FAPI ligands (which will all be referred to as FAPI below) has been used for targeting FAP in tumor stroma [20]. The first human studies were reported in 2018 [12, 15]. To date, multiple clinical studies have been performed to evaluate the potential efficiency of FAPI PET in clinical oncology. 68Ga‐FAPI PET/CT has been used to image various cancer types [14, 18], mostly in case series or case reports. In an early multicenter study, 68Ga‐FAPI uptake was evaluated in 28 different cancer types in patients (n = 80) [19], with suboptimal results from other imaging modalities. The result was promising, revealing remarkably high uptake and image contrast in several prevalent cancers such as breast, esophagus, lung, pancreatic, head–neck, and colorectal [19]. In another study, 75 patients with 12 different cancer types underwent imaging with 68Ga‐FAPI and 18F‐FDG PET/CT. Interestingly, the study showed that the detection rates of the primary tumor, lymph node, bone, and visceral metastases were significantly higher with 68Ga‐FAPI PET/CT [21]. Sarcoma, esophageal, breast, lung, pancreas, and primary liver cancers were among those with the highest uptake [19, 21]. In another recent study on 68 patients with inconclusive 18F‐FDG PET/CT, 68Ga‐FAPI PET/CT discriminated malignant lesions with an accuracy of 66.7% (12/18), localized primary cancer in 66.7% (4/12), detected tumor recurrence in 87.0% (20/23), and upstaged 33.3% (7/21) of patients [22]. When compared to 18F‐FDG, 68Ga‐FAPI showed higher intensity of uptake in most of the malignancies, providing higher contrast and tumor visualization [21–25], especially in gastrointestinal tract tumors such as primary esophageal cancer [23, 26]. In 35 patients with gastric, duodenal or colorectal cancer, Pang et al. demonstrated that the sensitivity and intensity of uptake were higher with 68Ga‐FAPI PET/CT for the primary lesions as well as lymph node, visceral, and bone metastases [24]. In the evaluation of hepatocellular carcinoma, 68Ga‐FAPI PET/CT detected seven out of eight, 15 out of 16, and 20 out of 20 tumors in three different studies [27–29]. Increased liver uptake was reported in cirrhosis [27, 28], although this apparently did not impact tumor visualization. The sensitivity of 68Ga‐FAPI PET/CT was reportedly higher than 18F‐FDG PET/CT (93 vs. 68%) and comparable with magnetic resonance imaging (93 vs. 100%) for the detection of primary liver malignancies [28]. Also, 68Ga‐FAPI PET/CT detected all (7/7) cholangiocarcinomas in contrast to 18F‐FDG PET/CT (4/7) [28]. Intense uptake in cholangiocarcinomas has already been reported in other studies [19, 21, 29]. When compared to 18F‐FDG PET/CT, Zhao et al. reported higher sensitivity (97.67 vs. 72.09%) and intensity of uptake (median standardized uptake value [SUV] 9.82 vs. 3.48) for 68Ga‐FAPI PET/CT in peritoneal carcinomatosis in patients with various cancers, particularly gastric (20/35) [30]. On the contrary, Ballal et al. demonstrated that the intensity of uptake and the detection rate of 68Ga‐FAPI and 18F‐FDG PET/CT were comparable in various malignancies, particularly breast and lung cancers [2]. They also reported lower intensity of 68Ga‐FAPI uptake in lymph nodes [2]. Differences in inclusion criteria may explain this discrepancy as most of the patients included in the aforementioned studies had malignancies with a known limited role of 18F‐FDG PET/CT. Finally, in nasopharyngeal cancer, results have been somewhat inconclusive. Compared to 18F‐FDG PET/CT, Zhao et al. (n = 45) reported higher intensity of uptake in primary tumors, regional lymph nodes, and bone and visceral metastases [31], whereas a study by Qin et al. (n = 15) showed lower SUVmax of primary tumors and metastatic lymph nodes [32]. The number of detected lymph nodes was also noted to be lower with 68Ga‐FAPI PET/MRI by Qin et al. [32]. It is clear that cancer staging and treatment algorithms hinge on imaging. 68Ga‐FAPI PET/CT has altered stage and management in some cancers. Detecting further metastases as new findings in 47% (10/21) of patients, Koerber et al. demonstrated a change in the management in 19% (4/21) of patients compared to standard imaging in lower gastrointestinal cancer [33]. The TNM stage was altered in three out of six treatment‐naïve patients [33]. When compared with CT in pancreatic cancer (n = 19), Röhrich et al. showed that 68Ga‐FAPI PET/CT changed stage in nine out of 12 and one out of seven with recurrent/progressive and newly diagnosed disease, respectively, while management was altered in seven out of 19 patients [34]. In nasopharyngeal cancer (n = 39 treatment naïve), 68Ga‐FAPI PET/CT upstaged 10 patients in 26% and altered the management in seven (18%) when compared with 18F‐FDG [31]. Also, when compared with MRI, T‐stage was upgraded in four (10%) and underestimated in two (5%) patients [31]. In another study, 68Ga‐FAPI PET changed stage in six out of 15 patients (three upstaged and three downstaged) [32]. 68Ga‐FAPI PET/CT was also used for the assessment of treatment response in a case of esophageal cancer. It showed reduced uptake in the tumor 2 weeks after chemoradiotherapy, while new regions of uptake were noted in the lungs as the result of radiation pneumonitis [35]. It was also used before and after cytoreductive surgery of peritoneal carcinomatosis to evaluate the successfulness of the procedure [36]. Additionally, 68Ga‐FAPI PET/CT has successfully detected disease recurrence in multiple studies with a reported sensitivity of 87.0% in one study [22] and more true positive lesions compared to 18F‐FDG PET/CT in another [31]. Finally, there are case reports of 68Ga‐FAPI uptake in primary extranodal (gastric, brain) lymphoma [37, 38], extramammary Paget disease [39], neuroendocrine tumor [40], cardiac angiosarcoma [41], chromophobe renal cell carcinoma [42], and a ground‐glass lung adenocarcinoma [43]. FAP is expressed minimally or not at all in normal cells [12]. Therefore, imaging with FAPIs provides absent or low background activity. When compared with 18F‐FDG, this feature is particularly useful for detection of malignant lesions in the brain and liver due to its higher contrast [2, 12, 44]. FAP is also an encouraging tracer in the evaluation of small lesions such as peritoneal carcinomatosis [21, 30, 45] and leptomeninges [46]. Since even tiny tumors require supporting stromal tissue, it is hypothesized that tumors as small as 3–5 mm could be detected with FAPI PET [12]. Moreover, the uptake of 68Ga‐FAPI is independent from the blood glucose level, which has important implications for patient preparation and particular utility in diabetic patients (Figure 30.1) [47]. Figure 30.1 A 69‐year‐old woman with recurrent cholangiocarcinoma underwent 68Ga‐FAPI PET/CT. Because of uncontrollable diabetic hyperglycemia, 18F‐FDG PET/CT could not be performed. The images revealed an intensely FAPI‐avid (SUVmax, 24.5) soft tissue mass in the Whipple surgical bed along with several mildly FAPI‐avid small peri‐pancreatic lymph nodes and adjacent peritoneal nodules. A 68Ga‐FAPI–avid (SUVmax = 9.3) myoma was also detected in the uterus.
30
68Ga‐FAPI, a Twin Tracer for 18F‐FDG in the Era of Evolving PET Imaging
Introduction
FAPI Subtypes, Biodistribution, and Dosimetry
Imaging Protocol
Oncologic Application
Imaging
Advantages
Stay updated, free articles. Join our Telegram channel

Full access? Get Clinical Tree
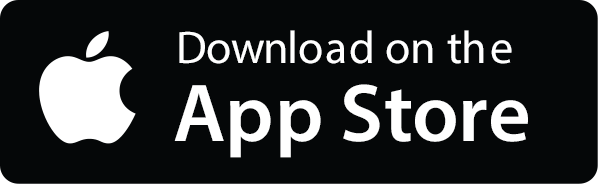
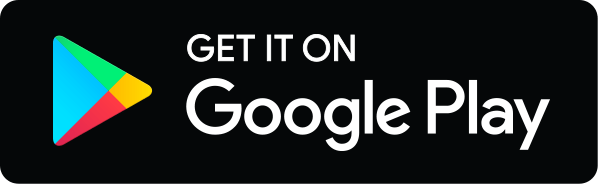