A manual, easy-to-use, self-shielded system including an organic-based 68Ge/68Ga generator is available on the market from ITG (ITG Isotope Technologies Garching GmbH, Garching, Germany). The system is equipped with a sterile single-use kit, no post-processing of 68Ga eluate is needed, and it has a built-in filter integrity test device (Fig. 2).
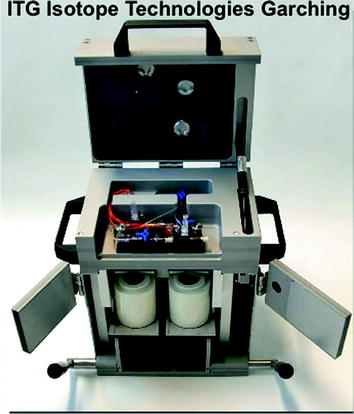
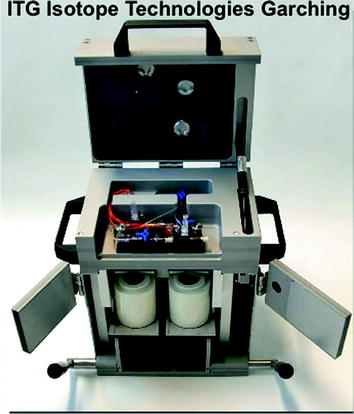
Fig. 2
Manual self-shielded system
Supply of automatic systems has considerably increased in the last few years. Several automated systems are commercially available, combining generator elution, post-processing, 68Ga labeling reactions, and purification of 68Ga-labeled peptide with pharmaceutical characteristics.
3.1 Classification and Characteristics of Automated Systems
A tentative classification of the available automated systems could be based on:
Post-processing approach: anionic, fractionation, cationic
System engineering: fully equipped compact system, modular system
Fluid path: fixed tubing system, disposable sterile cassette.
All the post-processing approaches mentioned in Sect. 2 have been adopted in developing and engineering automatic systems. The choice of post-processing approach is based on scientific considerations which fall outside the scope of this chapter.
There are, however, commercial reasons often based on collaborations and spin-offs between academia and companies which have actually led to preferential implementation of one of the post-processing approaches.
Moreover, fractionation was widely used, at least in the early years, because it is intrinsically easy to use and cost effective, and it shorten synthesis time.
Some companies still offer a choice of systems adopting different post-processing approaches.
Data from the literature evidence differences in the radiochemical yield of the whole 68Ga-DOTA-peptide labeling process among different post-processing approaches.
The 60% decay corrected (d.c.) radiochemical yield was reported using a fully automated system with a fractionation protocol (Decristoforo et al. 2007) and 30 μg DOTA-d-Phe1-Tyr3-octreotide (DOTATOC) in acetate buffer. Different amount of peptide used, strong influence of the type of buffer (Bauwens et al. 2010), and incubation parameters make comparison among automatic systems using different post-processing approaches extremely difficult.
A radiochemical yield of 95% was reported by cation exchange-based post-processing because of the high purity of the 68Ga eluted from AG50 X8 resin (Zhernosekov et al. 2007). Since this method requires manual interference in the labeling procedure, this protocol was completely automated by using a miniaturized multi-use column with a cation exchange resin (AG 50 W-X4) similar to that described by Zhernosekov and, successively, using a commercial, disposable cation exchange cartridge (Ocak et al. 2010).
Replacement of a multiple-use resin by a single-use cartridge has led to better cGMP compliance and simplification of the process, as cleaning and reconditioning of the column for multiple uses is avoided. Better microbiological safety with respect to sterility and pyrogens should also be considered. Inactive metal impurities, except Zn, are less reduced than with a permanent cation exchanger but without problems for synthesis.
Comparative decay/non-decay corrected yields of 68Ga-DOTATOC, with using different post-processing approach synthesis methods were reported by the same author (Ocak et al. 2010). These values ranged from 67% d.c. for the fractionation method to 77–84% d.c. using different cationic post-processing and different buffer. The longer overall synthesis time with fully automated prepurification as compared with a fractionated system (Decristoforo et al. 2007) was compensated by the advantage of using all available activity of 68Ga by concentration on the cation exchanger, thus balancing the radiochemical yield.
Commercial automatic systems based on fractionation, anionic, and cationic post-processing are shown in Figs. 3, 4, 5.
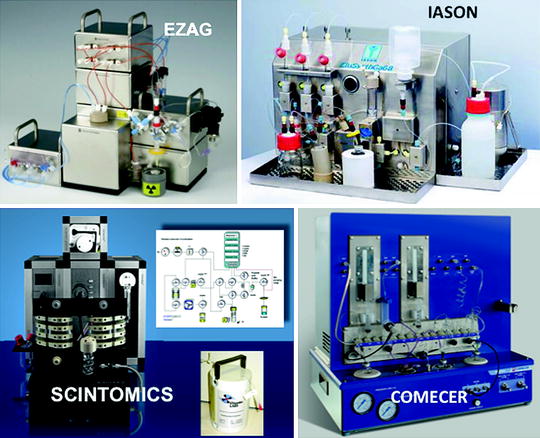
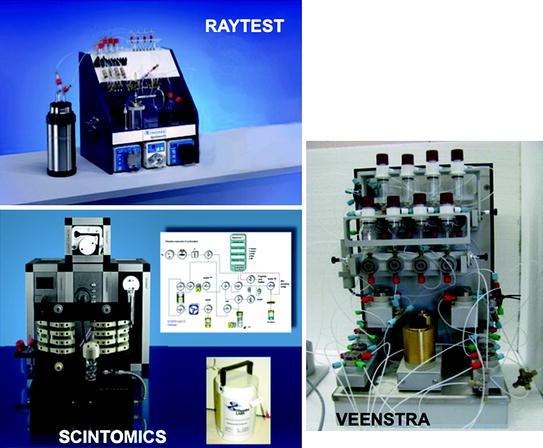
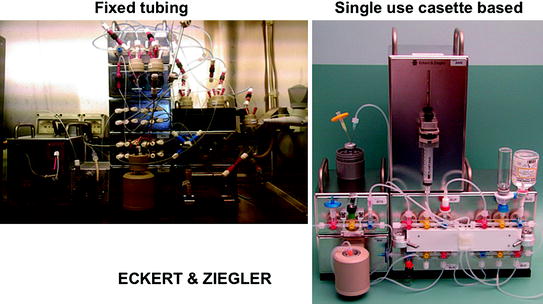
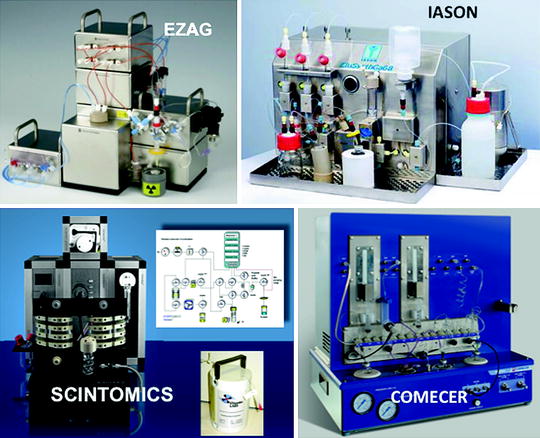
Fig. 3
Fractionation-based automatic systems
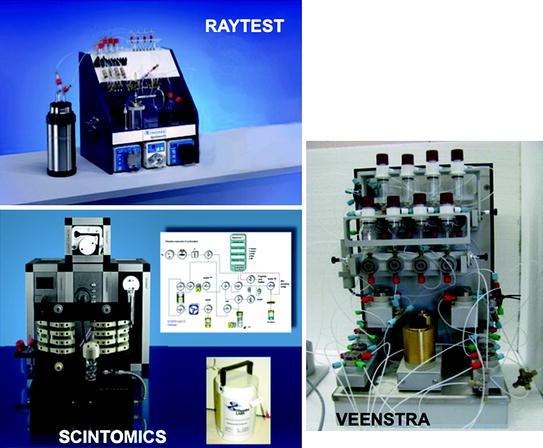
Fig. 4
Anionic exchange-based automatic systems
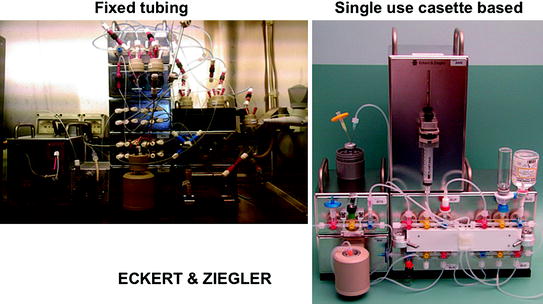
Fig. 5
Cationic exchange-based automatic systems
Fully equipped, ready-to-use, compact systems are available from companies such as Iason, Veenstra, Comecer, and Raytest (Figs. 3, 4, 5). These systems represent turnkey solutions, suitable for cGMP pharmaceutical environments, but, at least in principle, with limited flexibility.
Increasing flexibility can be achieved by “modular” systems or systems based on expandable platforms (Eckert and Ziegler, Scintomics). Automation of a broad spectrum of procedures for radiolabeling, isotope purification, routine tracer production, and other radiopharmaceutical processes is possible with such systems.
System integrated components, such as handling parts, sensors, pumps, valves, regulators, heaters, high-performance liquid chromatography (HPLC) equipment, etc., allow for a high level of flexibility in the construction of customized modules.
It is important to underline that the need for flexibility and regulatory requirements are often conflicting, since regulations pose some hurdles to the production of radiopharmaceuticals for clinical use. The different chemistry approaches used, the many types of PET tracer required for research and clinical studies, and the limited resources in production facilities call for flexible systems. On the other hand, regulations for small-scale hospital radiopharmaceutical preparation are based on risk analysis-based validation plans, extensive qualification/validation protocols, and full documentation and traceability, thus reducing the possibility of implementing flexible processes.
Since regulations (cGMP or local, national pharmacopoeia regulations) are becoming mandatory for radiopharmaceutical preparations, a current trend in engineering of automatic systems is to move from fixed tubing to cassette systems.
Single-use sterile cassettes avoid time-consuming cleaning validation procedures as well as intense cleaning and sanitation routines. By changing the cassette, multiple syntheses can be easily performed even for various tracers and with different radionuclides.
This is particularly attractive for management of neuroendocrine tumors, since radiolabeling of DOTA-somatostatin analogs with 68Ga for tumor staging and labeling of the same compound with a radionuclide such as 177Lu or 90Y for therapy become feasible. By simply changing the cassette, it is possible to avoid the delicate cleaning validation process to guarantee complete removal of 90Y or 177Lu.
68Ga/177Lu/90Y/111In-DOTA peptides have been synthesized with high purity, high radiochemical yield (>80% d.c. for 68Ga-DOTA peptides and 90% d.c. for 177Lu/90Y/111In-DOTA peptides), and acceptable synthesis time (Petrik et al. 2011). Sterile, single-use, disposable cassettes assembled under cGMP-compliant cleanroom conditions guarantee better control of sterility and apyrogenicity of the radiopharmaceutical preparation, even if complete control of these parameters relies only on a fully validated process.
Eckert and Ziegler engineered a self-shielded cassette-based solution for routine production, becoming an integral part of the synthesis system. Only single-use cassettes are located inside the shielded area, allowing weight reduction to one-tenth of that of a conventional hot cell. The system can be placed in virtually any laboratory, without any reinforcement of the floor, thus facilitating dissemination of the methodology.
Many companies are moving toward single-use disposable kits or a cassette approach. GE Healthcare has drawn up the Gallea project for development of a cassette system for 68Ga radiopharmaceutical preparations based on the Fastlab platform.
Cassette systems have also some drawbacks if compared with fixed tubing systems: first, the high cassette cost and the reliance on the cassette manufacturer; second, reduced flexibility. Optimized and fully validated cassettes for each radiopharmaceutical are needed, and the development of new cassette systems strongly relies only on marketing considerations. Third, the fluid path of the cassette is often engineered by commercially available disposable medical devices (tubes, stopcock valves, kits for intravenous injection). Leaks and bubbles are then possible, and special care should be taken to assess all components and connections to avoid malfunctioning resulting in low radiochemical yield.
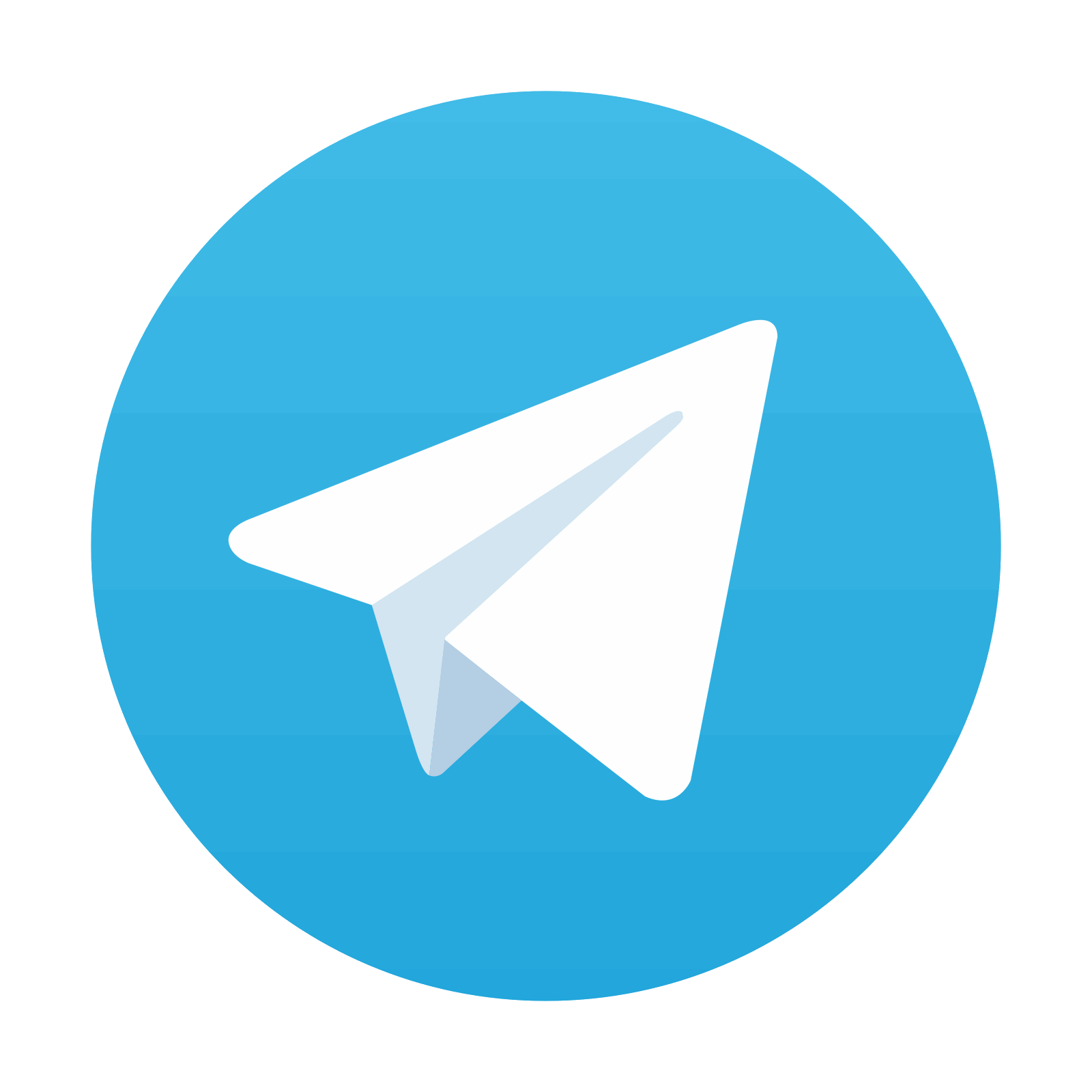
Stay updated, free articles. Join our Telegram channel

Full access? Get Clinical Tree
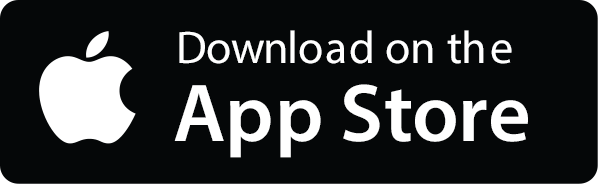
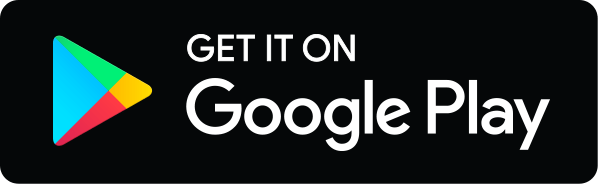