Fig. 1
A 40-year-old male was treated for a radiation-induced atypical clival meningioma. He had a history of a pediatric posterior fossa tumor, reported as a glioblastoma, and had received radiation therapy 30 years prior: 30 Gy to the whole brain and a 55 Gy boost to the posterior fossa with 6 MV photons. Pathology slides from his original tumor had been destroyed. He presented with left-sided hearing loss, dysphagia, and balance disturbance and was found to have a large left cerebellar-pontine angle/posterior clival meningioma with brainstem compression (a). Surgical debulking was undertaken via a two-stage approach including lateral suboccipital craniotomy with pathology showing a WHO grade 2 meningioma. A near-total resection of tumor was obtained (b). Unfortunately he suffered left cranial nerve VI and VII palsies with neurotrophic keratopathy eventually requiring left eye enucleation and multiple lower cranial nerve palsies with dysarthria and dysphagia requiring permanent tracheostomy and gastrostomy tube feeding. Within 6 months from surgery, his tumor had regrown and was again approximating the brainstem (c). No further surgery was advised. He was referred for proton therapy due to concern about brainstem tolerance to additional radiation considering his prior radiation and surgical trauma. A treatment plan was generated using a combination of through and patch fields (keeping the brainstem at the aperture edge) and anterior oblique fields with distal blocking of the brainstem to avoid delivery of any radiation through the brainstem. Two schemas were used of four fields each. The prescription dose was 63 Gy (RBE) in 35 fractions, allowing the surface of the brainstem to receive an additional 50 Gy (RBE) (d, e). Because of his debilitated condition, daily anesthesia was required to comply with immobilization. The first posttreatment MRI at 6 weeks showed central tumor necrosis and transient enlargement of the tumor without clinical worsening. At 12 months postradiation, his MRI showed significant regression of tumor, volumetrically reduced from 6 to 2.2 cm3 (f). At 37 months from radiation, the patient had continued radiographic regression of tumor
5 Proton Reirradiation of Chordoma
Chordomas are rare primary bone tumors with a high propensity for local recurrence even after aggressive surgery and radiotherapy. For clival chordomas, maximizing tumor debulking and optimizing residual tumor coverage by high-dose radiotherapy are associated with superior outcomes (McDonald et al. 2016a). For patients with recurrent disease after prior radiation, treatment options are limited. While effective targeted drug therapies are desperately needed in chordoma, local control measures remain the mainstay of treatment.
Salvage surgery alone rarely achieves a durable period of disease stability and has a reported 2-year overall survival of 63 % (Fagundes et al. 1995). Reirradiation options are typically constrained by the prior dose delivered to closely adjacent critical normal structures, particularly the spinal cord for extracranial chordomas and the brainstem and optic apparatus for clival chordomas. Small intracranial recurrences are often amenable to stereotactic radiosurgery (SRS) with satisfactory local control, although there is a not insignificant risk of marginal failure of about 15 % (Kano et al. 2011).
Researchers at the now-closed Indiana University Health Proton Therapy Center reported on 16 previously irradiated patients with recurrent or progressive chordoma (McDonald et al. 2013a). Half the patients underwent salvage surgery in management of their recurrent or progressive disease. At a median of 37 months after a median prior dose of 75.2 Gy, patients were retreated to an additional median dose of 75.6 Gy (RBE). At a median follow-up of 23 months, the 2-year estimate of local control was 85 % and overall survival 80 %. The 2-year estimate of late grade 3+ toxicity was 19 %. The disease control in this experience with aggressive proton reirradiation compares very favorably to other interventions in a population with a historically poor prognosis.
Figure 2 is an example of proton therapy in reirradiation of a recurrent cervical spine chordoma.
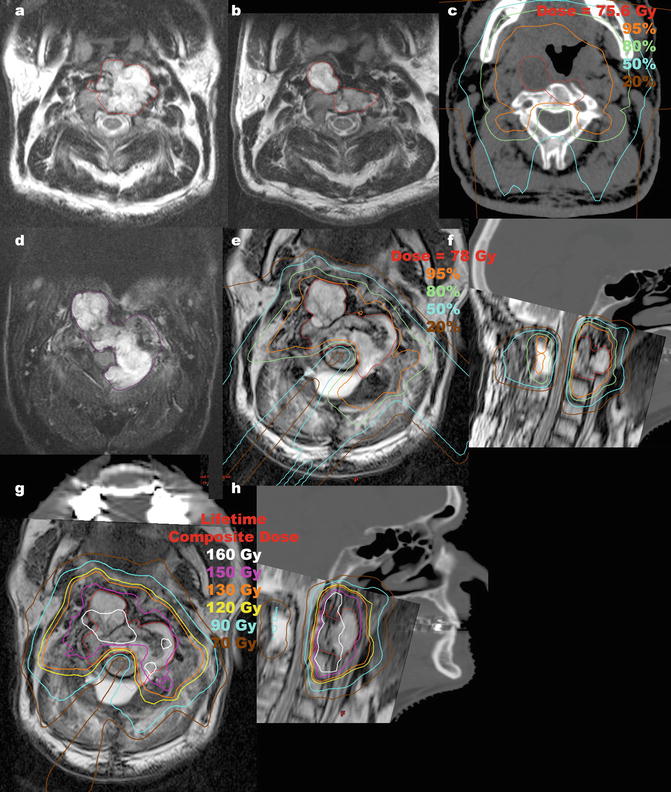
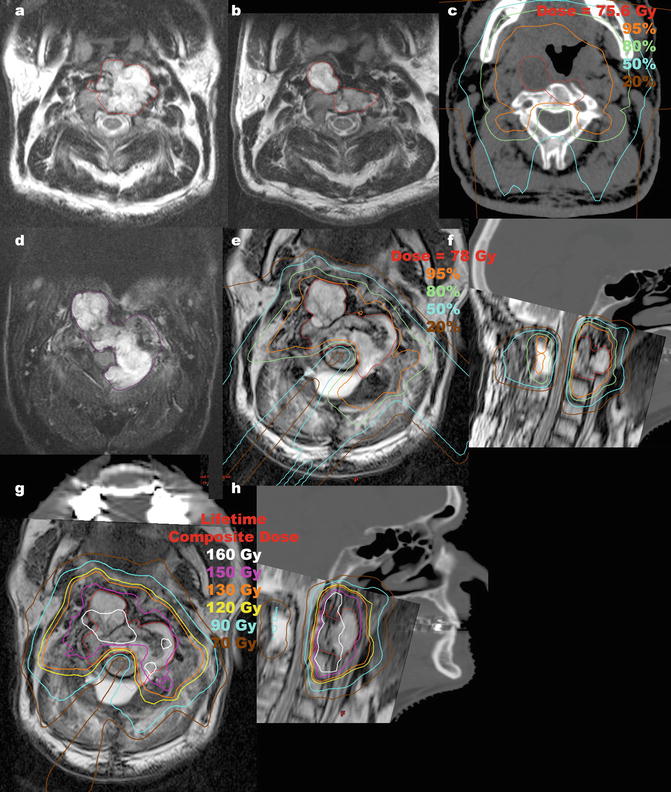
Fig. 2
A 67-year-old man was reirradiated for a cervical spine chordoma. He presented with dysphagia and imaging showed a destructive mass at C2 extending into the prevertebral space (a; tumor outlined in red). He underwent transoral partial resection with pathology showing chordoma and was observed. Imaging one year later showed a bulky recurrence (b; tumor outlined in red). After neurosurgical evaluation, the morbidity of re-resection was felt to be too great and he was referred for proton therapy. He was treated to 75.6 Gy (RBE) in 42 fractions by another physician (c, gross tumor volume outlined in red). Due to concern for potential surgical seeding, a large treatment volume was defined which covered the soft palate, resulting in permanent xerostomia and dental caries. Three years after proton therapy, the tumor remained stable in size but he developed a solitary supraclavicular nodal metastasis, which was completely excised. At 38 months from radiation, imaging showed progression of the primary tumor. A 6-month trial of imatinib was undertaken with repeat imaging showing further tumor progression now encroaching upon the cervical spinal cord (d; tumor outlined in magenta). He was referred for neurosurgical decompression and posterior spine stabilization, which achieved clearance around the spinal cord, although complete surgical resection was not possible. He was then retreated with proton therapy to 78 Gy (RBE) in 38 fractions, 4 years after his prior radiation therapy (e, f; gross tumor volume outlined in red). A CT myelogram was performed in the immobilization devices to define the cervical spinal cord. CT simulation was performed with an orthopedic metal artifact reduction algorithm in light of his spine stabilization hardware and dental amalgam artifacts. He was treated with two complex alternating schemas of through and patch fields, the first schema involving six fields and the second involving five fields. The spinal cord was blocked by all beams to keep the spinal cord surface dose at the 50 % isodose line. His lifetime dose distribution is shown (g, h). The maximum spinal cord point dose was 54.9 Gy from his initial course, 46.5 Gy from his reirradiation and cumulative lifetime maximum point dose 97.5 Gy (75.4 Gy to 0.5 cm3). He did not develop any oral mucositis during reirradiation and had only grade 1 odynophagia and grade 1 dermatitis. Three months after reirradiation, he started planned adjuvant therapy with erlotinib which was stopped after 1 month due to skin toxicity. At 6 months after reirradiation, he had a focus of posterior oropharyngeal wall soft tissue necrosis treated with hyperbaric oxygen. Unfortunately, the soft tissue necrosis progressed, leading to exposure of bone and required tracheostomy and gastrostomy tube feeding. He survived for two years after reirradiation without evidence of tumor progression or spinal cord myelopathy but died from a sudden carotid artery rupture, highlighting the significant risks of high dose retreatment
6 Proton Reirradiation of Gliomas
Recurrent or progressive infiltrative glioma develops in almost all patients after initial therapy. In the absence of high-quality data on optimal management, patients with recurrent glioma are typically evaluated for further resection, chemotherapy, reirradiation, and other interventions based on tumor histology, genetic factors, size, location, and patient performance status, among other factors (Stupp et al. 2014). For glioblastoma, the most common malignant primary brain tumor in adults, the standard of care for initial management is maximal safe surgical resection followed by radiotherapy with concurrent and adjuvant temozolomide (Stupp et al. 2009). The median progression-free survival is approximately 7 months. The Radiation Therapy Oncology Group is currently enrolling patients in a randomized phase II trial for patients with recurrent or progressive glioblastoma in which patients are randomized to bevacizumab alone or bevacizumab plus hypofractionated reirradiation to 35 Gy in 10 fractions. This trial should provide valuable prospective evidence to evaluate the potential benefit of early incorporation of reirradiation.
One of the most significant clinical concerns with reirradiation of gliomas is the risk of brain radiation necrosis. Proton therapy is theoretically appealing because highly conformal reirradiation can be delivered with lower dose to adjacent nontarget brain tissue. However, this would not reduce the risk of central radiation necrosis occurring within the reirradiation target. Furthermore, modern photon techniques of hypofractionated reirradiation for high-grade gliomas have been associated with no discernable or very low risk of radiation necrosis (Fogh et al. 2010). This is presumably due at least in part to the limited survival time of patients. For these reasons, the routine application of proton therapy in reirradiation of high-grade gliomas may not translate into measurable clinical improvements in toxicity.
If prognostic tools improve to accurately identify better prognosis patients (whose longer survival time would presumably place them at greater risk of radiation necrosis and neurocognitive effects of reirradiation), proton reirradiation may be of benefit in these select patients. Proton therapy may be a useful tool in prospective dose-escalation trials of reirradiation. A similar strategy is being employed in an open phase I/II trial at the University of Heidelberg evaluating the role of carbon ion therapy in recurrent gliomas (grades 2–4). The phase I component is designed to establish a recommended carbon ion dose via dose escalation from 30 to 48 Gy equivalent (GyE) in 3 GyE fractions, while the phase II component will compare 12-month survival against photon reirradiation to 36 Gy in 2 Gy fractions (Combs et al. 2010).
Researchers at the now-closed Indiana University Health Proton Therapy Center reported on 20 patients with recurrent gliomas who were treated with proton reirradiation (Galle et al. 2015). Three had grade I or II gliomas, 4 grade III, and 13 grade IV. The patient population was heterogeneous in terms of prior therapy and utilization of concurrent chemotherapy. Additionally, the dose of reirradiation varied from hypofractionated regimens to full dose reirradiation in conventional fractionation. Protracted fractionation was generally used in patients with a long time interval from prior radiation therapy (up to 12 years) based on a belief that such patients may be longer-term survivors. The median dose of reirradiation was 59.4 Gy (RBE) (range 37.5–60) for grade III tumors and 54 Gy (RBE) (range 30–60) for grade IV tumors. The median survival after reirradiation was 10.2 months for grade III tumors and 8.2 months for grade IV tumors. With reference to prior radiation dosimetry, efforts were made to direct proton reirradiation beams to minimize the volume of reirradiated brain. There was a 10 % crude incidence of radiation necrosis. It is difficult to derive any conclusions from such heterogeneous data, but it is quite uncommon to deliver full course reirradiation, and the apparent reasonably low risk of radiation necrosis is provocative even if the benefit of full dose reirradiation is unknown.
Figure 3 illustrates an example of proton therapy in reirradiation of a patient with a recurrent WHO grade III anaplastic astrocytoma.
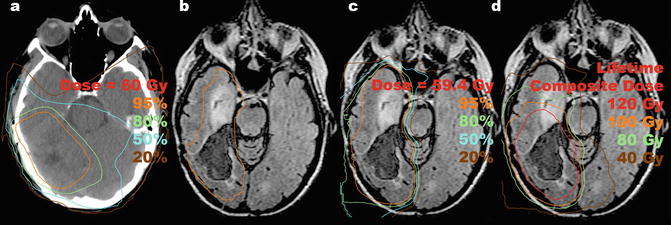
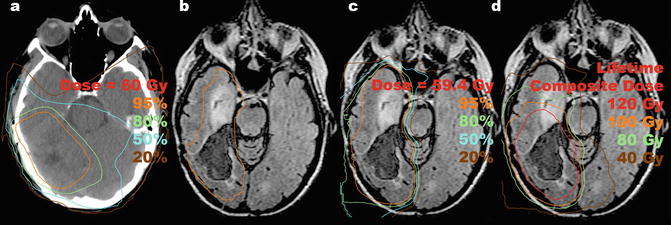
Fig. 3
A 48-year-old woman was reirradiated for a recurrent glioma. She had a history of a complete resection of right posterior temporal anaplastic astrocytoma (grade III) and received adjuvant radiation therapy to 60 Gy in 30 fractions (a) without chemotherapy. Seven years later she developed episodes of confusion prompting MRI scan that revealed local recurrence of non-enhancing tumor with progression of FLAIR abnormality into the anterior ipsilateral temporal lobe highly suspicious for tumor. She underwent radical subtotal resection guided by functional MRI, confirming recurrent grade III astrocytoma, IDH-1 intact, with residual inoperable tumor. She was offered reirradiation with proton therapy to 59.4 Gy (RBE) in 33 fractions with concurrent temozolomide. The residual tumor and the target volume for reirradiation are shown in orange (b). Proton therapy was used to avoid radiation to the contralateral hemisphere and to minimize dose to the brainstem (c). The previous tumor region received a cumulative dose of 120 Gy between the two courses separated by 7 years (d). She developed bone marrow suppression requiring dose de-escalation of temozolomide in the later course of therapy. Within 1 year, the patient developed brain parenchymal radiation necrosis that was treated with bevacizumab and hyperbaric oxygen treatment. She developed a sustained contralateral hemiparesis with dysarthria. Eighteen months after reirradiation, the patient was alive with no radiographic evidence of tumor progression
7 Proton Salvage Craniospinal Irradiation
Salvage craniospinal irradiation has been reported, primarily in children, in treatment of recurrent and disseminated ependymoma (Merchant et al. 2008), recurrent medulloblastoma after prior CSI (Massimino et al. 2009), and for other histologies with neuroaxis dissemination after prior focal radiation (Wei et al. 2012). Researchers at St. Jude Children’s Research Hospital reported on varied techniques of salvage reirradiation for recurrent ependymoma. For those treated with salvage CSI, standard photon technique with opposed lateral brain fields was used, with custom blocking designed to limit the brainstem and spinal cord to a maximum cumulative radiation dose of 55.8 Gy. While effective at shielding critical structures and limiting cumulative radiation dose, lateral blocks also shield a volume of cerebrospinal fluid and leptomeningeal space potentially harboring microscopic disease. This could theoretically reduce the effectiveness of salvage CSI and allow for reseeding. Others have used IMRT to attenuate dose to previously irradiated critical structures while maintaining coverage of the surrounding target volume (Wei et al. 2012), but this cannot achieve complete sparing in the same way as lateral blocks.
Using the finite distal range of proton therapy, researchers at the now-closed Indiana University Health Proton Therapy Center reported a novel technique to block a critical structure on lateral fields and then fill in or “plug” dose to cover the target volume lateral to the structure. The resulting plan created a “donut” hole of complete dose avoidance surrounding the critical structure. Two example cases were highlighted in which critical structures were felt to require complete sparing from additional radiation dose: one child receiving salvage CSI for recurrent and disseminated medulloblastoma in which the optic chiasm was spared, and one adult with recurrent and disseminated anaplastic meningioma in which the previously irradiated portion of the lateral brainstem was spared. Compared to lateral photon fields with blocks and with IMRT, this proton technique improved coverage of the planning target volume while reducing the mean and maximum dose to the critical organs at risk (McDonald et al. 2013b).
Similarly, a case report from researchers at the University of Pennsylvania reported on the use of pencil beam scanning proton therapy to deliver salvage craniospinal irradiation with brainstem sparing (Hill-Kayser and Kirk 2015). The case involved a child with a posterior fossa ependymoma whose prior radiation delivered a maximum brainstem dose of 60 Gy (RBE). Ten months later, spinal dissemination was detected and salvage CSI to 36 Gy (RBE) followed by focal tumor boosts was offered, using pencil beam scanning to limit the surface of the brainstem to an additional 5 Gy (RBE).
In addition to the ability to create regions of complete dose avoidance if necessary around previously irradiated critical structures, proton therapy offers the advantage of no exit dose to viscera anterior to the spine during CSI, which is expected to reduce both acute and late toxicity by complete radiation avoidance. Retrospective cohort analysis supports reduced acute gastrointestinal and hematologic toxicities with proton CSI compared to photon CSI in adult patients treated for medulloblastoma (Brown et al. 2013). Additionally, retrospective cohort analysis found that, compared to photon CSI, proton CSI was associated with fewer late endocrine abnormalities in children treated for standard risk medulloblastoma (Eaton et al. 2015). Radiobiologic modeling predicts that proton CSI is associated with a reduced risk of secondary malignancies compared to photon techniques (Zhang et al. 2013). These data support the role of proton therapy in craniospinal irradiation for patients of all ages.
8 Proton Reirradiation of Ocular Melanomas
Proton therapy is an established modality of treatment for ocular melanomas with a very high rate of local control and favorable toxicity profile (Dendale et al. 2006; Desjardins et al. 2012). In addition to close collaboration with a specialized ophthalmologist, the treatment requires a dedicated patient setup, planning software, and expertise which may not be available at every proton treatment center. However, the shallow beam range and therefore low proton energy required for treatment means that proton therapy for ocular melanomas is also available at a number of centers with low-energy cyclotrons unsuitable for treatment of broader indications. A systematic review and meta-analysis suggested charged particle therapy for uveal melanoma was associated with lower rates of local recurrence, retinopathy, and cataract formation than plaque brachytherapy (Wang et al. 2013).
Choroidal melanomas arising in proximity to the optic disk (juxtapapillary) may be inappropriate for plaque brachytherapy due to inability to properly position the plaque for adequate tumor coverage. Stereotactic radiosurgery (SRS), hypofractionated stereotactic radiation therapy (SRT), and proton beam therapy have been used for posterior choroidal melanomas with success. A comparative treatment planning study of SRT and proton beam therapy for choroidal melanomas arising near the optic disk or fovea centralis found superior dosimetry with proton therapy in the majority of cases (Hocht et al. 2005). Clinical data from the Clatterbridge Cancer Centre and Sheffield Ocular Oncology Service compared outcomes for patients treated with SRS compared to proton therapy for choroidal melanomas (Sikuade et al. 2015). SRS and proton therapy were selected for patients with tumors considered either too large for plaque brachytherapy or for those located too close (<2.5 mm) to the optic disk for plaque placement. While tumor control was very high with both treatments, their analysis found a statistically significant lower rate of severe vision loss with proton therapy compared to SRS for patients whose tumors touched the optic nerve and for those >3 mm from the fovea. It may be that the fractionation used for proton therapy in this series (53.1 Gy (RBE) in 4 fractions) conferred fewer late effects compared to SRS (35 Gy at the 50 % isodose line in 1 fraction) or that other confounding factors were related to the difference in visual preservation.
Researchers at the Massachusetts General Hospital (MGH) reported on 31 patients with recurrent uveal melanoma who received a second course of proton therapy (Marucci et al. 2006). Nearly all the patients had received 70 Gy (RBE) in 5 fractions for both the initial course and the salvage course of proton therapy. At a mean follow-up of 50 months, the 5-year estimate of local control after salvage proton therapy was 69 %. The 5-year eye retention rate was 55 %, with 27 % of those who retained their eye having useful vision of 20/200 or better. Of the nine patients undergoing enucleation, five were due to local recurrence and four due to intractable pain.
Researchers at the Helmholtz-Zentrum Berlin reported on 48 patients with recurrent uveal melanoma after a variety of prior treatments (54 % previously irradiated) who received salvage proton beam radiation, with most receiving 60 Gy (RBE) in 4 fractions. At a mean follow-up time of 81 months, the 10-year estimate of local tumor control after proton reirradiation was 92.1 %. One patient required enucleation for local recurrence. At 5 years after salvage proton therapy, 24 % had useful vision of 20/200 or better. Compared to the MGH experience, the improved tumor control and lower rate of enucleation may be related to fewer patients having had prior radiation treatment or differences in other confounding variables such as tumor size. Together these data suggest that salvage proton reirradiation yields eye preservation in the majority of patients and preservation of useful vision in about a quarter of patients.
While overall survival is not compromised by local therapy with plaque brachytherapy compared to enucleation for choroidal melanomas (Diener-West et al. 2001), it is unclear whether further local therapy provides comparable survival to enucleation for recurrent disease. The MGH group compared survival outcomes for their 31 patients receiving salvage proton therapy to a cohort of 42 patients undergoing enucleation. Patients selected for enucleation had, on average, larger tumors than those selected for reirradiation. The 5-year survival estimate for those treated with reirradiation was 63 % compared to 36 % for those enucleated (p = 0.040) suggesting that survival is not compromised by salvage proton therapy compared to enucleation (Marucci et al. 2011).
9 Proton Reirradiation of Head and Neck Cancers
Despite aggressive therapy, locoregional disease failure remains common in many head and neck cancers. Reirradiation is a potentially curative treatment option for appropriately selected patients, although only a small percentage of patients achieve long-term survival (McDonald et al. 2011). The toxicities of head and neck reirradiation can be significant. A prospective multi-institutional trial using an accelerated hyperfractionated reirradiation regimen interdigitated with chemotherapy reported early grade 3 or higher toxicities in 77 % of patients. While many were hematologic, radiation mucositis occurred in 16 % and gastrointestinal toxicity in 48 %. Grade 3 or higher late radiation toxicities were reported in 37 %. In total, treatment-related deaths occurred in 8 % (Langer et al. 2007). These results drive the desire to improve the therapeutic ratio of reirradiation. Proton therapy may be advantageous in reducing the volume of previously irradiated tissues receiving additional radiation dose, potentially reducing toxicities of retreatment. Proton therapy may also enable the option of retreatment for patients whose prior radiation dose distribution is felt to preclude the safe delivery of additional radiation using other modalities.
Researchers from the now-closed Indiana University Health Proton Therapy Center reported on 61 adult patients with recurrent, progressive, or second primary head and neck malignancies after prior radiotherapy (McDonald et al. 2016b). The most frequent histologies were squamous cell (54.2 %), adenoid cystic (11.0 %), and undifferentiated (8.2 %) carcinoma. The great majority of cases (90.2 %) involved skull base tumor sites, and 45 % had macroscopic intracranial perineural spread or direct intracranial tumor extension. These patients had been referred from over 30 separate institutions and practices, most often because there were felt to be no appropriate photon-based reirradiation treatment options. The patients were heavily pretreated; 18 % had received two to four prior courses of radiotherapy, 52.5 % had undergone two or more prior surgeries, and 59 % had received prior chemotherapy.
Patients were treated to a median dose of 66 Gy (RBE) for microscopic residual disease and 70 Gy (RBE) for gross residual disease. Concurrent chemotherapy was used in a minority of patients (29.5 %). With a median follow-up time of 15.2 months (28.7 months in those alive), the 2-year estimate of overall survival was 32.7 % and median survival 16.8 months. In a competing risk analysis with death as a competing risk, the 2-year cumulative incidence estimate for local failure was 19.7 % and distant metastasis 38.3 %. Acute toxicity of maximum grade 2 occurred in 47.5 %, grade 3 in 13.1 %, and grade 5 in 1.6 %. Late toxicity of maximum grade 2 occurred in 22.6 %, grade 3 in 15.1 %, grade 4 in 5.7 %, and grade 5 in 3.8 %. There were a total of three treatment-related deaths.
Given the heterogeneity and complexity of this patient population, it is difficult to assess the relative merits of proton reirradiation. Outcomes appear comparable to series of patients treated with photon-based reirradiation, despite a patient population with more adverse risk factors and largely felt ineligible for additional photon therapy. For many of these patients, proton therapy was used to extend a reirradiation option to those who would otherwise likely have received supportive care alone or palliative chemotherapy in a minority. Compared to historical expectations of survival outcomes with supportive care and palliative chemotherapy, the patient survival outcomes appear favorable.
Investigators at the Northwestern Medicine Chicago Proton Center and the ProCure Proton Therapy Center in Somerset, New Jersey, reported a pooled analysis of 92 patients who received proton therapy as reirradiation for recurrent or metachronous head and neck cancers (Romesser et al. 2016). The most frequent histologies were squamous cell carcinoma (56.5 %), adenocarcinoma (9.8 %), and sarcomas (5.4 %). The most common tumor site was the oropharynx (85.5 %), followed by the nasal cavity and paranasal sinuses (13 %), with 8.7 % being skull base tumors. Patients were heavily pretreated: 17.4 % had two or more prior course of radiotherapy and 48.9 % had prior chemotherapy.
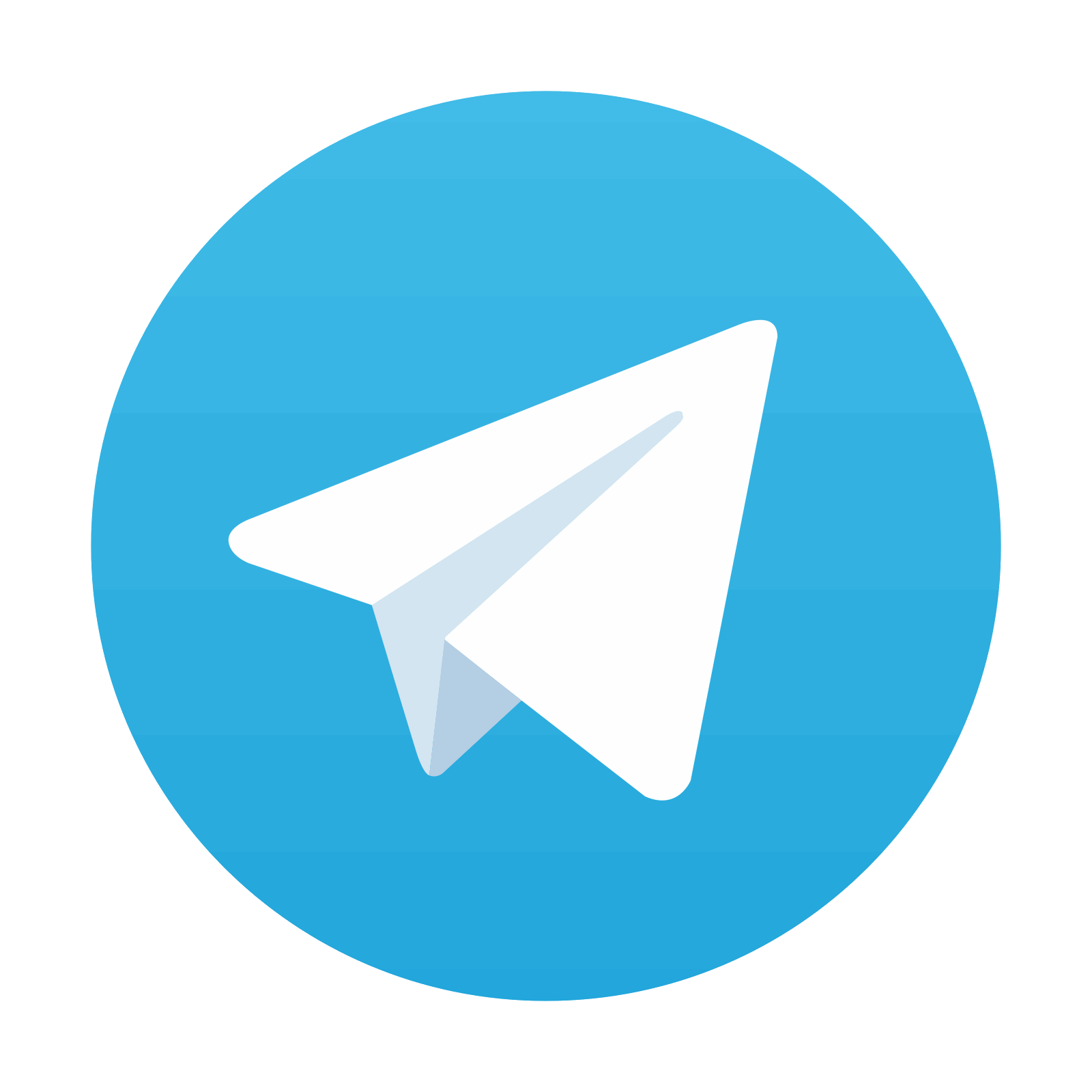
Stay updated, free articles. Join our Telegram channel

Full access? Get Clinical Tree
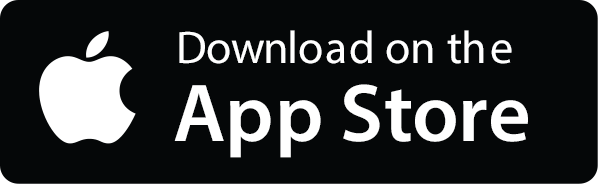
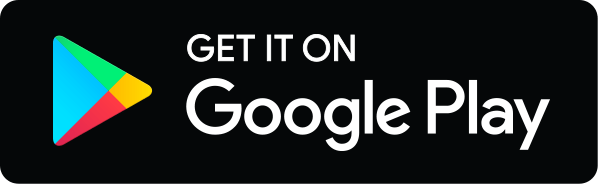