Author/study
PTV
Dose/fraction
Fractions
BED
CHT
MST
Floyd et al. [21]
GTV
5.0 Gy
10
75 Gy
No
7 m
n = 18
Sultanem et al. [22]
GTV
3.0 Gy
20
78 Gy
No
9.5 m
n = 25
Monjazeb et al. [23]
GTV + 5 mm
2.5 Gy
28
88 Gy
No
13.6 m
2.5 Gy
30
94 Gy
n = 21
2.5 Gy
32
100 Gy
Tsien et al. [24]
GTV + 5 mm
2.2 Gy
30
81 Gy
TMZ
20.1 m
n = 38
2.4 Gy
89 Gy
2.5 Gy
94 Gy
2.6 Gy
98 Gy
2.7 Gy
103 Gy
Panet-Raymond et al. [25]
GTV
3.0 Gy
20
78 Gy
TMZ
14.4 m
n = 35
Morganti et al. [26]
GTV + 1.5 cm
2.4 Gy
25
74 Gy
TMZ
20 m
2.5 Gy
25
78 Gy
n = 19
2.6 Gy
25
82 Gy
Chen et al. [27]
GTV + 5 mm
3.0 Gy
20
78 Gy
TMZ
16.2 m
n = 19
4.0 Gy
20
84 Gy
5.0 Gy
20
90 Gy
6.0 Gy
20
96 Gy
Reddy et al. [28]
GTV + 5 mm
6.0 Gy
10
96 Gy
TMZ
16.6 m
n = 24
Iuchi et al. [29]
GTV + 5 mm
8.5 Gy
8
126 Gy
TMZ
20.0 m
n = 46
7.3.2 Simultaneous Integrated Boost Technique
As mentioned above, GBM cells are highly infiltrative and have already spread widely into the surrounding brain at diagnosis. Although both the tumor bulk and infiltrating lesion should be included as treatment targets, their situations are different and a different treatment approach is required. The tumor bulk includes dens of tumor cells and few functioning neurons, which indicates the need for higher-dose RT and functional safety in this area. Tumor cells in this zone are suspected of being hypoxic, and dose escalation to overcome this problem is also expected. In contrast, the area of infiltration includes both tumor cells and functioning neurons, and differentiation of these cells owing to their different radiosensitivity is required. Tumor cells in this area are well oxygenated, and the required total radiation dose may be lower than that needed for the treatment of the tumor bulk. Less frequent distant failure after conventional RT also supports this approach; conventional dose delivery for the treatment of this area is desirable to spare the functioning neurons. Therefore, differing dose delivery is required to control both of these lesions. Using the simultaneous integrated boost technique, different doses can be delivered to layered targets. This technique is suitable for the treatment of infiltrative tumors including GBM. For GBM, two- or three-layered targets are usually settled, surrounding the enhancing tumor.
7.4 Immobilization System
The anatomic accuracy of IMRT depends on the reproducibility of the geometrical positioning of patients between the planning CT and treatment or during fractionated irradiations. Thermoplastic masks are widely used for noninvasive immobilization of patients during treatment (Fig. 7.1). However, we should be aware of the limitations of this immobilization system. Mask shrinkage after fabrication may have an effect on the immobilization of the patient, and flexibility during treatment may decrease reproducibility [31]. Head and shoulder masks, bite mouthpieces, and head and body cushions are employed to improve reproducibility, but the range of the interfractional displacement of the target has been reported as being 2.0–3.0 mm [32, 33]. This reproducibility error should be included in the margin surrounding the lesions during the settlement of the targets. Furthermore, the changes regarding the condition of the patient’s head after craniotomy, such as the presence of edema in the face and scalp, subcutaneous hematoma, and cerebrospinal fluid (CSF) leakage, also have an effect on the accuracy of immobilization. Therefore, immobilization devices should be fabricated after improvement in these postsurgical changes. Indeed, such an approach would mean a delay in the initiation of postsurgical RT; however, an improved outcome for patients with GBM has been reported after the initiation of RT at >4 weeks after surgery, probably owing to reoxygenation after surgical procedures [34].
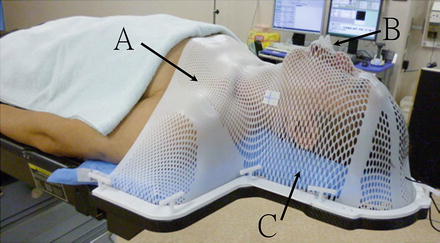
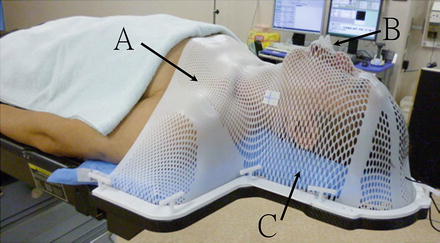
Fig. 7.1
Noninvasive immobilization system. Thermoplastic head and shoulder mask (A), bite mouthpieces (B), and head and body cushions (C) are used to improve the reproducibility of treatment
Before each treatment, megavoltage electronic portal images are generated and bony landmarks are used to ensure geometric accuracy. Correction of the position of the head is performed by adjustment of the treatment table in three dimensions prior to treatment (Fig. 7.2).
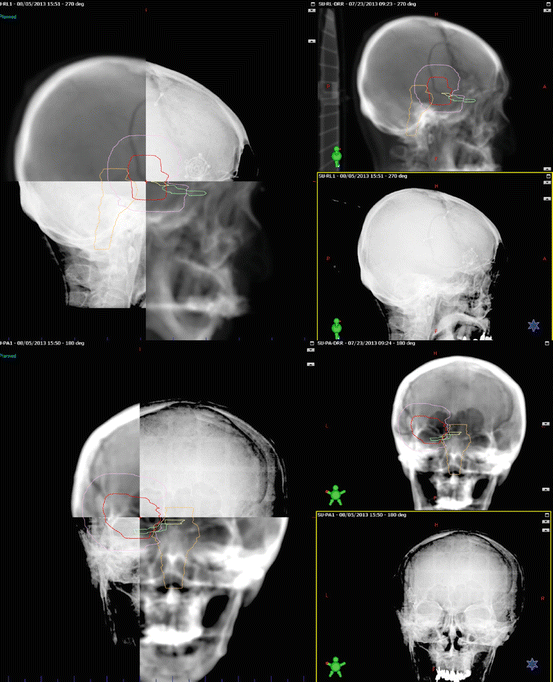
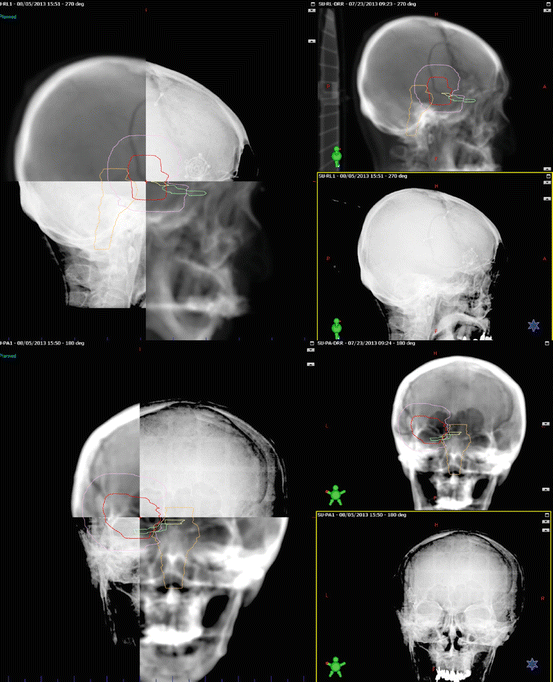
Fig. 7.2
Megavoltage electronic portal images are generated and bony landmarks are used to ensure geometric accuracy before each treatment
7.5 Target Delineation
7.5.1 Target Delineation on CT/MRI
After fabrication of the facial mask, thin slice CT is performed for planning. The data from the CT images are also used for absorption correction during the planning of dose delivery. For target delineation, MRIs are also obtained and merged into the planning CT. Target volumes are contoured on these images in line with the International Committee on Radiation Units and Measurements (ICRU) reports 50 and 62 [35, 36]. Gross tumor volume (GTV) is usually defined as the enhanced lesion on T1-weighted MR images and the surgical cavity. In the treatment of patients with GBM, a simultaneous integrated boost technique is usually employed, and layered clinical target volumes (CTVs) are settled to cover both the tumor bulk and microscopic spread of the tumor. Because the dominant pattern of failure is local after standard GBM treatment, higher dose delivery to the regional tumor is required. Therefore, the CTV of the tumor bulk (CTVb) is defined as being equal to the GTV. GBM is a highly infiltrative tumor, and migration of tumor cells into the surrounding brain should also be included in the CTV. Two different approaches exist regarding the definition of the CTV for the infiltrating area (CTVi). The first approach is based on the experiences of treatment failure after conventional RT. The majority of the recurrences have been reported to arise within 15–20 mm of the tissue surrounding the enhanced lesion, and the CTVi may be defined as the expansion of the GTV to encompass the surrounding 15–20 mm zone. This expansion is automatically performed in the planning software without consideration of the anatomical spread of tumor cells. Therefore, excessive volume should be manually removed from the CTVi after automatic expansion (Fig. 7.3). The other approach regarding the definition of the CTVi is based upon the estimated microscopic spread of tumors cells. As described above, the volume of the edema surrounding the enhancing lesion includes microscopically detected migrating tumor cells and is also defined as the CTVi. MRIs of both the T2-weighted images and fluid-attenuated inversion recovery (FLAIR) images are used to visualize edema. Although discordance between CTVs based upon T2-weighted and FLAIR images has been reported [37], FLAIR images are more frequently employed because the CSF is also visualized brightly on T2-weighted images and may impair the visualization of edema. After contouring the CTVs, the planning target volume (PTV) is defined by expanding the CTV with a 3.0–5.0 mm margin, which includes the immobilization and treatment errors.
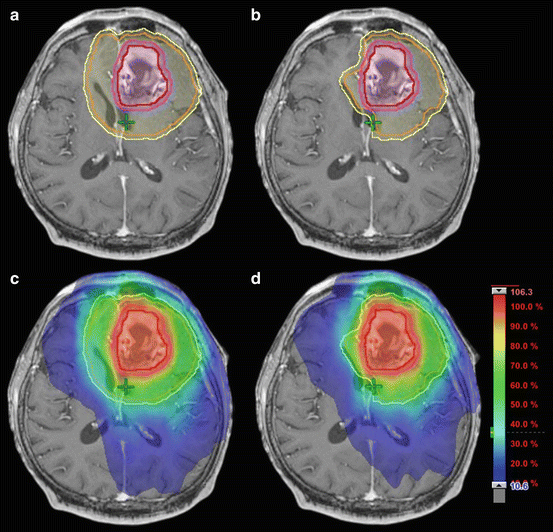
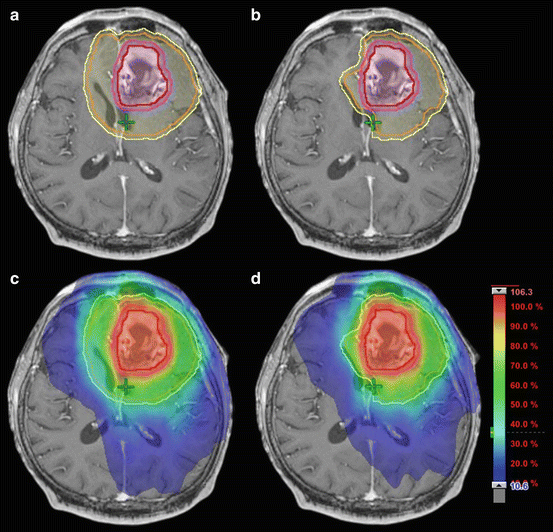
Fig. 7.3
Target delineations and dose deliveries with and without consideration of anatomical spread of tumor cells. The clinical target volume for the infiltrating area (CTVi; orange line) was contoured automatically by expanding it by a distance of 20 mm from the gross tumor volume (GTV; red line) (a). However, this automatic expansion did not consider the anatomical spread of tumor cells. In this case, tumor cells could infiltrate only via the corpus callosum to the contralateral frontal lobe and could not move across the falx. Furthermore, the ventricle wall blocked the way of tumor cells to the caudate head. Therefore, the excessive volumes expanded across the falx and the ventricle was removed from the CTVi (b). After contouring the CTVs, PTVb for tumor bulk (pink line) and PTVi for the infiltrating tumor (yellow line) were defined by expanding the GTV and CTVi with a 3 mm margin. Prescribed doses of 68 Gy for PTVb and 40 Gy for PTVi were delivered by 8 fractions. The different distributions of dose delivery before (c) and after removal of excessive volume from the CTVi (d) were also demonstrated. This difference indicated the significance of anatomical consideration during delineation of the CTVs to minimize the dose for the surrounding normal brain
7.5.2 Target Delineation Based upon Biological Imaging
Although MRIs provide precise geometrical information, they do not directly visualize the location of tumor cells. The contrast-enhanced area is usually diagnosed as tumor bulk, but enhancement is only the result of leakage of contrast medium through the impaired brain-blood barrier (BBB) of tumor vessels. The high-intensity area on T2-weighted or FLAIR images may include infiltrating tumor cells, but this does not mean that migrating tumor cells exist anywhere in these areas. Furthermore, induced neovascularization as a physiological reaction after surgery also causes contrast enhancement, and changes in the blood circulation after surgery also cause an increase in the intensity of normal brain surrounding the surgical cavity on T2-weighted or FLAIR images. Therefore, CTVs contoured on these images may include the area without the need for dose delivery. To decrease the excessive radiation delivery to the critical structures adjacent to the target, biological imaging is required for the delineation of real targets.
Amino acid (AA) positron emission tomography (PET) has been tried to improve the delineation of the distribution of glioma cells. l-[methyl-11C]methionine (MET) and O-(2-[18F]fluoroethyl)-l-tyrosine (FET) are the most widely used tracers. These tracers are transported across the BBB by means of the membrane transport system, and the accumulation of these tracers reflects the protein synthesis taking place in tumor cells. The uptake of these tracers is not dependent on the disruption of the BBB, and the significantly higher accuracy of AA-PET relative to CT and MRI in defining the distribution of tumor cells has been reported [38–43]. MET-PET and FET-PET provide comparable diagnostic information for the delineation of tumors [44], even though the short half-life of 11C (20 min) limits the use of MET-PET to institutions with an onsite cyclotron. In the majority of the primary cases, the AA tracer accumulates beyond the enhanced lesion and within the high-intensity area on T2-weighted or FLAIR MRIs (Fig. 7.4). Although target delineation based upon these accurate images will contribute to the sparing of neurological functions in patients after IMRT, the major remaining problem regarding the use of PET information is the threshold of uptake. The uptake of AA is semiquantitatively expressed by the ratio of uptake in the lesion to that in the contralateral normal brain (T/N ratio). However, limited data is available regarding the optimal value of the T/N ratio required for drawing the target volume and the optimal radiation dose for the control of these areas.
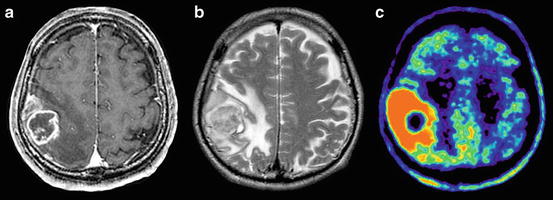
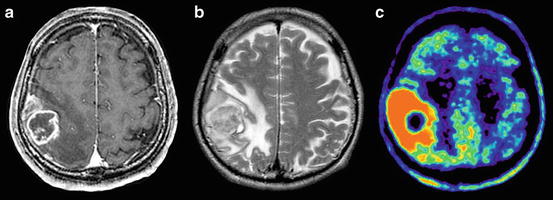
Fig. 7.4
Different visualizations of tumor on enhanced MRI (a), FLAIR image (b), and methionine-PET (c)
On the other hand, PET is also used to define the radioresistant area in the tumor. Hypoxia is one of the major causes of radioresistance in GBMs, but heterogeneous distribution of hypoxic cells has also been reported [45, 46]. Although some limitations remain, the significance of [18F]FMISO-PET in the visualization of the hypoxic area in gliomas has been reported [47, 48]. In the treatment of head and neck cancers, [60Cu]ATSM-PET- and [18F]FMISO-PET-guided radiotherapy planning have been reported [49, 50], and these images may also contribute to the treatment of GBM in the future.
7.5.3 Organ at Risk (OAR)
As the prognosis of patients with GBM is poor, some oncologists may feel little need for the preservation of critical structures from late toxicities [51]. However, temozolomide prolongs the survival of GBM patients, and the 5-year survival rates have improved to 13.8 % for patients with the methylated O-6-methylguanine-DNA methyltransferase (MGMT) gene [4]. Therefore, the issue of late toxicity has become more significant.
In treatment planning for brain tumors, OARs include the eye lens, retina, optic nerve, chiasm, cochlea, and brain stem. A study by Emami et al. in 1991 was the first to summarize the clinical experience regarding partial organ tolerance doses for OARs [52]. More recently, QUANTEC (quantitative analysis of normal tissue effects in the clinic) articles have updated/refined these data (Table 7.2) [53–56]. As these data are based upon clinical outcomes after conventional fractionated radiotherapy or radiosurgery involving single fraction treatment, the tolerance doses for OARs have been estimated from these data using the LQ model. However, the reliability of the LQ model and the value of the α/β ratio are still debated in hypofractionated RT (see Chap. 4), and further investigations are required to define the tolerance doses for OARs.
Table 7.2
Approximate dose/volume/outcome data for critical organs following conventionally fractionated radiotherapy and stereotactic radiosurgery
Organ | End point | Irradiation | Dose | Rate | |
---|---|---|---|---|---|
Eye, lens | Cataract | Whole organ | 10 Gy | 5 %a | [51] |
18 Gy | 50 %a | [51] | |||
Eye, retina | Blindness | Whole organ | 45 Gy | 5 %a | [51] |
65 Gy | 50 %a | [51] | |||
Optic nerve/chiasm | Optic neuropathy | Whole organ | 50 Gy | 5 %a | [51] |
65 Gy | 50 %a | [51] | |||
3D-CRT | Dmax <55 Gy | <3 %b | [53] | ||
Dmax 55–60 Gy | 3–7 %b | [53] | |||
Dmax >60 Gy | >7–20 %b | [53] | |||
SRS | Dmax <12 Gy | <10 %b | [53] | ||
Cochlea | Hearing loss | 3D-CRT | Mean dose ≤45 Gy | <30 %b | [54] |
SRS | ≤14 Gy | <25 %b | [54] | ||
Ear, mid/external | Acute otitis | Whole organ | 30 Gy | 5 %a | [51] |
40 Gy | 50 %a | [51] | |||
Chronic otitis | Whole organ | 55 Gy | 5 %a | [51] | |
65 Gy | 50 %a | [51] | |||
Brain stem | Necrosis, infarction | Whole organ | 50 Gy | 5 %a | [51] |
65 Gy | 50 %a | [51] | |||
Neuropathy/necrosis | Whole organ | Dmax <54 Gy | <5 %b | [55] | |
3D-CRT | D1-10 cc ≤59 Gy | <5 %b | [55] | ||
Dmax <64 Gy | <5 %b | [55] | |||
SRS | Dmax <12.5 Gy | <5 %b | [55] |
7.6 Dose Delivery
7.6.1 Inverse Planning
After contouring the PTVs and OARs, the prescribed doses and the tolerance doses are inputted into the inverse planning system, and the dose distribution calculated automatically. In cases where the tumor exists adjacent to the critical organs, it may be difficult to deliver the prescribed doses to the PTV homogeneously while keeping the doses to the OARs below the limit of the tolerance doses. In such cases, the preferred dose delivery/avoidance should be decided individually based upon the estimated prognosis and functions of the patient.
7.6.2 Coplanar and Noncoplanar Beam IMRT
Although IMRT has improved the conformity and homogeneity of dose delivery to targets, especially concave tumors, conventional coplanar beam IMRT still has limitations with regard to the sparing of critical organs. In the treatment of tumors located in the posterior or temporal fossa, PTVs and OARs (optic nerve, chiasm, brain stem, and cochlea) may be included in the same axial plane, and the coplanar beam cannot completely avoid critical organs (Fig. 7.5a) [57]. In such cases, noncoplanar beam arrangement may decrease the doses to the OARs (Fig. 7.5b) [58].
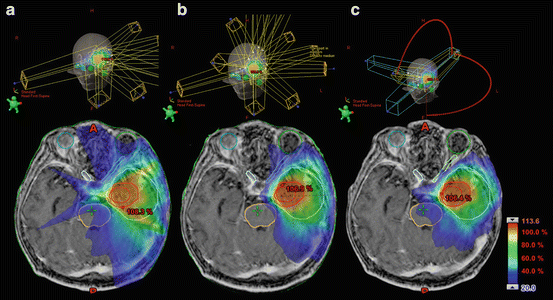
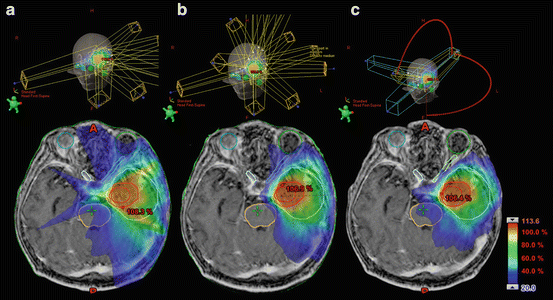
Fig. 7.5
Dose plans for a patient with glioblastoma located in the left temporal fossa. With coplanar planning, the angles of the fields were limited to avoid the organs at risks (OARs) and the isodose curves were stretched along the gantry angles (a). Noncoplanar beam arrangement improved the heterogeneity of dose delivery in the PTVs and decreased the dose to the OARs (b), even though 11 fields were required for this plan. On the other hand, an excellent distribution of doses was achieved using volumetric-modulated arc therapy (VMAT) with only two arcs of noncoplanar beams (c). In addition to the improvement of conformity and homogeneity of dose delivery, beam-on times and monitor units (MU) were decreased using VMAT. Beam-on times in coplanar IMRT, noncoplanar IMRT, and noncoplanar VMAT were 6.9, 8.1, and 3.5 min, respectively, and MU values were 3,430, 4,040, and 2,120, respectively. Prescribed doses were 68 Gy/8 fractions for PTVb (GTV + 5 mm) and 40 Gy/8 fractions for PTVi (GTV + 20 mm)
7.6.3 Volumetric-Modulated Arc Therapy (VMAT)
IMRT is usually performed using multiple static intensity-modulated beams. However, using static fields, the isodose curves in the range of lower doses extend along the gantry angle [58]. This “tail” of the low-dose field extends more sharply and over a greater distance when the angle or number of fields is limited to decrease the doses to the OARs adjacent to the PTV (Fig. 7.5a). Recently, VMAT, a rotating IMRT, has also become available for the treatment of patients with GBMs. With this method, a more homogeneous distribution can be achieved, not only in the low-dose field but also in the PTV, while maintaining a decreased dose to the OARs (Fig. 7.5c). The range of rotation can be changed and a noncoplanar arc can also be made available to further decrease the doses to the critical organs.
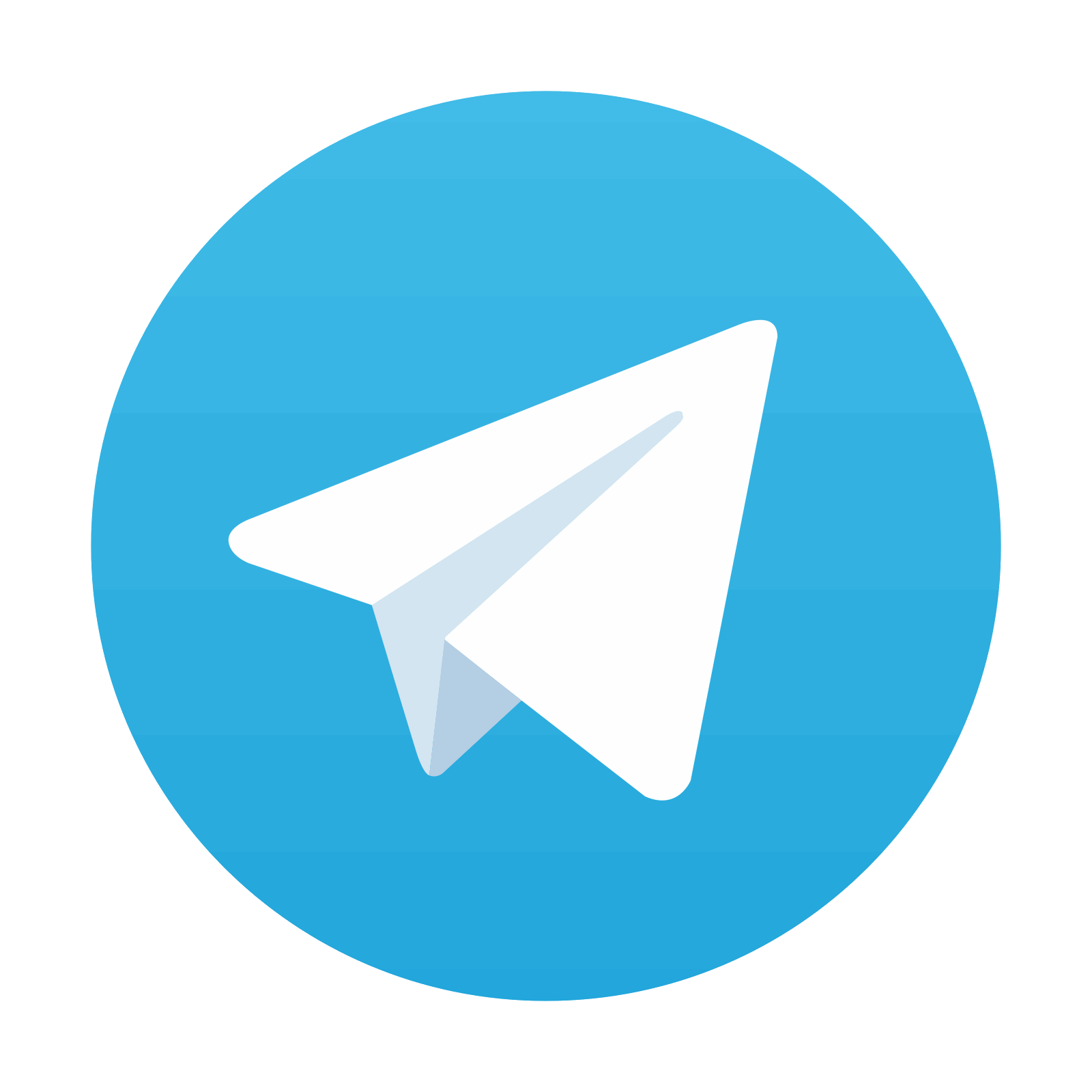
Stay updated, free articles. Join our Telegram channel

Full access? Get Clinical Tree
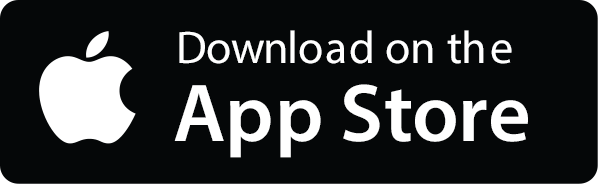
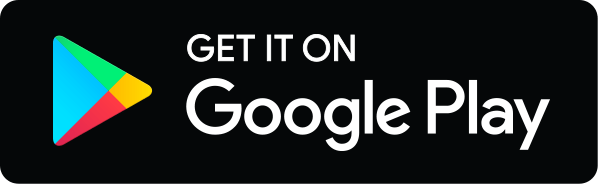