As their above studies included all symptomatic late complications (except xerostomia), the complication rates of individual OAR were actually much lower than 20 %.
This formula of estimation is very similar to the suggestion by van der Kogel (1993) that the maximum tolerable total dose in two courses amounted to 130 % of the maximum tolerable dose in a single course. In addition, he suggested the 100 % tolerable dose should be higher than the usual maximum dose constraint in the primary course since a higher risk should be accepted for re-irradiation (e.g., 60 Gy instead of 50 Gy for spinal cord):
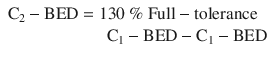
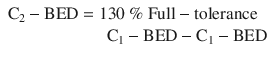
Many have since studied the late complication rates from re-irradiation, focusing on the central nervous system (CNS) structures. Nieder et al. (2005 and 2006) analyzed the data from a total of 78 patients receiving Σ − BED ranging from 102 to 205 Gy2 (α/β = 2 Gy) to their spinal cords and developed a score system which divided patients into three risk groups according to the Σ − BED, time interval before re-treatments, and the BED of any one course. The risk of myelopathy increased from 3 % to 25 % to 90 % from the low-risk to the high-risk groups. They suggested that the risk of radiation-induced myelopathy was small if Σ − BED ≤ 135 Gy2 (α/β = 2 Gy) for cervical/thoracic cord as long as BED of each course was ≤ 98 Gy2 and the interval between the courses was at least 6 months. The formula recommended for the second course BED was:


Mayer and Sminia (2008) studied the incidence of clinically symptomatic necrosis from the re-irradiation of gliomas. In contrast to the spinal cord, they found a large volume effect for normal brain tissues, but no correlation between the incidence of radionecrosis and the time interval before re-treatment (the minimum interval in their series was 3 months). Radiation-induced brain necrosis occurred only when the cumulative equivalent dose in 2 Gy fractions (EQD2) was > 100 Gy.
The study by Sulman et al. (2009) on severe toxicity (those leading to hospitalization, corrective surgery, or death) from re-irradiation by IMRT for other head and neck cancers suggested that there was 50 % recovery for CNS structures 12 months after the primary course; their recommended formula for planning re-irradiation was:


Jones and Grant (2014) derived a more detailed formula for estimating the tolerable C2-BED for CNS structures as a function of the remaining dose tolerance and the treatment gap:
where, taking a more cautious approach,
and t = treatment gap in years. The tolerable C2 dose can be calculated from the C2-BED taking into account the fractionation scheme and the corresponding α/β ratio.
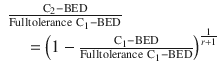
![$$ r=1.5+{e}^{\left[1.2\left(t-1\right)\right]} $$](https://radiologykey.com/wp-content/uploads/2017/11/A174_2016_48_Chapter_IEq1.gif)
These observations concurred with findings from animal experiments regarding late damage on CNS by re-irradiation and the estimated tolerance (Ruifrok et al. 1992; Ang et al. 1993; Mason et al. 1993; Wong et al. 1993). Partial recovery ranging from 20 to 55 % has been reported. The amount of recovery depends on the initial dose, the interval between the two courses, their fractionation schedules, the age and the species of animals, as well as the type and the site of the normal tissue damaged.
The above provides a rough guidance for clinicians to estimate the dose permitted at re-irradiation. Table 1 shows the maximum tolerable re-irradiation dose according to the different models assuming that the 100 % tolerance dose for an OAR by a single course is 60 Gy, the dose received at the primary course was 50 Gy, and the numbers of fractions are 35 and 30 for the first and second courses respectively. The maximum tolerable dose varied from 32 to 48 Gy (assuming that the treatment gap between the two courses is 2 years). An even higher dose can be applied if the maximum dose points of the two courses do not overlap. However, one must be cautious that the models were based on rather limited clinical data; detailed dose distributions within the OARs are often not known in these studies. Accurate prediction is difficult given the complex interplay of multiple factors and the wide range of individual susceptibility to radiation injury. Furthermore, if protection of normal tissues will compromise the adequacy of tumor coverage, patients should be duly informed of the risk and the grave consequence of salvage failure, the target dose should be given top priority if patients accept the extra risk.
Table 1
Estimation of maximum tolerable re-irradiation dose by different models basing on the assumption that the 100 % tolerance dose for an OAR by a single course is 60 Gy, the dose received at the primary course is 50 Gy, and the numbers of fractions are 35 and 30 for the first and second courses respectively
Lee et al. (2000) | Sulman et al. (2009) | Jones and Grant (2014) | ||
---|---|---|---|---|
Full-tolerance C1 dose | 60 Gy | N/A | 60 Gy | 60 Gy |
Full-tolerance C1-BED | 94.3 Gy3 | N/A | 94.3 Gy3 | 111.4 Gy2 |
Formula for C2-BED | 129 % Full-tolerance C1-BED – C1-BED | 135 Gy2 – C1-BED | Full-tolerance C1-BED – 50 % C1-BED | ![]() |
Ref tolerance BED | 121.6 Gy3 | 135 Gy2 | 94.3 Gy3 | 111.4 Gy2 |
C1 dose | 50 Gy | 50 Gy | 50 Gy | 50 Gy |
C1-BED | 73.8 Gy3 | 85.7 Gy2 | 73.8 Gy3 | 85.7 Gy2 |
Tolerable C2-BED | 47.8 Gy3 | 49.3 Gy2 | 57.4 Gy3 | 86.6 Gy2 |
Tolerable C2 dose | 34 Gy | 32 Gy | 39 Gy | 48 Gy |
Besides the total dose, the dose per fraction is another important factor affecting the risk of late toxicities. Large fractional doses incur higher risk; hyperfractionation schedule (Karam et al. 2015) is worth considering; the schedule currently used in our center is 1.2Gy per fraction, twice daily, at least 6 h apart to a total dose of 64.8 Gy.
5.3 Technical Factors
Treatment techniques for recurrent disease reflect the technological development of radiotherapy delivery in the past few decades. Initial studies mostly employed 2D external RT (Wang 1987; Lee et al. 1997; Teo et al. 1998) and/or brachytherapy (Leung et al. 2000a). Brachytherapy as sole treatment was mainly confined to superficial mucosal recurrence; salvage rate of 60 % and above had been reported for rT1 or limited rT2 disease using interstitial implants with either radioactive gold grains (Kwong et al. 2001) or iridium mold (Law et al. 2002). However, severe late soft tissue and bony complications were not uncommon. More recently, image-guided brachytherapy approach has been studied, but preliminary results by Shen et al. (2015) only achieved a median survival time of 18 months for a cohort of 30 patients treated with CT-guided permanent implantation of iodine-125 seeds.
Conformal radiotherapy gradually replaced 2D RT in the 1990s as dosimetry allows not only better tumor coverage but also better sparing of OARs (Zheng et al. 2005; Li et al. 2006; Luo et al. 2010). Available reports showed encouraging local control, but no significant reduction in late toxicities. The study by Zheng et al. (2005) using 3D conformal technique to a median dose of 68 Gy reported a very encouraging 5-year local salvage rate of 71 %. However, the 5-year OS only improved to 40 % because of serious late toxicities (all patients developed one or more late ≥ grade 3 toxicities and treatment mortality was 13 %).
In the modern era, either IMRT or stereotactic technique is used. Stereotactic radiosurgery/radiotherapy is advantageous due to the rapid dose falloff and geometric precision. Encouraging results of stereotactic treatment have been reported with local salvage rates ranging from 53 to 86 % (Chua et al. 1999 and 2009; Chen et al. 2001; Leung et al. 2009; Ozyigit et al. 2011; Dizman et al. 2014). However, very high radiation dose given per fraction could induce severe damage of normal tissues leading to torrential hemorrhage with fatal outcome (Chua et al. 1999; Ozyigit et al. 2011). Stereotactic radiosurgery should be avoided in patients with tumor encasing the carotid artery.
Currently, IMRT is the most commonly used method. Most series aimed to deliver radiation dose ≥60 Gy to recurrent gross tumor volume (GTV). Encouraging local control rate ranging from 52 to 86 % have been reported (Chua et al. 2005a; Han et al. 2012; Hua et al. 2012; Qiu et al. 2012 and 2014; Chen et al. 2013; Tian et al. 2014). However, late complications and treatment-related death varied significantly among different series. Important issues such as optimal total dose, fractionation schedule, and dose constraint for OARs remain to be defined.
Development of intensity-modulated proton therapy (IMPT) lead to further improvement in physical dose distribution: its unique beam properties (the Bragg peak, rapid distal falloff, and potentially sharper penumbra) could facilitate better sparing of OARs (Widesott et al. 2008). A recent study by Lin et al. (1999) using IMPT to doses of 59.4–70.2 cobalt gray equivalent in a cohort of 16 recurrent NPC patients (12 of whom had rT4 disease) reported 50 % OS and locoregional PFS. More importantly, the doses to critical OARs were low (0–22 Gy), and no CNS side effects were observed with a mean follow-up of 24 months.
5.4 Integration with Systemic Treatment
Despite the lack of high-level evidence, induction and concurrent chemotherapy is often employed with re-irradiation. In particular, induction chemotherapy could reduce the recurrent tumor bulk, potentially leading to better sparing of adjacent OARs especially for rT3–4 disease, and eradicate micrometastasis. Extrapolating from the experience of primary treatment, concurrent chemotherapy may be more potent for improving tumor control; the main concern is whether this would further aggravate the risk of late toxicities.
Various chemotherapy combinations have been studied including cisplatin (Poon et al. 2004; Koutcher et al. 2010), 5-fluorouracil (Poon et al. 2004; Ngan et al. 2015), gemcitabine (Chua et al. 2005), and taxane (Ngan et al. 2015). Other novel agents such as anti-EGFR agents (Lartigau et al. 2013; Ngan et al. 2015; Vargo et al. 2015) and anti-angiogenic agents (Seiwert et al. 2008) have been tested in recurrent head and neck cancer. However, data on recurrent NPC are relatively sparse (Xu et al. 2016), and the risk of bleeding should be duly considered for drugs targeting on angiogenesis (Hui et al. 2011).
6 Prognostic Factors
The most important prognostic factor is the stage of disease and GTV at the time of recurrence (Han et al. 2012; Chen et al. 2013; Tian et al. 2015; Xiao et al. 2015). Advanced recurrent T stage, especially extensive intracranial extension, is associated with worse prognosis (Leung et al. 2000b; Chua et al. 2005a; Han et al. 2012; Qiu et al. 2012). Larger recurrent tumors had poorer outcome not only because of proximity to critical structures limiting the RT dose but also of increasing risk of radioresistance due to hypoxia. The study by Xiao et al. (2015) on 291 patients with locally recurrent NPC showed that the 5-year OS rates were 63.1 % and 20.8 % for patients with tumor volume <22 cm3 and ≥22 cm3, respectively. Those with tumor volume ≥22 cm3 also had higher incidence of distant metastasis and radiation-induced toxicities.
The latency is another prognostic factor (Lee et al. 1999; Qiu et al. 2012). The study by Lee et al. (1999) on 847 recurrent NPC showed that those with long latency had better prognosis due to lower risk of distant failure: the 5-year distant failure-free rates for recurrence ≤2 years, 2–5 years, and ≥5 years were 57 %, 67 %, and 83 %, respectively. Histological type has also been shown to be an independent prognostic factor: Hwang et al. (1998) reported that patients with undifferentiated carcinoma had significantly better 5-year locoregional progression-free rate and survival than those with keratinizing type.
7 Treatment Outcomes
Table 2 summarizes the results achieved by re-irradiation using IMRT. These studies can be divided into two groups based on the dose employed in the second course of irradiation.
Table 2
Efficacy and late toxicities of re-irradiation by intensity-modulated radiotherapy
Author | Pt. no. | rT1–2 (%) | Dose (Gy) | Chemo. | Yr. | L-FFR (%) | OS (%) | Brain necrosis (%) | Massive bleeding (%) | RT-related death (%) |
---|---|---|---|---|---|---|---|---|---|---|
Chua (2005a) | 31 | 25 | 50–60 | 68 % | 1 | 56 | 63 | 7 | NR | NR |
Karam (2015) | 27 | 78 | 40–60 (1.1–1.4 Gy/fr BID) | 85 % | 3 | 53 | 57 | 0 | 0 | 0 |
Koutcher (2010) | 29 | 45 | 45–59 | 93 % | 5 | 52 | 60 | 22 | NR | NR |
Ngan (2015) | 32 | 0 | 60 | 100 % | 2 | 75 | 68 | 35 | 13 | 13 |
Qiu (2012) | 70 | 53 | Median 70 | 44 % (I) ± 18 % (C) | 3 | 49 | 52 | NR | 9 | 9 |
Han (2012) | 239 | 25 | 61.7–78.7 | 49 % | 5 | 86 | 45 | 28 | NR | 35 |
Chen (2013) | 54 | 20 | 49.8–76.6 | 52 % | 2 | 64 | 44 | 19 | 11 | 24 |
Tian (2014) | 117 | 21 | 65.4–73.1 | 0 % | 5 | 64–71 | 37 | 21 | 25 | 32 |
Studies from North America (Koutcher et al. 2010; Karam et al. 2015) and Hong Kong (Chua et al. 2005b; Ngan et al. 2015), using re-irradiation dose of around 60 Gy combined with systemic chemotherapy, achieved OS of around 60 %. Major late complication rates were variable. A recent phase 2 study by the Hong Kong NPC Study Group explored the possibility of improving outcome for rT3-4 tumor by integrating re-irradiation with more intensive systemic therapy using triplet induction consisting of docetaxel, cisplatin, and 5-fluorouracil (TPF), followed by re-irradiation with concurrent weekly docetaxel and cetuximab (Ngan et al. 2015). Preliminary outcome in 32 recruited patients showed encouraging 2-year OS of 67 %, but the toxicity rate was high [eight temporal lobe necrosis (TLN) and two fatal epistaxis were observed]. In addition, the tolerance to induction TPF was poor in this cohort of recurrent patients (five patients withdrew after the first cycle of TPF due to fatigue; and ≥ grade 3 neutropenia and hyponatremia occurred in 38 % and 28 %, respectively).
Four studies from China used re-irradiation dose of around 70 Gy. The largest study was reported by Han et al. (2012): a total of 239 patients (25 % rT1–2) were re-irradiated, and the mean total dose to GTV was 69.94 Gy and the mean dose per fraction was 2.31 Gy. The 5-year local relapse-free survival was 85 %, but the OS rate was only 45 % with 35 % who died of treatment-related death toxicities. Chen et al. (2013) showed similar findings in 54 recurrent NPC patients treated to an average GTV dose of 69.96 Gy. The 2-year local failure-free survival was 64 %, but the OS rate was only 44 % with 48 % of patients suffering from severe late toxicities, and 25 % died of treatment complications. Similarly, in the randomized phase 2 study reported by Tian et al. (2014) on two different fractionation schemes (60 Gy in 27 fractions vs. 68 Gy in 34 fractions), high incidence of treatment-related death was noted in both arms (24 % vs. 41 %). Furthermore, OS was in fact better for the group receiving 60 Gy in 27 fractions due to lower rate of treatment-related death.
8 Treatment Complications
Late toxicities are the main prohibitory factor for re-irradiation of NPC. Figure 1 illustrates some of the possible late treatment complications after re-irradiation. Other common toxicities include xerostomia, hearing impairment, trismus, cranial nerve palsy, and hypopituitarism. Detailed studies on structured QOL measurements are lacking.
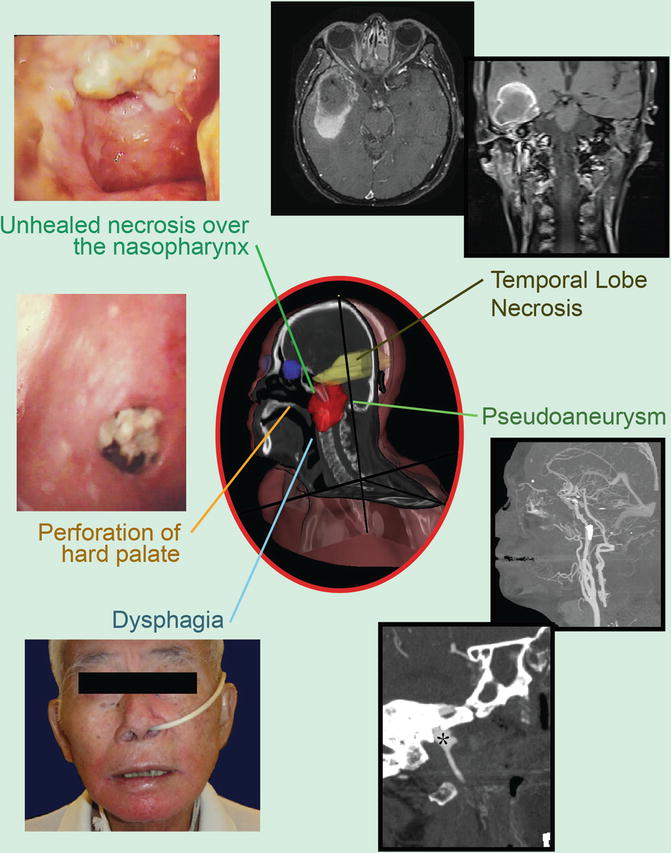
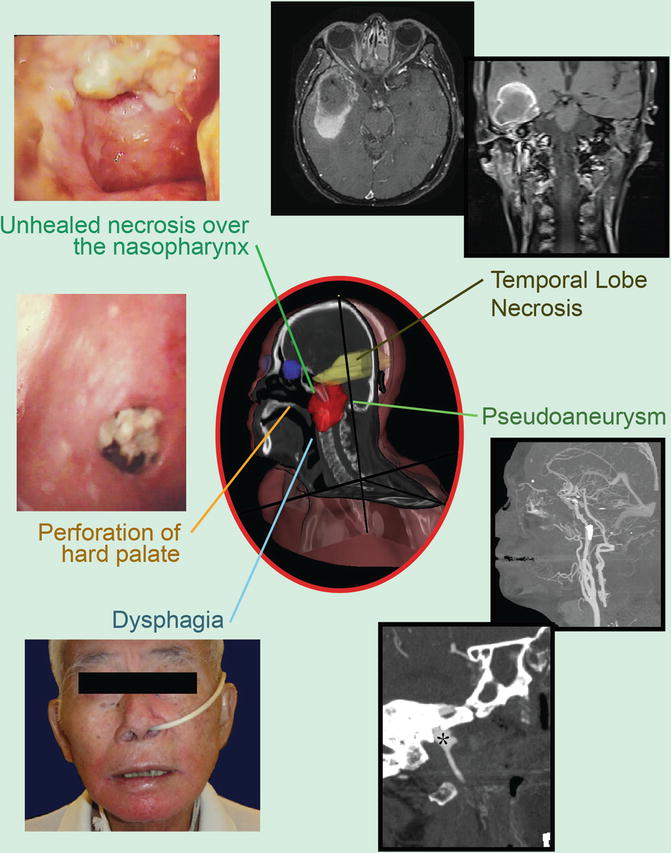
Fig. 1
Potential late complications due to re-irradiation: pseudoaneurysm (black asterisk)
8.1 Carotid Blowout
Carotid blowout is the most daunting complication among all and is the main cause of treatment-related death. Literature-based systematic review by McDonald et al. (2012) on 1554 patients reported a crude rate of carotid blowout after re-irradiation of the head and neck (H&N) region of 2.6, and 76 % of them died. But the reported hemorrhage rate following re-irradiation for NPC varied widely; the incidence following IMRT or SRT ranged from 0 to 25 % (Seo et al. 2009; Ozyigit et al. 2011; Chen et al. 2013; Benhaim et al. 2014). The total re-irradiation dose is the significant aggravating factor. In a phase 2 randomized study by Tian et al. (2014) comparing two IMRT dose regimens in recurrent NPC, the massive hemorrhage rate at a median follow-up of 25 months was 19 % in the group re-irradiated to 60 Gy in 27 fractions compared to 31 % in the group with 68 Gy in 34 fractions. Whether this is aggravated by combining RT with intensive systemic therapy is uncertain, interim analysis of the prospective phase 2 study by the Hong Kong NPC Study Group mentioned above reported a 14 % bleeding rate following 60 Gy plus intensive induction-concurrent therapy (Ngan et al. 2015).
8.2 Temporal Lobe Necrosis
TLN is another potentially life-threatening late toxicity. While some may be asymptomatic especially at early phase of development, others may suffer from debilitating symptoms (including headache, dizziness, memory loss, epilepsy, pressure symptoms, changes in conscious level, and occasional intracranial hemorrhage) (Lee et al. 2002). The incidence is much higher in cohorts after re-irradiation than single-course RT, ranging from 7 to 35 % (Chua et al. 2005a; Koutcher et al. 2010; Han et al. 2012; Chen et al. 2013; Tian et al. 2014; Ngan et al. 2015). Risk of TLN depends on fractional dose, cumulative dose, RT techniques, and time interval between two courses of RT (Lee et al. 1998; Bakst et al. 2011; Chen et al. 2011; Zhou et al. 2014). The study by Liu et al. (2014) on over 200 recurrent NPC patients re-irradiated to around 70 Gy revealed a 31 % risk of TLN with a median latency period of only 15 months; a maximum summated dose of less than 125Gy EQD2 and interval between courses of at least 2 years is recommended.
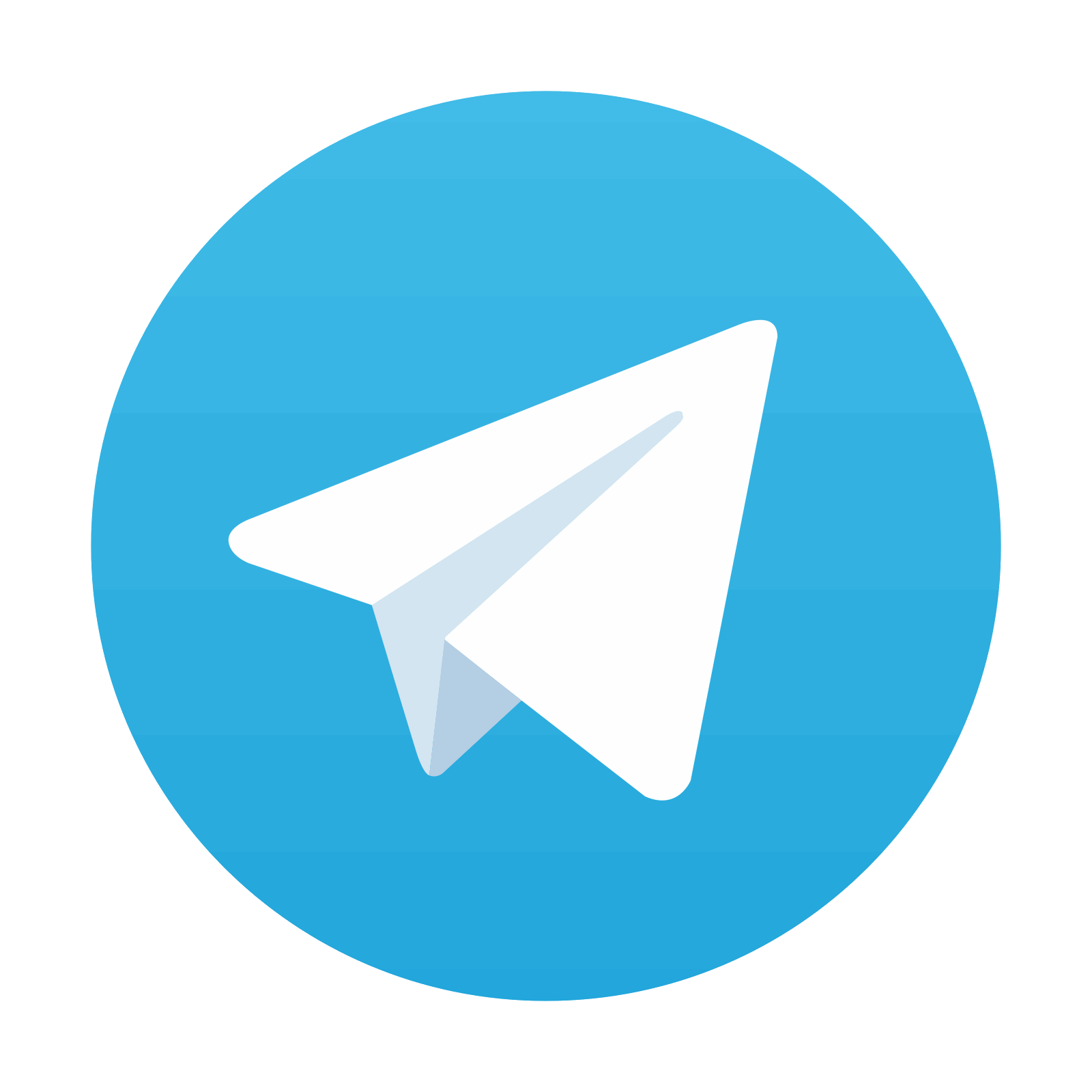
Stay updated, free articles. Join our Telegram channel

Full access? Get Clinical Tree
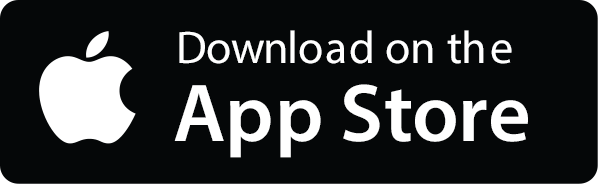
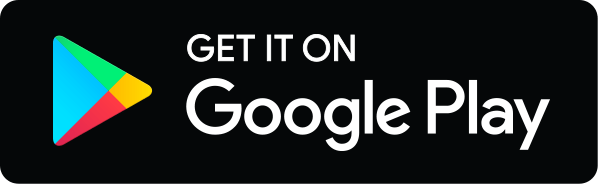