Shabnam Mortazavi1, Sonya Khan2, Kathleen Ruchalski1, Cory Daignault3, and Jerry W. Froelich4 1 Radiology, David Geffen School of Medicine at UCLA, Los Angeles, CA, USA 2 Los Angeles and Veterans Administration, Greater Los Angeles Healthcare Systems, University of California, Los Angeles, CA, USA 3 Minneapolis VA Medical Center, Minneapolis, MN, USA 4 Radiology, University of Minnesota, Minneapolis, MN, USA The breast is a modified cutaneous gland located within the superficial pectoralis fascia, overlying the pectoralis major muscle superiorly, serratus anterior muscle laterally, and upper abdominal oblique muscles inferiorly. Adult female breast tissue typically extends from the second to seventh ribs, bounded by the sternum medially and midaxillary line laterally. Breast parenchyma consists of glandular, adipose, and connective tissue. A typical female breast consists of approximately 15–20 segments demarcated by lactiferous ducts converging at the nipple in a radial configuration. The functional unit of the breast is the terminal ductal lobular unit (TDLU). Each TDLU comprises a lobule and terminal duct connecting the TDLU to a lactiferous duct (Figure 12.1). Blood flow to the breast is typically supplied by the internal mammary, lateral thoracic, and intercostal arteries. Venous drainage of the breast parallels the arterial anatomy at the level of the deep breast tissues; however, superficial draining veins do not follow the path of arterial blood supply. Typical regional lymphatic drainage of the breast includes the axillary, supraclavicular, and internal mammary nodal chains. Axillary lymph nodes are subdivided into three named levels, defined in relation to the pectoralis minor muscle. Level I (low axillary) nodes are lateral to the muscle, level II (mid axillary) nodes are deep to the muscle, and level III (high axillary) nodes are superior and medial to the muscle (Figure 12.2). The three major breast lymphatic drainage routes are axillary, interpectoral, and internal mammary. Breast cancer is the most common cancer in women and about one out of eight women (roughly 12%) in the United States will develop invasive breast cancer in their lifetime [1]. The term “breast cancer” encompasses a heterogenous disease process, subclassified and described clinically by histopathology, biomarkers, and genomic profile. Histopathological subtypes: Breast carcinomas arise from the epithelial component of either lobules or the terminal duct. There are many different types of breast cancer [2]. The most common histopathological subtype (approximately 80%) is invasive ductal carcinoma, followed by invasive lobular carcinoma (20%). Biomarker subtypes: Currently, there are three major clinically measured biomarkers: estrogen receptor (ER), progesterone receptor (PR), and human epidermal growth factor receptor 2 (HER2) expression. Accordingly, there are three clinical biomarker‐based subclassifications of breast cancer: (ER and/or PR)‐positive and HER2‐negative, HER2‐positive, and triple‐negative. Approximately 70% of breast cancers are hormone receptor positive [3]. Molecular subtypes: There are currently four distinct, clinically diagnosed molecular subtypes of breast cancer, classified on the basis of gene‐expression analysis and hormone receptor status: luminal A, luminal B, HER2‐enriched, and triple‐negative/basal‐like tumors [4]. Figure 12.1 (a) Lateral ductogram mammographic images obtained immediately following intraductal contrast administration via cannulation of a single periareolar lactiferous duct, illustrating the extent of a single lactiferous duct. A terminal ductal lobular unit (TDLU) is depicted (b). Figure 12.2 (a) Axillary lymph nodes used to determine N category according to the 8th AJCC Cancer Staging Manual. (b–g) Axial FDG PET‐CT images demonstrate FDG‐avid and/or enlarged (b) right axillary level I node, seen lateral to the pectoralis muscles (arrow), (c) left axillary level II lymph nodes (arrow), deep to the pectoralis minor muscle, (d) a left axillary level III lymph node (arrow), medial and superior to the pectoralis minor muscle, (e) left (arrow), greater than right, internal mammary chain lymph nodes in a patient with mediastinal and axillary level I lymphadenopathy, (f) a right chest wall/intramammary lymph node (arrow), and (g) a left interpectoral (Rotter) lymph node (arrow), by definition located between the pectoralis major and minor muscles in a patient with enlarged left axillary level I and II and neck lymph nodes. A general familiarity with the highly regulated and algorithmic paradigm of conventional breast imaging, which centers on the diagnosis and sequelae of breast cancer, and an understanding of the varied and crucial tasks nuclear imaging examinations fulfill within this complex system are essential for clinically meaningful correlation between molecular and nonmolecular imaging modalities. The term “breast imaging” encompasses multiple imaging modalities. Detailed guidelines for the clinical uses of these modalities are outlined in the American College of Radiology Appropriateness Criteria and Practice Guidelines [5]. Conventional mammography and digital breast tomosynthesis (DBT) utilize ionizing radiation, captured on a detector, to depict breast anatomy in multiple orthogonal or quasi‐orthogonal imaging planes, with the goal of identifying/localizing alterations in typical anatomy suspicious for malignancy. DBT uses a moving X‐ray source to image the breast at different angles, obtaining multiple digital images that can be reconstructed to obtain a quasi‐tridimensional (3D) representation of the breast and allowing overlapping breast tissue to be viewed separately, increasing breast cancer detection and decreasing the false‐positive rate, particularly in women with radiographically dense breast tissue [6]. As the relative amount of fat decreases and glandular tissue increases, the breast becomes denser on mammography and cancer detection becomes more challenging. Figure 12.3 demonstrates quasi‐orthogonal, standard mediolateral oblique (MLO), and craniocaudal (CC) screening mammography views as performed for the purposes of localizing breast findings to one of four quadrants via triangulation. These standard image views have been adopted for dedicated molecular breast imaging (MBI), as discussed later, and can be used to localize MBI findings as well, as in the case of the right breast findings shown in Figure 12.3. Figure 12.3 Right breast craniocaudal, aka CC (a), and mediolateral oblique, aka MLO (b), standard screening mammogram views in a high‐risk patient demonstrates heterogeneously dense breast tissue. The parenchymal pattern was stable from previous mammograms, without mammographic evidence for malignancy. Subsequent imaging with MBI in the CC (c) and MLO (d) projections demonstrates mass‐like uptake in the lower outer quadrant that yielded invasive lobular carcinoma on tissue sampling. Source: Reprinted from Huppe et al. [7], with permission from Elsevier. Breast ultrasound is a preferred modality for image‐guided breast procedures and has been investigated as a breast cancer screening modality [8]. Dynamic, gadolinium‐based contrast‐enhanced magnetic resonance imaging (MRI or CE‐MRI) is the most sensitive imaging tool for detection of breast cancer, providing functional physiologic data via dynamic contrast enhancement analysis in addition to superb anatomic detail due to MRI’s high soft tissue contrast resolution, in some cases detecting cancer occult on clinical examination or conventional modalities (mammography and ultrasound). Breast MRI utilizes magnetic fields (no ionizing radiation) to create multiplanar cross‐sectional images through the selected field of view, requiring a dedicated breast coil to obtain images of diagnostic quality while the patient lies in the prone position with both or one breast(s) hanging free in the coil cavity. Dedicated breast positron emission tomography systems (dbPET), discussed later, employ similar prone positioning. Intravenous gadolinium‐based contrast agents are needed to reliably detect cancers, extent of malignant disease, and some common benign lesions. Additional physiological data is obtained through kinetic analysis of the rate of contrast uptake and washout by the background breast parenchyma and any lesions of interest, a process that is typically performed by the interpreting physician at a separate workstation using specialized post‐processing software. Analogous kinetic analysis techniques have been devised and are being studied for the relative rate of various nuclear imaging radiotracer uptake, particularly FDG, in the setting of potentially malignant lesions, including breast lesions. One relative advantage to CE‐MRI is the relative swiftness of gadolinium washout from target lesions and the background compared to 18F‐fluorodeoxyglucose (FDG), allowing the overlay of both the vascular uptake and washout characteristics of lesions to be studied with CE‐MRI. Breast MRI can be used to supplement screening mammography in women with an increased risk for breast cancer, evaluate extent of disease in selected cases, and monitor response to systemic therapy in the neoadjuvant setting [9]. In selected cases, it may be used for problem solving. Figures 12.10, 12.14, 12.16, and 12.17 depict clinical examples of breast MRI. MBI, a term that includes breast‐specific gamma imaging (BSGI), positron emission mammography (PEM), and dbPET, is a physiologic approach to breast imaging, performed following intravenous injection of radiopharmaceuticals, and imaged with dedicated single‐photon gamma imaging or annihilation coincidence detection systems (i.e. PET‐CT). Radiopharmaceutical uptake is dependent on physiologic factors, such as increased vascularity (blood flow) and mitochondrial activity/concentration in malignant cells, resulting in images that depict functional activity localizing to malignant, and a subset of benign, breast lesions [7]. Molecular imaging techniques (BSGI, PEM, dbPET) therefore are not affected by anatomic characteristics, such as breast density or postsurgical distortion, unlike mammography or ultrasound, but do suffer from lower anatomic resolution and precise localization. Imagers first recognized the potential for molecular imaging of breast cancer in the 1970s, when single‐photon‐emitting radiopharmaceuticals administered for bone and cardiac imaging were noted to incidentally localize to breast malignancies [10–12], resulting in the development of scintimammography, a term referring to single‐photon‐emitting radiopharmaceutical breast imaging performed with scintillation gamma cameras. Despite early promise, researchers found that early planar and SPECT scintimammography techniques, performed with large field‐of‐view whole‐body detectors, had low sensitivity for detection of small (<1.5 cm), nonpalpable, yet still clinically relevant breast lesions. The low sensitivity of these early MBI systems was a result of detector size, shape, and distance from the breast, limited field of view, and limited intrinsic spatial resolution [13–17]. As a result, clinical use of initial MBI techniques had been largely abandoned by the late 1990s. Advances over the past 20 years have led to the development of dedicated molecular breast‐imaging systems, both single‐photon gamma cameras and coincidence‐detection systems, offering improved detection of subcentimeter breast lesions [11, 18], opening the door to a new wave of clinical MBI research and a second chance for molecular imaging to establish utility in clinical breast cancer detection and evaluation. Notably, in addition to improved resolution and sensitivity for small breast lesions, these dedicated breast systems provide the ability to perform variations of lateral and CC image projections comparable to radiographic mammography, enabling ease of comparison, and the potential for meaningful integration and correlation with conventional breast‐imaging practices [19]. Several compact gamma cameras have been developed over the past two decades, including the use of cadmium zinc telluride semiconductor detectors and dual‐head configurations with breast compression (analogous to radiographic mammography), which provide substantially improved spatial resolution compared to conventional gamma cameras. Innovations responsible for improving the spatial resolution of these systems include use of pixelated arrays of small detector elements, improved spatial discrimination of the electronics coupled to the detector elements, exclusion of nearby organs with avid physiologic radiopharmaceutical uptake from the field of view, and reduction of the lesion‐to‐detector distance via direct contact with and mild compression of the breast [20, 21]. Current, commercially available detector configurations have been shown to reliably detect subcentimeter cancers with sensitivity and specificity comparable to mammography [19, 22–24] Initially, the dose of 99mTc‐sestamibi prescribed for reliable detection was approximately 20 mCi (740 MBq), considered too great an exposure for screening purposes [25]. However, as the sensitivity of gamma cameras has improved by modifying collimators and optimizing acquisition parameters and proximity of the imaged breast [26, 27], there has been a reduction in the necessary prescribed dose of 99mTc‐sestamibi to approximately 8 mCi (296 MBq) [25, 26], with some institutions reporting a prescribed dose range as low as 5–10 mCi while maintaining clinically reliable lesion detection [26, 28]. Although guidelines have been suggested, no particular patient preparation has been found necessary [7]. Absolute contraindications are pregnancy and allergy to the radiotracer (which is extremely rare). In a patient with known breast cancer, the injection should be performed in the contralateral arm to avoid lymphatic uptake from extravasation of the radioisotope during injection, as this will be imaged as axillary uptake and can be mistaken for pathological lymphatic involvement [29]. The examination is performed with the patient comfortably seated, in gentle breast compression during image acquisition, which typically begins immediately after radiotracer administration, continuing for approximately 175 000 counts, requiring approximately 5–10 minutes per image view. Imaging in projections comparable to radiographic mammography allows for multimodality correlation. The radiotracer is taken up by the breast almost immediately following injection, with relatively constant activity for the duration of the 40‐ to 50‐minute study, therefore additional radiotracer administration is not necessary prior to obtaining additional images. Additional images can be acquired as deemed necessary by the interpreting physician and may include exaggerated CC, true lateral, or, in the case of a single detector camera, imaging with the detector on the opposite side of the breast to bring the lesion of interest closer to the detector. “Second‐look” mammography may be performed to identify MBI‐detected lesions with subtle mammographic findings that may not have been appreciated before MBI examination [7] (Figure 12.4). Figure 12.4 (a) MBI performed in this patient with a known malignancy in the contralateral breast demonstrates a focus of abnormal uptake in the central right breast. (b) “Second‐look mammography” demonstrates a corresponding asymmetry seen when the nipple is in profile. Stereotactic biopsy of the asymmetry yielded atypical ductal hyperplasia, which was upgraded to DCIS on excision. (c) Molecular breast imaging in this patient with known right breast cancer demonstrates an abnormal focus of uptake in the medial left breast for which second‐look ultrasound was recommended. (d) Second‐look ultrasound demonstrates an irregularly shaped hypoechoic mass corresponding to the MBI finding. Biopsy yielded invasive ductal carcinoma. Source: Reprinted from Huppe et al. [7], with permission from Elsevier. Several compact breast‐specific PET systems, referred to as PEM or dedicated breast PET (dbPET), have been developed to overcome the limitations of conventional whole‐body PET scanners [30]. Breast‐specific PET system designs incorporate substantially smaller detector separation and use smaller crystals compared to conventional PET, resulting in substantially improved spatial resolution. Several dedicated breast‐imaging systems for positron‐emitting radiopharmaceuticals have been designed [20, 31], differing by geometry and mobility of the detectors (stationary flat, rotating flat, stationary full or partial ring) and positioning/immobilization of the imaged breast [32]. PEM is performed with the patient seated upright in mild compression [33]. Images are obtained in the same projections as mammography, facilitating correlation of imaging findings, with the possibility of direct co‐registration in some system designs. Alternatively, dedicated breast PET (dbPET) is performed with the patient lying prone with breasts hanging freely through a small ring of detectors, similar to positioning with breast MRI [34]. The increased spatial resolution of dbPET systems translates to considerably improved tumor‐to‐background (TBR) standard uptake value (SUV) ratios when compared to conventional whole‐body FDG PET/CT [35] (Figure 12.5). Image acquisition is performed approximately 60–120 minutes following a standard whole‐body FDG dose and proceeds for approximately 20 minutes per breast (10 minutes each in the CC or MLO projection, respectively) for PEM systems and for approximately 5–15 minutes per breast for dbPET systems [20, 32, 36]. In menstruating women, MBI is ideally performed during the follicular phase of the menstrual cycle (days 7–14), the goal being to minimize physiologic background parenchymal radiotracer uptake, analogous to the background parenchymal enhancement seen on breast MRI. Figure 12.5 Peripheral and nonperipheral breast cancer images of dbPET scanned in the prone position and whole‐body PET/CT scanned in the supine position. Representative clinical images of peripheral (39‐year‐old woman, clinical size 12 mm, a–c) and nonperipheral (63‐year‐old woman, clinical size 9 mm, d–f) breast cancers on dbPET (a, d), PET/CT (b, e), and PET (c, f) with focal FDG uptake in the background mammary gland tissue. Although the focal uptakes were visualized on both the dbPET and whole‐body PET/CT images, it was more obvious on dbPET with a higher TBR than on whole‐body PET/CT, regardless of the peripheral or non‐peripheral location. Source: Reprinted from Satoh et al. [35], with permission from Springer Nature (Creative Commons). One dilemma in interpretation and correlation of multimodality breast‐imaging findings is the inevitable difference in breast tissue orientation due to changes in patient positioning among the various imaging modalities: prone for breast MRI, supine for breast ultrasound, and upright with varying degrees of compression and obliquity for mammography. Molecular breast‐imaging techniques offer an added advantage in allowing for breast positioning that corresponds to the conventional breast‐imaging modalities: prone for dbPET, supine for whole‐body FDG PET‐CT, and upright with mild compression for BSGI and PEM. Developed and periodically revised by international experts in breast imaging, in conjunction with the American College of Radiology (ACR), the breast‐imaging reporting and data system (BI‐RADS) lexicon provides a framework for standardized reporting of breast‐imaging finding descriptions, assessments, and recommendations, improving communication between providers through a common language and providing for high‐quality patient care through interpreting physician audit and patient outcome tracking. The current edition (5th, published in 2013) provides guidelines for mammography, breast ultrasound and breast MRI [37]. Interpretation and reporting of molecular breast‐imaging modalities (BSGI, PEM, and dbPET) are not specifically addressed by the current BI‐RADS lexicon, although a corresponding MBI lexicon has been developed and proposed as a means of standardizing reporting and facilitating correlation between imaging modalities [38, 39]. Table 12.1 provides a summary of the BI‐RADS descriptor categories, recommendations, and applicable malignancy risk. With the implementation of dedicated breast‐imaging systems, MBI now has potential clinical utility in both screening and diagnostic populations. Current, clinically available BSGI and PEM imaging systems and protocols have demonstrated markedly improved sensitivity and specificity for detection of breast cancer, comparable to conventional imaging modalities, with the notable exception of ductal carcinoma in situ (DCIS) [40–42]. In women with dense breasts, MBI demonstrates improved sensitivity compared to radiographic mammography, improved specificity compared to breast ultrasound, and overall similar performance compared to breast MRI [8, 43–45]. Screening mammography, the only breast‐imaging modality proven to reduce mortality in randomized trials [46], is performed in asymptomatic women at periodic intervals with recommendations available from the National Comprehensive Cancer Network (NCCN), the US Preventive Services Task Force, and the American Cancer Society. Despite recent advances, including administered dose reduction [26, 28, 47] MBI results in a significantly higher effective whole‐body radiation dose (approximately 2.5 mSv for 8 mCi 99mTc‐sestamibi) compared to conventional digital mammography (approximately 0.5 mSv for a two‐view digital mammogram). Accordingly, the ACR does not recommend BSGI, PEM, or dbPET for routine breast cancer screening [5]. Adjuvant screening with breast ultrasound and/or MRI has shown increased detection of breast cancer in select subgroups of women [8, 48], including those with dense breast tissue, which decreases the sensitivity of screening mammography. The addition of BSGI to screening mammography has been shown to significantly increase the sensitivity and cancer detection rate in women with dense breasts [25] (Figure 12.3). Table 12.1 BI‐RADS assessment categories and recommendations. n/a, not applicable. Diagnostic breast imaging is a tailored imaging evaluation of specific clinical findings/symptoms or an imaging abnormality detected at screening. A notable indication for MBI is problem solving for indeterminate cases or challenging clinical situations, including cases that require additional evaluation following complete mammographic and sonographic evaluation. A common example is invasive lobular carcinoma, which is characteristically occult clinically and on conventional mammography and ultrasound imaging. Available studies suggest that MBI may have a significantly greater sensitivity in detecting invasive lobular carcinoma compared to mammography, ultrasound, or even MRI [49, 50]. Additionally, certain clinical situations, such as women with implants or direct silicone injections for augmentation, present a diagnostic challenge when imaged with mammography, ultrasound, and even MRI (Figure 12.6). MBI can also be used to assess for primary breast cancer in patients with metastases or metastatic axillary lymphadenopathy of an unknown primary. MRI is indicated to screen women with a greater than 20% lifetime risk of breast cancer [9] and as a powerful diagnostic problem‐solving tool. However, approximately 15% of patients are unable to undergo evaluation by MRI due to implantable devices/metallic foreign bodies, body habitus, renal insufficiency, patient positioning, and claustrophobia. In women unable or unwilling to undergo MRI, MBI serves as an alternative form of physiologic breast imaging for detection of lesions not visible on mammography or ultrasound, determining extent of disease, and monitoring post‐treatment response, disease recurrence, and high‐risk surveillance. Studies comparing MBI to breast MRI have shown that MBI has a comparable high sensitivity and cancer detection rate, with possibly a higher specificity than breast MRI [25, 28, 51–53]. Notably, the high sensitivity of MBI is independent of breast density [54]. In the context of preoperative staging, contrast‐enhanced MRI, with its increased contrast resolution and sensitivity compared to mammography or ultrasound, has been shown to detect additional malignancy in 20% of patients, including 5% in the contralateral breast [55]. Findings to date suggest that MBI is not adversely affected by post‐therapeutic changes of the breast, presenting a valuable alternative to mammography, ultrasound, or MRI in post‐treatment patients [56]. Figure 12.6 (a) Screening mammogram in a patient with a history of silicone injections demonstrates innumerable injection granulomas significantly limiting evaluation for malignancy. (b) MBI in this patient demonstrates no abnormal uptake. Source: Reprinted from Huppe et al. [7], with permission from Elsevier. Image‐guided biopsies are utilized for both nonpalpable, image‐detected findings and palpable findings to direct the needle to the most suspicious portion of a lesion. Ultrasound guidance is the preferred and overall most commonly utilized modality, followed by stereotactic and, rarely, MRI‐guided biopsies. Recently, MBI‐guided biopsy techniques (99mTc‐sestamibi‐guided and high‐resolution PEM‐guided biopsies) have emerged as safe and effective adjuvants to ultrasound‐guided or stereotactic biopsy and potential alternatives to MRI‐guided biopsy [57]. Performed with the patient upright and in gentle compression, the target lesion is optimally positioned and images are obtained from multiple angles to determine the depth of the lesion [58] (Figure 12.7). An average procedure time of approximately 30 minutes has been reported for PEM‐guided techniques [59]. Studies of 99mTc‐sestamibi‐guided biopsies have demonstrated positive predictive values comparable to what has been reported for MRI‐guided biopsy [60]. For all image‐guided breast biopsies, it is recommended that metallic markers or microclips be placed at biopsy sites following sampling to facilitate subsequent follow‐up and/or excision [61]. Table 12.2 provides a comparison of the significant differences and advantages and limitations of MBI‐guided and other common image‐guided breast biopsy methods. Figure 12.7 Procedure steps of 99mTc‐sestimibi MBI‐guided breast biopsy using the GammaLoc® stereotactic localization system. Source: Collarino et al. [57] / Springer Nature / CC BY 4.0. Table 12.2 Comparison of image‐guided biopsy modalities. Source: Modified from Collarino et al. [57]. First used for penile cancer staging in 1976 by Dr. Cabanas, this technique is driven by the concept of a sentinel lymph node (SLN) and the assumption that if the first loco‐regional lymph node to drain from the target malignant lesion/region has no histopathologic evidence of metastatic disease, then the remaining loco‐regional lymph nodes must also be disease free [62]. Noninvasive, imaging assessment of axillary lymph nodes is challenging. Axillary lymph nodes can appear abnormally enlarged in a number of physiologic and nonmalignant disease processes, including reactive inflammation, which can occur in the setting of breast cancer in the absence of nodal metastasis. Normal lymph nodes are highly vascular at baseline, limiting the diagnostic usefulness of vascular flow data obtained on contrast‐enhanced, ultrasound, or MRI evaluation. As a result, the overall sensitivity and specificity of current ultrasound and MRI techniques for detection of lymph node tumor metastases is highly variable [63–66], without sufficient negative‐predictive value to reliably exclude microscopic metastases and provide definitive staging in the setting of breast cancer. Thus, histopathologic sampling at time of surgery continues to be necessary and the standard of care for determining axillary involvement in breast cancer patients. Sentinel lymph node biopsy (SLNB) performed at time of surgery is now the preferred surgical staging technique when clinically indicated. Prior to SLNB, local surgical staging was performed solely by axillary lymph node dissection (ALND), a nonspecific technique involving extensive removal of axillary lymph nodes, associated with considerable post‐procedure morbidity, including upper extremity lymphedema in up to 25% of patients [67]. In contrast, SLNB reduces the number of lymph nodes harvested to just those most likely to harbor metastatic disease, typically two to five nodes, without negatively impacting disease‐free survival or overall survival [68–70]. The American Society of Clinical Oncology recommends SLNB in patients with early breast cancer (T1 and T2) with clinically negative nodes and in patients with DCIS with planned mastectomy. SLNB can be performed for patients with a history of prior excisional biopsy without impairing diagnostic accuracy [71]. Despite the higher associated morbidity, ALND is indicated in certain clinical situations, including locally advanced (T4a, b, c) or inflammatory breast cancer, or following SLNB, when SLNB yields three or more positive lymph nodes in T1 or T2 disease, any number of positive lymph node(s) in T3 disease, and any number of positive lymph node(s) with histopathologic evidence of extranodal extension of tumor cells [72–75]. Intraoperative SLN localization is performed by the breast surgeon using a handheld gamma probe. SLNB is typically done in conjunction with intraoperative blue dye injection to aid intraoperative SLN visualization. There are many available axillary lymphoscintigraphy radiotracers available worldwide, among them 99mTc sulfur colloid and 99mTc tilmanocept, which are the most commonly used in the United States. The goal of any SLNB injection is to accurately map the progressive route of lymphatic drainage originating from a target lesion or tissue of interest. This can be done indirectly via regional dermal injection or directly via peritumoral injection. Although generally both techniques are considered clinically equivalent, there are a few important distinctions between the two methods. The dermal injection technique deposits tracer in the dermis and broadly includes routes described as periareolar, subareola, intradermal, and subdermal. A successful intradermal injection should create a small skin wheal at the site of injection, which is usually periareolar with this technique. While injection can be performed elsewhere in the breast, such as in the skin overlying the tumor, those injections tend to have a longer transit time to the axilla than the periareolar injections [76]. Furthermore, dermal injection in the upper outer quadrant can potentially confound intraoperative SLN localization with a gamma probe due to proximity of the injection site to the axilla. Studies have shown strong concordance between different routes of dermal injection for the identification of an axillary SLN [77]. However, dermal injections rarely drain to the internal mammary chain [77]. Early studies favored peritumoral injections as these were thought to better reflect the lymphatic drainage channels available to malignant cells; however, the key technical challenges of this method can produce false negative results. Studies have shown that lesions located in the deep breast and lesions in patients with small breasts can preferentially drain to the internal mammary lymph nodes (IMLN) rather than the axillary nodes [78], potentially resulting in false‐negative SLNB if only the axilla is sampled. When peritumoral injections are used in isolation, they are associated with a higher axillary false‐negative rate and slower lymphatic drainage than with isolated dermal injection [79]. Some centers utilizing peritumoral injections do so with a high‐dose 2‐day protocol to allow ample time for lymphatic drainage. When performed, the operator should carefully review any mammographic imaging available to guide the injections, which is typically performed in four aliquots. As neoplasms are not directly connected to the body’s lymphatic system, a misplaced injection inside the tumor may result in little to no drainage of radiotracer. Similarly, injection directed into an adjacent postoperative or post‐biopsy seroma would have minimal to no lymphatic drainage. As anatomic imaging guidance is not typically performed during radiotracer injection, this failure to migrate may not be recognized until after the patient is in the operating room. Finally, a deep peritumoral injection performed without imaging guidance carries the added risk of injury to the lung and/or chest wall. Peritumoral injection is technically more complex than dermal injection, but offers the opportunity to identify IMLN drainage. Opinions and research differ on the clinical benefits of IMLN drainage mapping and sampling, which remain unclear and controversial. A prospective study from 2005 found that most surgeons do not perform IMLN SLN biopsies even when internal mammary drainage is demonstrated by lymphoscintigraphy [80], a decision supported by a 1999 randomized trial that did not demonstrate a survival benefit associated with routine internal mammary dissection [81]. Conflictingly, a 2012 study found that internal mammary drainage identified on preoperative imaging was associated with significantly worse disease‐free survival [82]. It is important to note that axillary and internal mammary lymphatic drainage may not overlap, so lack of evidence for metastatic disease in one nodal basin cannot predict the disease status of another basin [83]. A recent meta‐analysis of breast cancer patients found a pooled estimated rate of metastatic IMLN involvement of 15% and that patients with axillary lymph node involvement were six times more likely to have IMLN disease [84]. Ultimately, when peritumoral injections are performed, they are typically done in conjunction with some form of dermal injection. Overall, the choice of injection technique should be made in correspondence with available preoperative imaging and the breast surgeon to optimize the clinical value of this procedure. A typical approach to SLN localization utilizes both radiotracer and intraoperative blue‐dye injection. Clinical outcome investigations comparing this dual‐tracer technique to the single injection of either blue dye or radiotracer generally favor the dual‐tracer technique [85, 86]. Although imaging can be performed following radiotracer injection, it is not typically performed in a number of practice settings. A study of preoperative, post‐injection imaging did not show significant impact on the SLN identification rate or false‐negative rate [87]. Still, there are benefits to preoperative imaging following radiotracer injection, including identification of potentially confounding skin contamination or interstitial spread of tracer or nonvisualization of axillary drainage, which can be an important prognostic sign, as this is associated with an increased risk of multiple invaded lymph nodes [88]. If performed, scintigraphic imaging should include anterior and lateral transmission images using a 57Co sheet to optimize anatomic localization. SPECT–CT imaging, if available, can also be performed at time of breast lymphoscintigraphy, and can increase the sensitivity and specificity of findings (Figure 12.8). Dosimetric considerations with radiotracer‐localized SLNB are minimal. The effective dose for a 0.5 mCi SLNB injection is approximately 0.05 mSv [89]. This is less than the dose from a conventional chest radiograph (0.1 mSv). The fetal dose for a 0.5 mCi injection was calculated to be less than 0.014 mGy [90]. This is well below the NCRP maximum permissible dose equivalent of 5 mSv used for fetal exposure of pregnant workers. Lactating women should discontinue breast feeding for 24 hours following the procedure to avoid exposure to the nursing infant [91]. PET is used for multiple indications in the setting of breast cancer, including initial staging, treatment response, and surveillance for possible recurrence [92]. In addition to other fundamental guidelines of patient preparation and study performance, for the indication of breast cancer it is recommended to administer the 18F‐FDG and intravenous iodinated contrast in the upper extremity contralateral to the primary breast malignancy to prevent artifacts in the ipsilateral axilla that may obscure evaluation of regional lymphadenopathy. Figure 12.8 (a) Planar image obtained 20 minutes following right periareolar subdermal injection of 5 mCi 99mTc‐sulfur colloid in a 58‐year‐old female patient with biopsy proven right breast malignancy without obvious axillary metastases on preoperative imaging demonstrates the periareolar injection site and two additional foci approximating the expected location of right axillary lymph nodes. (b–d) Subsequent SPECT–CT images localize the periareolar injection site (b), intense activity in a right axillary level I SLN (c), and faint activity in a higher right axillary level I versus subpectoral level II lymph node (d). Detection and characterization of primary breast tumors is most commonly performed via mammography, ultrasound, and/or breast MRI. PET‐CT is not performed for the evaluation of the primary breast tumor per se; however, the primary tumor is visualized and further characterized with FDG PET‐CT when this modality is employed for whole‐body staging or assessment of treatment response.
12
A Correlative Approach to Breast Imaging
Anatomy of the Breast and Locoregional Lymph Nodes
Breast Cancer Classification
Molecular Techniques in Breast Imaging: A Correlative Approach to the BI‐RADS Paradigm
Conventional Nonmolecular Breast Imaging Modalities
Molecular Breast Imaging
Limitations of Early Scintimammography
Dedicated Molecular Breast‐imaging Systems
Single‐photon Gamma Imaging Systems
Annihilation Coincidence‐detection Systems
Breast‐imaging Reporting and Data System
Clinical Indications and Practical Uses of Molecular Breast Imaging
Supplemental Breast Cancer Screening
Assessment category
Recommendation
Likelihood of malignancy
0 – Incomplete
Needs additional imaging evaluation (recall)
and/or
prior imaging for comparison
n/a
1 – Negative
Routine screening
~0%
2 – Benign
Routine screening
~0%
3 – Probably benign
Initial short‐term (typically 6 months) imaging follow‐up
<2%
4 – Suspicious
Tissue diagnosis
>2% to <95%
5 – Highly suggestive of malignancy
Tissue diagnosis
>95%
6 – Known biopsy‐proven malignancy
Appropriate action
n/a
Diagnostic Problem Solving
Alternative to Breast MRI
Image‐guided Breast Biopsies
Molecular Breast Imaging: Key Concepts
Biopsy method
Compression
Patient positioning
Advantages
Limitations
Ultrasound (US)
No
Supine
Preferred method, real‐time verification of needle position, fast, no ionizing radiation, low cost, patient comfort, accessibility to all areas of the breast
Not useful for microcalcifications or distortions
Stereotactic
Yes
Upright/prone or lateral decubitus
Useful for microcalcifications (ex vivo sample verification possible) and mammographic distortions
Ionizing radiation, patient discomfort/risk of vasovagal response
MRI
Yes
Prone
Useful for ultrasound and mammographically occult lesions, no ionizing radiation
High cost, long procedure time, limited in patients with claustrophobia, obesity, and renal insufficiency, need for gadolinium contrast administration
MBI
(BSGI/PEM)
Yes (mild)
Upright
Useful for indeterminate or poorly visualized lesions on mammography or ultrasound, less compression than mammography or MRI techniques, ex vivo sample verification possible
Long procedure time, ionizing radiation, difficult to sample lesions close to the pectoral muscle
Breast Lymphoscintigraphy and Sentinel Lymph Node Biopsy
Role of Lymphoscintigraphy in Staging Breast Cancer
Breast Lymphosintigraphy Injection Techniques
Dermal Radiotracer Injection
Peritumoral Radiotracer Injection
Comparison of Radiotracer Injection Techniques
Dual Blue‐dye and Radiotracer SLN Localization Technique
Breast Lymphoscintigraphic Imaging (Sentinel Lymph Node Mapping)
Role of PET in Breast Cancer
Breast Lymphoscintigraphy and SLNB: Key Concepts
PET in Primary Breast Cancer
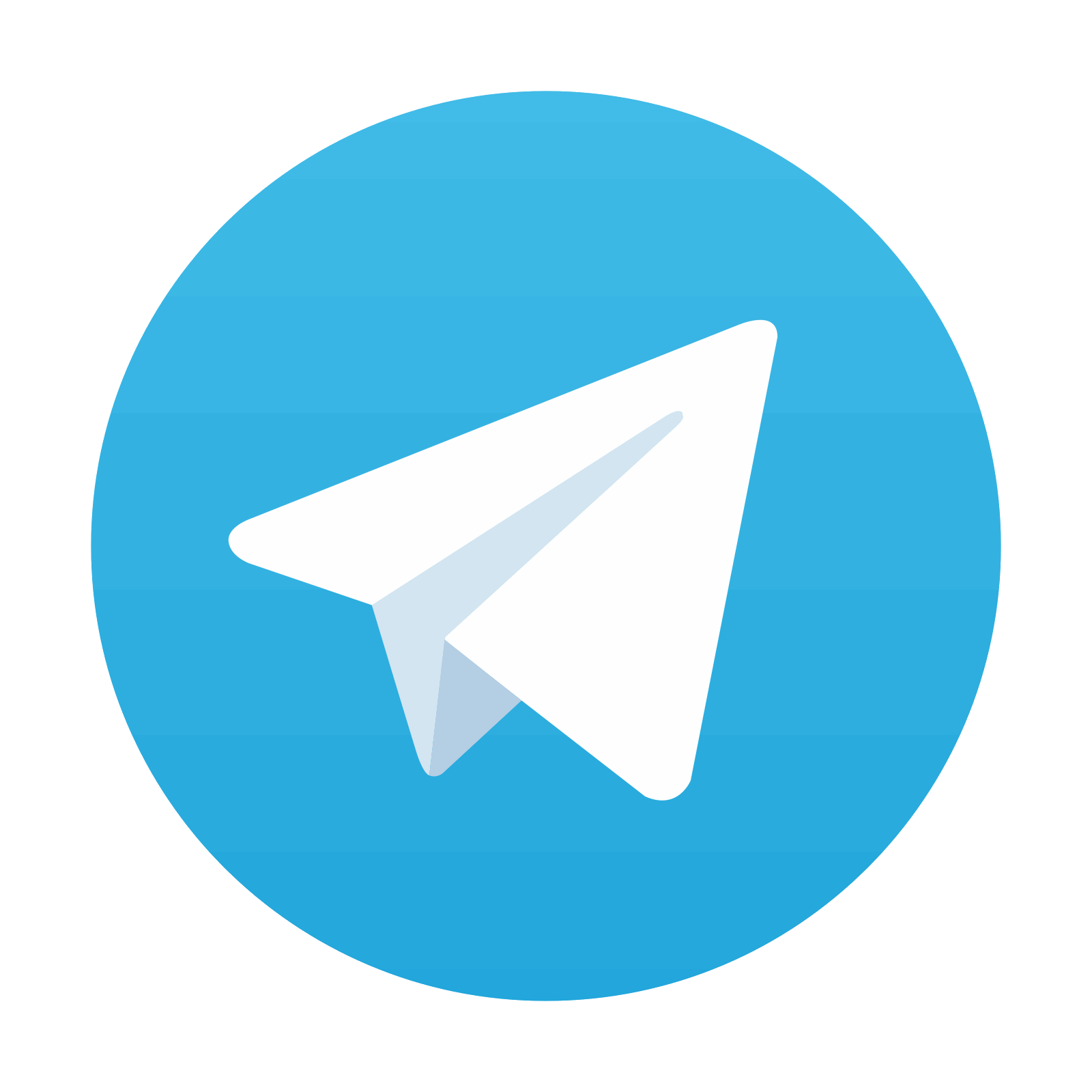
Stay updated, free articles. Join our Telegram channel

Full access? Get Clinical Tree
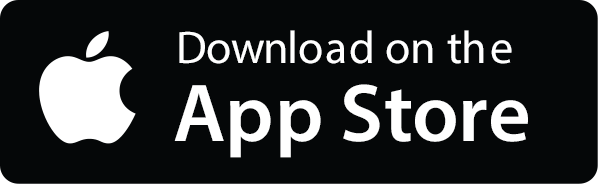
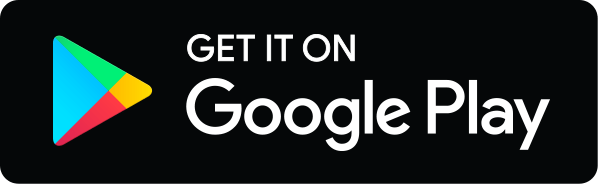