Cerebral Aneurysms
Incidence and Natural History
The incidence of a cerebral aneurysm in the general population is 0.5% to 5%. Women more commonly harbor a cerebral aneurysm than men, and aneurysm development increases with advancing age. Some families demonstrate a genetic predisposition to develop cerebral aneurysms, with a prevalence of 4% when a first-degree relative has a confirmed aneurysm, increasing to 8% if two first-degree relatives have aneurysms. Twenty percent of patients with unruptured cerebral aneurysms have a family member with an aneurysm or a history of subarachnoid hemorrhage. A genetic propensity without confirmed genetic locus mutation to develop cerebral aneurysms can be found in some families without known syndromic disorders.
The risk of new aneurysm development in patients with previously treated or untreated cerebral aneurysms is approximately 0.7% to 2% per year. Subarachnoid hemorrhage is the most common presenting sign of a cerebral aneurysm, and a cerebral aneurysm is the second most common cause of subarachnoid hemorrhage, trauma being the most common etiology. From 80% to 90% of nontraumatic subarachnoid hemorrhages are due to a ruptured cerebral aneurysm, a far more common cause than an arteriovenous malformation (AVM), which accounts for 5% of subarachnoid hemorrhages. Other less common presenting signs and symptoms include cranial nerve palsy, headache, seizure, and transient or permanent neurologic dysfunction that may be due to mass effect, neighboring inflammation, aneurysm thrombosis, or aneurysm-generated emboli. These nonhemorrhagic symptoms can be seen with aneurysms of any size but occur more commonly with larger aneurysms and those in the posterior circulation or located along the internal carotid artery.
Ruptured Cerebral Aneurysm
The annual incidence of rupture of an asymptomatic cerebral aneurysm has been estimated at 0.5% to 2%, or roughly 7 to 10 cases per 100,000 population. The rate of aneurysm rupture is cumulative over decades, so there is a 10% risk after 10 years and a 30% risk after 30 years. Symptomatic intact aneurysms have a higher rate of bleeding—4% or more per year. Some studies have suggested that for aneurysms less than 7 to 10 mm in diameter, the incidence of annual rupture is less than 0.05% to 0.7%. Other reports, however, have documented that 70% to 85% of ruptured aneurysms are less than 9 to 10 mm in diameter, and that even small (2-3 mm) aneurysms may cause subarachnoid hemorrhage. The larger the aneurysm, the greater the risk of rupture, with large aneurysms (10-24 mm) having a hazard ratio of 9, and giant aneurysms (≥25 mm) having a hazard ratio of 76 compared with a 3- to 4-mm aneurysm. Several arbitrary size classification systems designate aneurysms as small, large, or giant, the medium category having been neglected. The presence of a daughter sac, multilobulation, irregular surface, a tubular or bottleneck shape (increased aspect ratio or aneurysm height-to-neck ratio), increased size or volume orifice area ratios (ratio of maximum aneurysm diameter to parent vessel diameter; ratio of aneurysm volume to neck area), and interval growth probably raise the likelihood of rupture. Smoking and larger initial aneurysm size are associated with interval aneurysm growth. Anterior and posterior communicating aneurysms are almost twice as likely to rupture as aneurysms in other locations. In addition, vertebral basilar and posterior cerebral aneurysms carry a significant risk of rupture. A second unruptured aneurysm discovered in a patient with subarachnoid hemorrhage has an increased risk of causing a future second subarachnoid hemorrhage. Patients with a treated ruptured cerebral aneurysm have an increased incidence of new aneurysm formation and recurrent subarachnoid hemorrhage. Younger patients and women have a greater propensity for aneurysmal hemorrhage, especially if they have multiple aneurysms, although statistically a patient with a ruptured aneurysm is more likely to be of increased age. Some of the factors implicated in aneurysm rupture include a positive family history of aneurysmal subarachnoid hemorrhage, smoking and exposure to smoke, hypertension including variable episodes of blood pressure elevation, excessive alcohol intake, use of stimulants and vasoconstricting agents, excessive caffeine ingestion, specific genetic syndromes (see following), and extreme straining or Valsalva maneuvers including sexual intercourse. Smoking and positive family history may be additive. The majority of cerebral aneurysms, however, do not bleed, and there is no convincing evidence that pregnancy and childbirth are associated with aneurysm rupture.
A ruptured cerebral aneurysm carries significant morbidity and mortality, with the 30-day mortality traditionally being stated as 40% to 65% ; 12% to 15% expire before reaching medical care. Older age, severe comorbidities, worse presentation grade ( Tables 12-1 and 12-2 ), large amount of subarachnoid hemorrhage, intraventricular or intraparenchymal hemorrhage, and global cerebral edema predict worse outcome. Untreated patients with ruptured cerebral aneurysms have a rebleeding rate of 2% to 4% in the first 24 hours and then 15% to 20% in the first 2 weeks. Thereafter the bleeding rate is approximately 3% per year. The risk of rebleeding is 10 times higher in the 10% to 40% of patients with a sentinel aneurysmal bleed, a symptom most often deduced as a preceding headache that in some instances can quickly abate. Rebleeding carries a 50% to 67% mortality. Initial aneurysm size is not a prognostic factor influencing rebleeding in the acute period. Ruptured aneurysms typically increase in size if left untreated.
Grade | Characteristics |
---|---|
I | Asymptomatic or minimal headache |
II | Moderate to severe headache, nuchal rigidity, oculomotor palsy, normal level of consciousness |
III | Confusion, drowsiness, mild focal neurologic deficit |
IV | Stupor or hemiparesis |
V | Comatose or moribund with decerebrate posturing |
Grade | Glasgow Coma Scale Score | Clinical Evaluation |
---|---|---|
1 | 15 | No motor deficit |
2 | 13-14 | No motor deficit |
3 | 13-14 | Motor deficit |
4 | 7-12 | ± Motor deficit |
5 | 3-6 | ± Motor deficit |
From 21% to 25% of patients who enter the hospital for treatment of subarachnoid hemorrhage eventually die as a result of the condition. Approximately 14% to 23% of survivors of a ruptured cerebral aneurysm are left with significant morbidity ; in 13% to 32% the morbidity is the direct result of delayed vasospasm-caused ischemia. More than half to two thirds will be able to resume independent living and return to work, one third requiring lifetime care. Some 20% to 45% are left with long-lasting cognitive deficits, and more than 50% have more subtle residual memory, emotional, or neuropsychological impairments. Surgical clipping of acutely ruptured aneurysms results in improved functional outcome compared with delayed intervention. These data also argue for elective treatment of an unruptured aneurysm.
Unruptured Cerebral Aneurysm
Unruptured cerebral aneurysms are most often asymptomatic, identified incidentally on a noninvasive imaging study. Focal mass effect can manifest as compression of adjacent cranial nerves or brain parenchyma, a typical example being third-nerve palsy caused by a posterior communicating aneurysm. Other diseases, however, can cause similar neurologic deficits. For example, third-nerve palsy could be caused by diabetes or microvascular disease instead of a posterior communicating aneurysm. When magnetic resonance imaging (MRI), MR angiography (MRA), or computed tomography angiography (CTA) fail to diagnose the cause of the patient’s third-nerve palsy, catheter cerebral angiography may be required to exclude a cerebral aneurysm, because the morbidity and mortality associated with cerebral aneurysms are so significant. When catheter cerebral angiography does not demonstrate an aneurysm in such a patient with no history of subarachnoid hemorrhage, the patient can be presumed to have small-vessel cerebrovascular disease. Sometimes the angiogram supports this diagnosis by showing other areas of atherosclerotic disease.
Growth and (potentially) rupture of a cerebral aneurysm has been linked to untreated or variably elevated systolic blood pressure, smoking, female sex, age older than 74, aneurysm location in the internal carotid artery or middle cerebral artery, aneurysm diameter of 7 mm or greater, and multiple cerebral aneurysms.
Types of Aneurysms, Etiology, and Pathology
Aneurysms may be classified by etiology and characterized according to morphology, size, or location. Most cerebral aneurysms are acquired lesions and are saccular or fusiform. Older medical reports proposed that saccular or berry cerebral aneurysms were due to an embryologic abnormality of the walls of cerebral vessels. Stehbens and others have refuted this theory. Cerebral aneurysms can develop from hemodynamic stresses, which typically occur at vessel bifurcations or terminations or along the outer curvature of looping vessels. The aneurysm’s location is a continuation of the primary flow direction in the parent vessel, with greater prevalence in tortuous arteries and increased arterial bifurcation angles. Rupture and thrombosis of cerebral aneurysms may also be related to hemodynamic forces. Supporting this theory is the higher incidence of cerebral aneurysms in patients with intracranial vascular anomalies, including fenestrations and congenital or iatrogenic vascular variants of the circle of Willis (e.g., persistent trigeminal artery, unilateral absence or hypoplasia of the A1 segment of an anterior cerebral artery [ Fig. 12-1 ], azygos anterior cerebral artery A2 segment [ Fig. 12-2 ; also see Fig. 12-5 ], and occlusion or absence of an internal carotid artery). Disturbed or unstable blood flow patterns, resonant frequency–generated vibrations, oscillatory wall shear stress, transmural pressure, and entrance and internal aneurysmal flow rates and patterns—many characteristics investigated by computational fluid dynamics—are proposed mechanisms of aneurysm development and rupture (see Fig. 12-30 ). Supporting this reasoning is that flow-generated aneurysms associated with cerebral AVMs have an increased risk of rupture. There is no significant pressure difference within aneurysms compared with parent arteries, but intraaneurysmal flow velocities are lower; wall shear stress analysis is complex. Regions of relative flow stagnation have been linked to aneurysm growth and rupture.
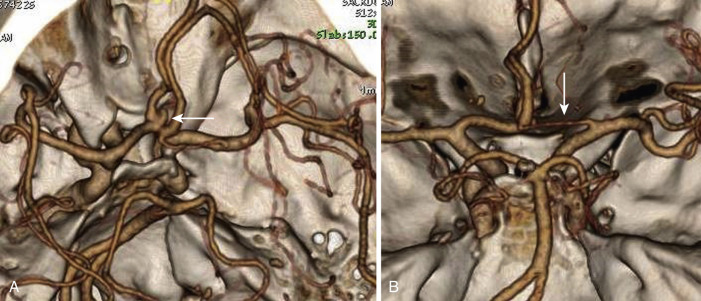
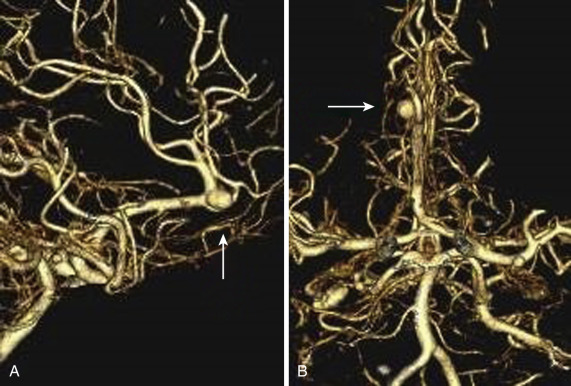
Fusiform aneurysms are vascular ectasias caused by advanced focal atherosclerotic disease that has degraded the vessel’s media. The vertebrobasilar system is more commonly affected than the anterior circulation ( Fig. 12-3 ). The involved vessel is frequently elongated and tortuous, so in effect the native vessel is replaced by aneurysmal dilatation. Although fusiform aneurysms may rupture, thrombosis due to blood stagnation may be the mode of presentation. Local mass effect, neighboring tissue edema, wall calcification, ischemia due to penetrating vessel occlusion or emboli generated by mural thrombus (see Fig. 12-3C ), and cranial nerve palsies are other pathologic sequelae. Rarely, fusiform aneurysms can form giant serpentine aneurysms due to repeated thrombosis and recanalization.
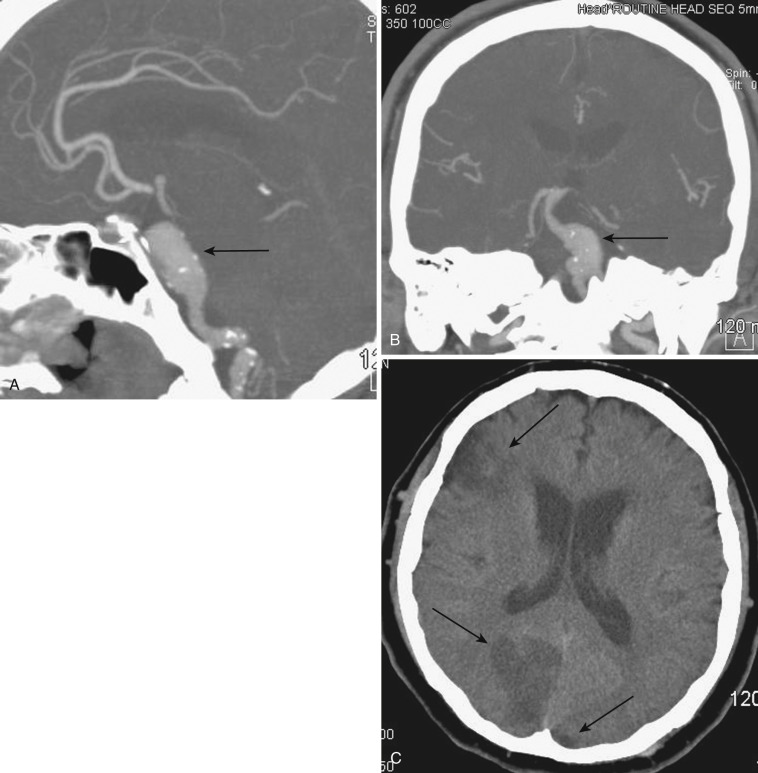
An infundibulum represents the residua of a developmental vessel that has undergone incomplete regression. It most commonly involves the junction of the internal carotid artery and posterior communicating artery and less commonly involves the origin of the anterior choroidal artery, ophthalmic artery, middle cerebral branch, a lenticulostriate or thalamoperforating artery, or rarely other intracranial artery origins ( Fig. 12-4 ). Infundibula are usually 3 mm or less in diameter. They can be difficult to differentiate from cerebral aneurysms unless the characteristic origination of the emerging vessel from the summit of the dome of the infundibulum is identified. Infundibula have rarely been reported to cause subarachnoid hemorrhage.
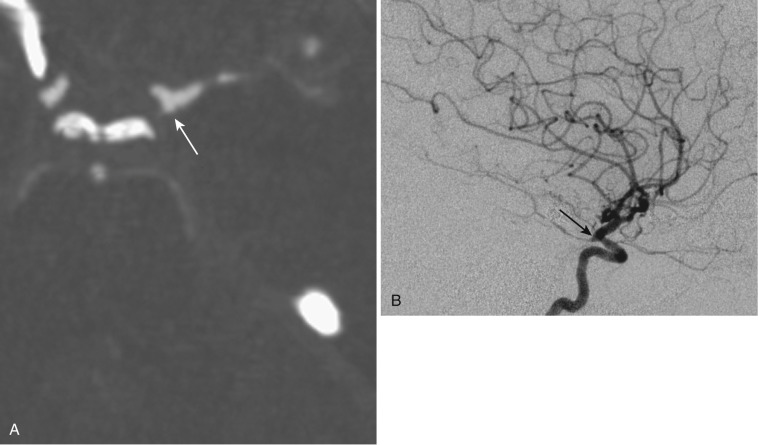
Etiology
Leading causes of cerebral aneurysms are:
- •
Atherosclerosis
- •
Hypertension
- •
Smoking
- •
Familial genetic predisposition, sometimes transmitted as autosomal dominant transmission, with a reported incidence of 17.9%
- •
Abuse of cocaine, methamphetamine, ephedrine, heroin, and other drugs that produce arteritis or hypertension ( Fig. 12-5 )
FIG 12-5
Sagittal CTA demonstrating a pericallosal aneurysm (arrow) in a cocaine user with an azygous A2 segment of the anterior cerebral arteries.
- •
Vascular malformations (≈6%-10% incidence of aneurysm, both proximal flow–related and nidal) ( Fig. 12-6 ), and moyamoya disease
FIG 12-6
Catheter cerebral angiogram, AP view, of a right middle cerebral territory AVM with three flow-related aneurysms (arrows).
- •
Specific vascular diseases and syndromes, such as spontaneous cervical carotid or vertebral artery dissection (more commonly seen in women), spontaneous or posttraumatic intracranial dissection, polycystic kidney disease (10%-22.5% incidence of aneurysm, increasing with age ; see Fig. 12-1 ), α 1 -antitrypsin deficiency, coarctation of the aorta (10% incidence), bicuspid aortic valve (10% incidence), neurofibromatosis type 1 (von Recklinghausen’s disease), hereditary hemorrhagic telangiectasia (HHT, Osler-Weber-Rendu disease; 2.5% incidence), and Takayasu’s arteritis
- •
Connective tissue disorders, such as fibromuscular dysplasia (20%-50% incidence of aneurysm, more commonly seen in women) ( Fig. 12-7 ), Marfan’s syndrome, pseudoxanthoma elasticum, and Ehlers-Danlos syndrome type IV (in which aneurysms of the carotid siphon to supraclinoid segment are more common) ( Fig. 12-8 )
FIG 12-7
Woman with carotid fibromuscular dysplasia seen on the CTA sagittal reconstruction of the left carotid ( arrow in A ), better illustrated on the cervical lateral view of the catheter left carotid angiogram ( arrow in B ), and also indicated in the right carotid artery on the lateral right carotid cerebral angiogram ( white arrow in C ) that also demonstrates a small ruptured right posterior communicating aneurysm ( black arrow in C ).
FIG 12-8
Patient with genetically confirmed Ehlers-Danlos syndrome and new-onset headache. A, Axial CTA section documenting a new pseudoaneurysm (arrow) involving the subarachnoid intracranial right vertebral artery, not seen on a prior CTA ( B ) (arrow). Note the interval apparent vertebral artery lumen enlargement. C, Lateral oblique view of the right vertebral cerebral catheter angiogram confirming the pseudoaneurysm (arrow) located proximal to posterior inferior cerebellar artery (PICA). The widened vertebral artery segment proximal to the pseudoaneurysm indicates this is a dissection.
- •
Inflammation or mycosis due to septic emboli causing destruction of the endothelium, spread of infection to the vasa vasorum with subsequent vessel wall destruction and focal dilatation, or local infectious/inflammatory vessel wall invasion (mycotic or infectious aneurysm) ( Fig. 12-9 )
FIG 12-9
CTA demonstrating a distal posterior cerebral artery territory mycotic pseudoaneurysm (arrow) with adjacent parenchymal hematoma in a patient with mitral valve vegetations.
- •
Neoplasm, especially pituitary adenoma
- •
Penetrating and nonpenetrating trauma
- •
Radiation ( Fig. 12-10 )
FIG 12-10
Axial image of a CTA ( A ) revealing a small left supraclinoid carotid segment aneurysm (black arrow) that had produced subarachnoid hemorrhage in a patient with previous radiation therapy for a suprasellar mass as a child. Note the right craniotomy and right suprasellar subarachnoid catheter (white arrow). B, 3D reconstruction image from a rotational left carotid catheter cerebral angiogram confirms the aneurysm (arrow).
Hypertension and smoking may be additive causal factors. Estrogen deficiency as a hormonal causative factor has been proposed. Rarely cerebral aneurysms may be congenital. Except for congenital aneurysms, aneurysms with uncommon causes often develop in unusual locations that are distal to the circle of Willis.
Dissection.
Dissection of the internal carotid or vertebral arteries may be intracranial or extracranial and can be spontaneous, posttraumatic, or secondary to disease of the vessel wall, such as with fibromuscular dysplasia. A pseudoaneurysm accompanies up to one third of internal carotid dissections and 7% of vertebral artery dissections ( Fig. 12-11 ). Dissections and dissecting pseudoaneurysms may manifest as ischemic symptoms due to emboli or vessel stenosis or occlusion. Intracranial dissections and dissecting pseudoaneurysms most often present in young to middle-aged men and often present as subarachnoid hemorrhage, especially when occurring in typically involved vertebral or posterior inferior cerebellar arteries. Rebleed rates are particularly high, with accompanying significant mortality reaching 80%. Dissections with or without pseudoaneurysms can less commonly involve the basilar, posterior cerebral, or middle cerebral arteries. A blister-type aneurysm may represent a small focal dissection, most commonly involving the proximal intracranial internal carotid artery. Connective tissue disorders, fibromuscular dysplasia, and α 1 -antitrypsin deficiency have an increased incidence of dissections and cerebral aneurysms.
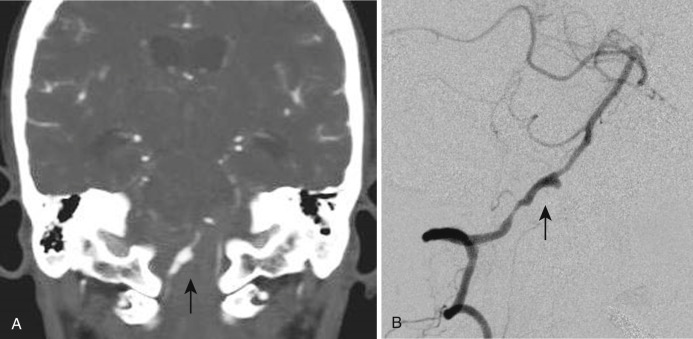
Trauma.
Trauma typically results in a pseudoaneurysm rather than a true aneurysm, because no normal vascular components constitute the sac within a hematoma surrounding the injured vessel. Posttraumatic pseudoaneurysms due to blunt trauma often occur in unusual locations, including the proximal skull base (internal carotid artery), posterior or anterior cerebral arteries (caused by tentorial or falcine impaction, respectively), and meningeal or scalp arteries. Penetrating head trauma near the circle of Willis predicts arterial injury and pseudoaneurysm formation. Traumatic pseudoaneurysms may not be visualized initially but then can rapidly enlarge ( Fig. 12-12 ) ; 50% of untreated posttraumatic aneurysms bleed, with an almost uniformly fatal outcome. Traumatic pseudoaneurysms may also be iatrogenic.
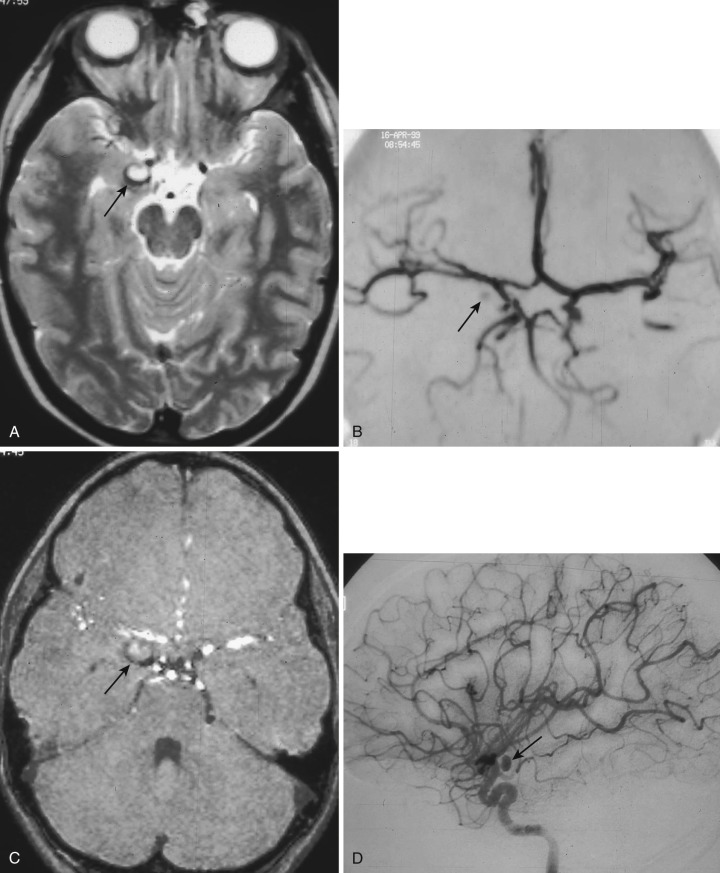
Neoplasm.
Uncommon neoplastic aneurysms (e.g., cardiac myxomatous aneurysms) are pathologically pseudoaneurysms resulting from primary or metastatic tumor invasion of a vessel, or metastatic emboli to the vessel lumen or its vasa vasorum. Pituitary adenomas are associated with a higher incidence of cerebral aneurysms, with acromegaly-producing adenomas theorized to sometimes produce diffuse cerebrovascular ectasia or unilateral or bilateral cavernous carotid aneurysms due to growth hormone secretion. ( Fig. 12-13 ).
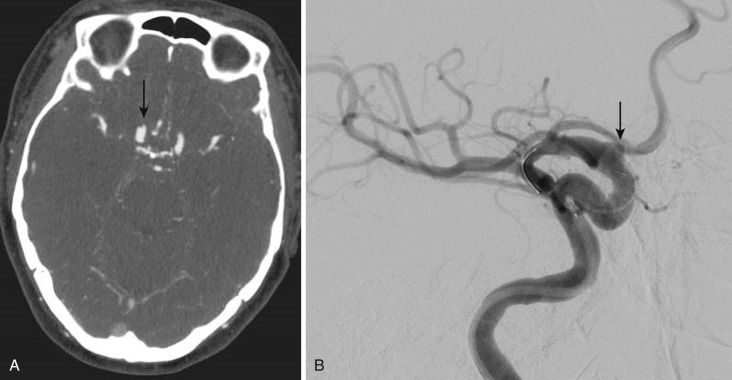
Pathology
The walls of saccular cerebral aneurysms contain intima and adventitia, but the media and internal elastic membrane are thinned or absent, supporting the proposition that degeneration of elastica and muscle cells are the foundations of aneurysm development. Tunica media pathology has been confirmed in cerebral aneurysms found in patients with genetic predispositions. Aneurysm walls may contain variable cellularity of disordered and dedifferentiated smooth muscle cells, fibroblasts, macrophages, leukocytes, mast cells, deficient collagen, and a hyaline-like proteinaceous substance, with the composition progressively abnormal from neck to dome. Alterations in levels or activity of numerous substances including elastin, fibronectin, tumor necrosis factor (TNF)-α, matrix metalloproteinases, oxidative stress reactants, and other proinflammatory isolates have been reported.
Location
Most cerebral aneurysms arise from the circle of Willis and middle cerebral artery bifurcations; 90% involve the anterior circulation and 10% the posterior circulation. The most common sites are the anterior communicating artery at its junction with the adjacent anterior cerebral artery (30%-35%), the posterior communicating artery at its union with the internal carotid artery (30%-35%), the middle cerebral artery bifurcation (20%-35%), the basilar terminus (5%), the carotid terminus (5%), and the origin of the ophthalmic artery (5%). The posterior communicating location is typically categorized with the posterior circulation. Less commonly, aneurysms occur along the course of the anterior cerebral arteries (particularly at the origin of the callosomarginal or pericallosal arteries), middle cerebral artery M1 segment and its branches, posterior cerebral arteries, superior cerebellar arteries, anterior inferior cerebellar arteries, and posterior inferior cerebellar arteries. Cavernous carotid aneurysms may grow to large size, possibly because of rupture prevention by the cavernous sinus dura.
Multiple aneurysms are found in 15% to 30% of patients, more often in women than in men by a 5 : 1 ratio, and most frequently involve the middle cerebral artery. Of the patients with multiple cerebral aneurysms, 75% have two, 15% have three, and 10% have four or more. Bilateral symmetric aneurysms are called mirror aneurysms, and they most often involve the internal carotid arteries or middle cerebral artery bifurcations ( Fig. 12-14 ). When one of multiple aneurysms ruptures and causes subarachnoid hemorrhage, it is most often the largest, although this is not always the case. For instance, Nehls and colleagues have reported the propensity for anterior communicating aneurysms to rupture when several are present simultaneously. Vasculopathies such as fibromuscular dysplasia and connective tissue disorders, as well as syndromes such as polycystic kidney disease, are associated with a higher incidence of multiple cerebral aneurysms. Familial aneurysms tend to be larger, multiple, rupture at a younger age and smaller size, and more frequently occur at the middle cerebral artery bifurcation.
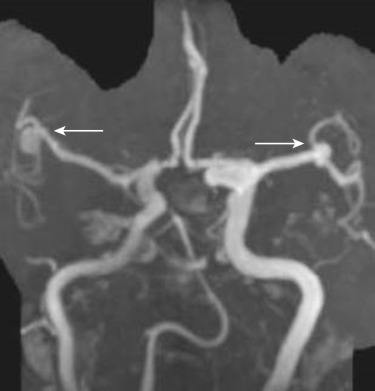
Imaging and Clinical Evaluation
Imaging objectives for patients with a cerebral aneurysm are (1) visualization of subarachnoid hemorrhage if present; (2) confirmation of the size, location, and morphology of the aneurysm; (3) evaluation of the adjacent cerebral vasculature, including evidence of spasm, atherosclerosis, vascular displacement, and incorporation of arterial branches into the aneurysm’s wall; and (4) demonstration of any accompanying adjacent brain pathology.
The typical patient with a ruptured cerebral aneurysm is a woman between age 40 and 60 years who presents with “the worst headache of my life,” neck pain (meningismus), nausea/vomiting, and photophobia, with or without a neurologic deficit. Clinical assessment classifies the patient as Hunt and Hess grades I through V (see Table 12-1 ) or World Federation of Neurological Surgeons grades 1 through 5 (see Table 12-2 ). CT scan of the head identifies subarachnoid hemorrhage, and the patient next typically undergoes CTA, which is used to triage the patient to surgery for clipping or endovascular coiling plus dictating other management. In patients in whom the CT scan shows no hemorrhage, lumbar puncture should typically be performed. The presence of xanthochromia is the sine qua non to confirm subarachnoid hemorrhage; without it, any amount of red blood cells in the cerebrospinal fluid (CSF) may be unreliable because of the possibility that the lumbar puncture itself is the source of the hemorrhage. Xanthochromia may take 12 hours to manifest.
In some situations, MRI and MRA may be performed to evaluate the subarachnoid hemorrhage and to identify a cerebral aneurysm. These examinations can be especially important in the patient with impaired renal function, thereby avoiding iodinated or gadolinium (Gd)-containing intravenous (IV) contrast. MRI without and with contrast may be beneficial to determine if there is an unusual cause for subarachnoid hemorrhage such as hemorrhagic primary or metastatic malignancies. These noninvasive MRI tests may be used routinely when no aneurysm is evident on CTA in the patient with subarachnoid hemorrhage; reserved for cases in which the clinical suspicion for a cerebral aneurysm is low; obtained to better evaluate brain parenchyma status, cranial nerve compression, or hemorrhage age and location; or performed in the patient who is a poor candidate for cerebral angiography or aneurysm intervention (i.e., elderly, extremely poor grade, or poor operative or endovascular candidate because of severe medical comorbidities). MRI and MRA may be contraindicated in patients with implanted ferromagnetic lines or devices such as pacemakers, or in patients who would require sedation because of claustrophobia or uncooperative clinical status when concurrent clinical evaluation is considered paramount. Patient motion may significantly degrade MRI quality.
MRA or CTA may also be employed as screening tests in populations known to have a higher incidence of cerebral aneurysms (see preceding discussion), for monitoring known untreated aneurysms, and to reveal intracranial vascular lesions in patients with headaches. MRA may be preferred as a screening noninvasive imaging study for patients with a low suspicion of a cerebral aneurysm, in young patients, and in patients who require frequent periodic aneurysm evaluation. Better spatial resolution, inherent in CTA, may be reserved for patients with aneurysms less than 3 mm in diameter and when planning intervention at the expense of x-ray radiation.
Computed Tomography
Acute Subarachnoid Hemorrhage.
The most important role for CT in the patient with a cerebral aneurysm is in identification of acute subarachnoid hemorrhage, which is demonstrated as increased density within a cisternal space. The location of the subarachnoid hemorrhage may help determine the location of the underlying cerebral aneurysm ( Fig. 12-15 ). For example, blood in the sylvian fissure most likely has arisen from a middle cerebral aneurysm, and blood in the fourth ventricle is a common sign of rupture of a posterior inferior cerebellar artery aneurysm. Some subarachnoid hemorrhage patterns are not diagnostic of aneurysm location, and none is foolproof.
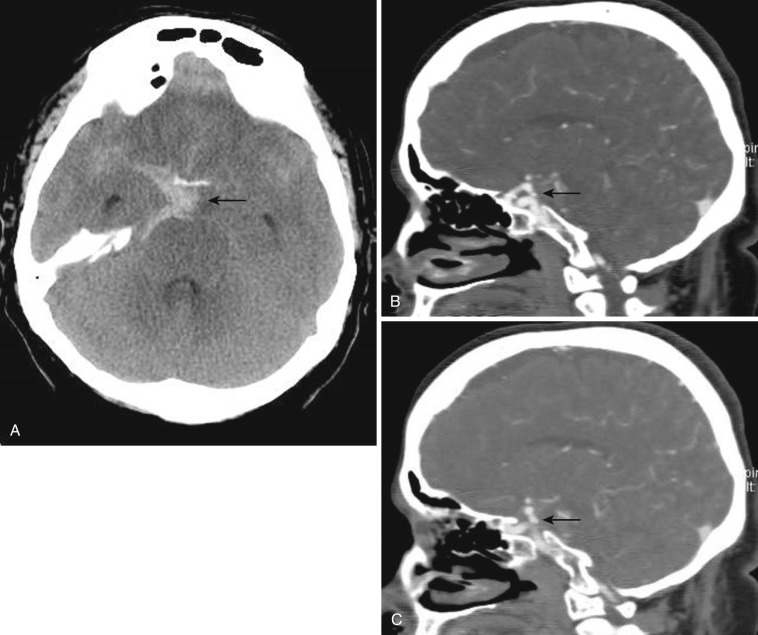
The CT demonstration of subarachnoid hemorrhage progressively decreases until it disappears by approximately 3 weeks, depending on the initial amount of subarachnoid hemorrhage. Subarachnoid hemorrhage is not identified with CT acutely in only a small percentage of cases but may be missed in up to half of patients by 7 days owing to clearance.
A small amount of aneurysmal subarachnoid hemorrhage anterior to the brainstem may mimic perimesencephalic (prepontine and interpeduncular) hemorrhage, a finding believed to be due to the rupture of small perimesencephalic veins or a perforating artery, or secondary to basilar artery dissection ( Fig. 12-16 ). Perimesencephalic hemorrhage accounts for as much as 10% of spontaneous subarachnoid hemorrhage and usually results in a good outcome, unlike an aneurysm rupture. Conversely, the chance of a ruptured aneurysm producing a perimesencephalic pattern of subarachnoid hemorrhage is 2.5% to 8.9%.
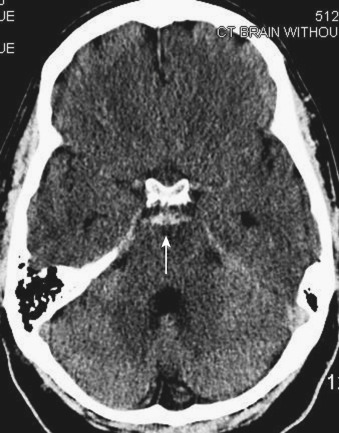
CT is also excellent for identifying hydrocephalus (which develops in 15%-20% of patients after subarachnoid hemorrhage) ( Fig. 12-17 ; see Fig. 12-19 ), intraventricular hemorrhage (13%-28% of cases) ( Fig. 12-18 ), parenchymal hematoma (20%-30%) (see Fig. 12-9 ), and the occasional subdural hematoma. Brain parenchymal hemorrhage develops from rupture of the cerebral aneurysm into adjacent brain substance after the blood passes through overlying arachnoid and pia. Both parenchymal and subarachnoid hemorrhages may obscure an underlying ruptured cerebral aneurysm on CT or MRI.
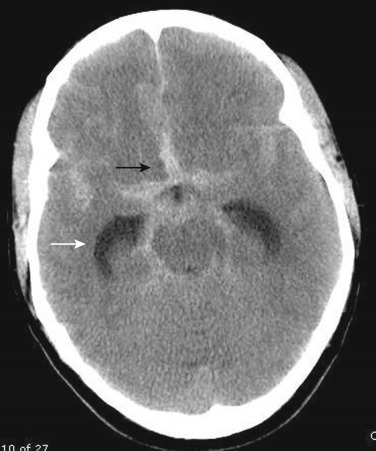
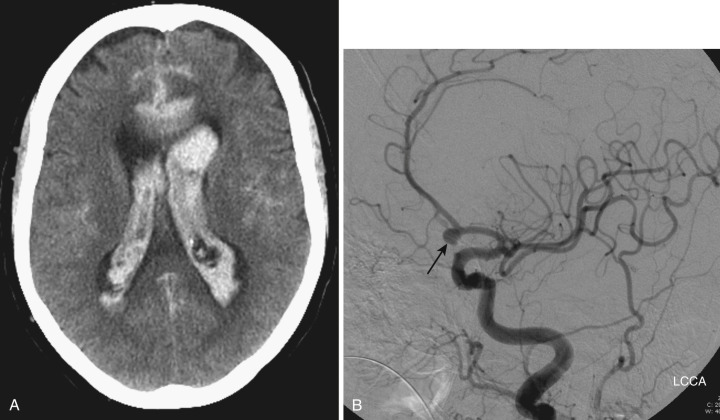
Cerebral Aneurysm.
CT examination is secondarily helpful for identifying cerebral aneurysms typically 5 mm or larger in diameter. The rate of identification of cerebral aneurysms by thin-section, high-resolution, contrast-enhanced CT scanning has been reported to be at least 67% for aneurysms 3 to 5 mm in diameter and to approach 100% for larger aneurysms. Focal, slightly dense areas of luminal blood in typical locations where aneurysms arise ( Fig. 12-19 ), globular or elongated areas of contrast enhancement or a similarly shaped filling defect within a collection of blood ( Fig. 12-20 ), variable calcification involving the aneurysm’s walls, and clot within larger aneurysms characterize cerebral aneurysms. Larger cerebral aneurysms are typically more easily identifiable because of their conspicuous size, but they are also often identified by the presence of internal clot and peripheral wall calcifications, especially in giant aneurysms (>25 mm in diameter) ( Fig. 12-21 ). Giant aneurysms are most commonly identified in middle-aged women; are typically located in the extradural carotid artery, the middle cerebral artery bifurcation, or the basilar summit; and usually manifest with mass effect but can present as subarachnoid hemorrhage. Any large aneurysm can produce mass effect on adjacent brain or cranial nerves (see Fig. 12-19 ). CT is excellent for identifying skull base erosion due to large or giant aneurysms, especially if thin sections (≤3 mm) are obtained.
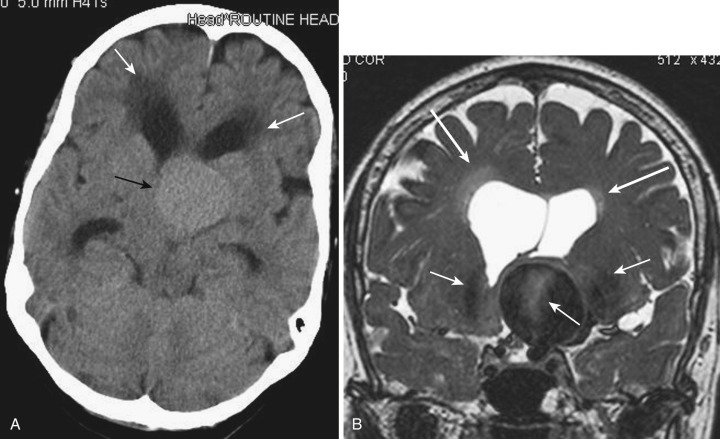
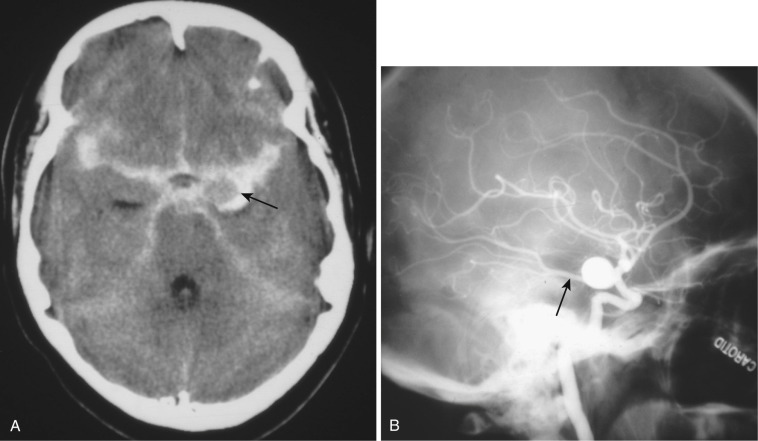
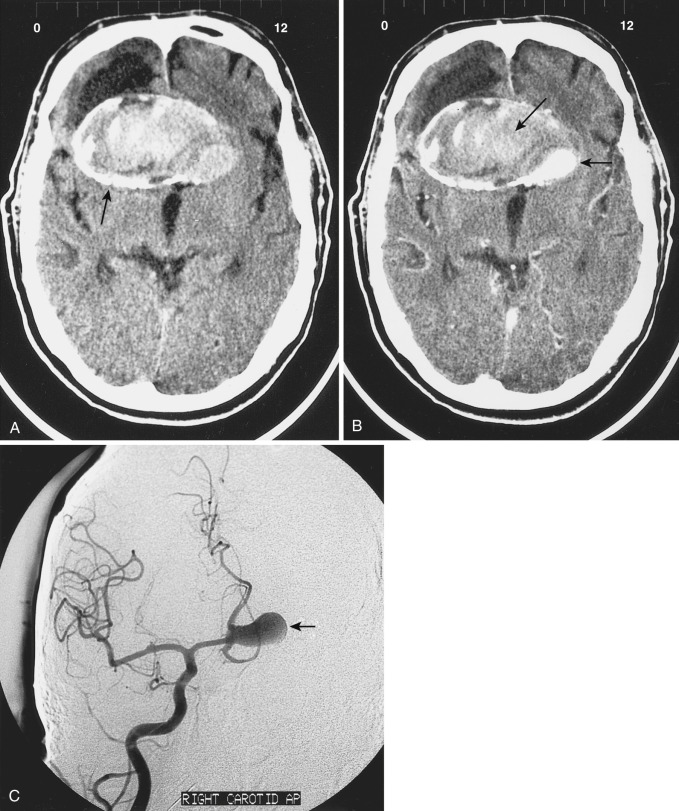
Computed Tomography Angiography
CTA using multirow CT detectors is typically the first study performed to identify a cerebral aneurysm. CTA is excellent for evaluating the morphology, location, and orientation of a cerebral aneurysm, its relationship to adjacent osseous and brain parenchymal structures, and revealing perforating or branch arteries arising next to or from the dome of the aneurysm ( Fig. 12-22 ). After a variable delay based on bolus tracking, thin-section spiral CT slices of 1 mm or less with less than 10% slice gap are acquired during rapid IV infusion of 40 to 70 mL of a high iodine–containing contrast agent for 4 to 5 mL/sec, with kVp (peak kilovoltage) and mAs (milliamperes per second) settings of 80 to 120 and 150 to 400, respectively. Although typical 16-, 32-, or 64-row-detector CT scanners are commonplace, more recent commercial offerings are 256- and 320-row-detector machines. The acquired axial images and the reconstructed two- and three-dimensional (2D and 3D) images created from any of a variety of reconstruction methodologies (e.g., multiplanar reformation, maximum intensity projection [MIP], shaded surface display, ray-sum projection, direct volume rendering) reproduce the cerebral vasculature for scutiny. Both the source images and the reconstructed images should be reviewed because sometimes one will be superior to the other. The exclusion of adjacent skull base bone and the contrast enhancement of nonarterial structures can be problematic ; however, bone removal algorithms and improved contrast bolus timing have been used to mitigate these issues. Electrocardiographic gating of 3D CTA (4D CTA) permits visualization of aneurysm pulsation and calculation of volume change, which may predict unruptured aneurysm growth.
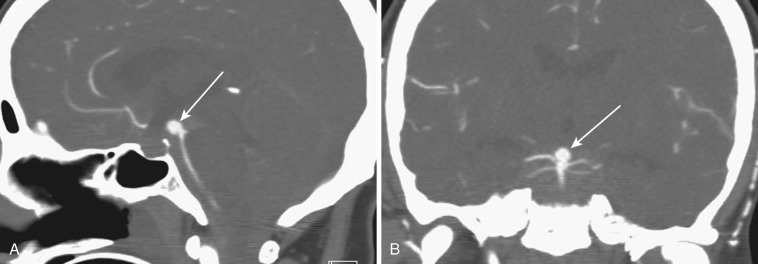
3D CTA has a reported sensitivity of 77% to 100% for identifying cerebral aneurysms. Aneurysms measuring 5 mm or less in diameter may be missed. The sensitivity of CTA for identifying aneurysms 3 to 5 mm or less in diameter is 61% to 93%, significantly better than MRA for this size of aneurysm. CTA has some benefits over MRA, such as for evaluation of calcified atherosclerotic disease of adjacent vessels or in the dome of the aneurysm, although calcification can be suppressed on reconstructed images. CTA source images can be superior to MRA in some instances for visualization of the aneurysm neck or dome and their relationship to osseous skull base structures such as the anterior clinoid process and optic strut, helping to determine whether the aneurysm neck is intradural or within the cavernous sinus.
CTA has been recommended as sufficient for aneurysm treatment planning by several centers. Its advantages include identification of aneurysm dome and parent vessel calcification that influence surgical clipping, rapid acquisition and reconstruction speed, a multitude of processing and reconstruction algorithms including bone subtraction, multiple viewing angles, image manipulations, and relative noninvasiveness. Software reconstruction enhancements for CTA (as well as MRA) can provide endoscopic views of the dome and neck of an aneurysm. Disadvantages include radiation exposure (although efforts have been made to decrease patient dose), difficulty differentiating small aneurysms from infundibula or permitting their identification in regions of complex vascular anatomy (see Fig. 12-4 ), obscuration by adjacent bone, the “kissing vessel” artifact (creating a false-positive aneurysm), variably visualizing fenestrations and small arteries, and potential contrast complications such as allergic reaction and contrast nephropathy. CTA using MIP reconstructions can have difficulty separating the contrast-enhancing patent portion of the aneurysm lumen from intraluminal and perianeurysmal clot, and it can miss some small cerebral aneurysms that are detectable only with catheter cerebral angiography. CTA has been compared to both catheter cerebral angiography and MRA for aneurysm identification and characterization, including mural thrombus detection with mixed conclusions.
It has been suggested that CTA may be sufficient (with a 100% negative predictive value) to exclude a cerebral aneurysm in patients with a CT scan negative for subarachnoid hemorrhage, those with perimesencephalic subarachnoid hemorrhage pattern, or those with CSF xanthochromia or bloody CSF only, especially considering lumbar puncture complications.
Cerebral Vasospasm and Delayed Cerebral Ischemia Due to Subarachnoid Hemorrhage.
Catheter cerebral angiographic vasospasm is identified in as many as 70% of aneurysmal subarachnoid hemorrhage patients. The development of vasospasm is related to the amount of subarachnoid blood, with predictive grades constructed by several authors. Approximately 25% to 45% of patients with aneurysmal subarachnoid hemorrhage develop symptomatic cerebral vasospasm, with as many as 30% developing delayed cerebral ischemia. Approximately 50% of patients with large vessel vasospasm are symptomatic. The risk is greater in younger patients. Roughly 35% to 55% recover and 25% to 30% die.
Transcranial Doppler evaluation has limited sensitivity and specificity for cerebral vasospasm. CTA is beneficial in the noninvasive determination of large proximal-vessel cerebral vasospasm, with reported sensitivity of 77% to 87% and specificity of 93% to 99% compared with catheter cerebral angiography ; as a screening examination it can be used to avoid the more invasive examination.
Although CTA’s sensitivity and specificity for large proximal arterial vasospasm that is clinically symptomatic is over 90% ( Fig. 12-23 ), catheter cerebral angiography remains necessary when CTA is negative, and also for vasospasm treatment with angioplasty or intraarterial infusion of vasodilation agents.
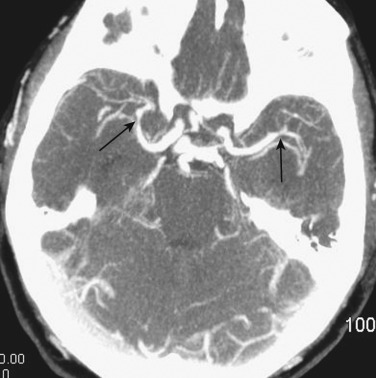
CT perfusion performed by rapid CT imaging during IV bolus contrast administration can determine when cerebral vasospasm compromises tissue perfusion with a sensitivity of 74%, a specificity of 93%, and high positive and negative predictive values. Scan parameters are typically 5 mm thick with minimal slice gap acquired during an IV infusion of a high iodine–containing contrast agent at a rate of 7 mL/sec using an mAs of 100 and kVp of 80. Diminished cerebral blood flow and increased mean transit time or time to peak parameters are particularly helpful and can be predictive of delayed cerebral ischemia, infarction, and permanent neurologic deficits ( Fig. 12-24 ). CT perfusion is superior to transcranial Doppler to predict the need for endovascular treatment of cerebral vasospasm, and its combination with CTA can accurately diagnose cerebral vasospasm. Neither severe vasospasm nor decreased CT perfusion always lead to delayed cerebral ischemia, and patients without spastic cerebral arteries or abnormal cerebral perfusion can go on to infarction.
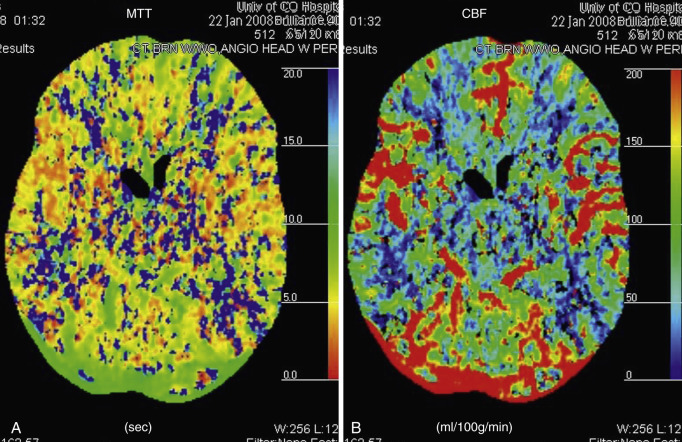
MRA does not appear to be reliable for imaging cerebral vasospasm.
Magnetic Resonance Imaging
Acute Subarachnoid Hemorrhage.
The identification of acute subarachnoid hemorrhage by routine MRI has been notoriously poor, although there are reports that claim otherwise. The following two reasons have been postulated for the lack of visualization of acute subarachnoid hemorrhage on MRI :
- 1.
Normal P o 2 (partial pressure of oxygen) in CSF inhibits the development of deoxyhemoglobin.
- 2.
Lysis of red blood cells in subarachnoid CSF prevents heterogeneous magnetic susceptibility.
However, with the employment of pulse sequences including intermediate-weighted spin echo or fast-spin echo with TE (echo time) values of 22 to 45 milliseconds, T1-weighted spin echo, FLAIR (fluid-attenuated inversion recovery) imaging, gradient echo imaging, and susceptibility-weighted imaging (SWI), acute subarachnoid hemorrhage can frequently be identified ( Figs. 12-25 and 12-26 ). Other imaging problems can obscure precise identification of subarachnoid hemorrhage on MRI, including CSF and vascular pulsation artifact and magnetic susceptibility artifact along the skull base. With experience, acute subarachnoid hemorrhage often can be correctly identified on MRI. Subarachnoid hemorrhage older than 12 to 24 hours can routinely be identified on MRIs, often helping pinpoint the underlying ruptured cerebral aneurysm by a collection of blood.
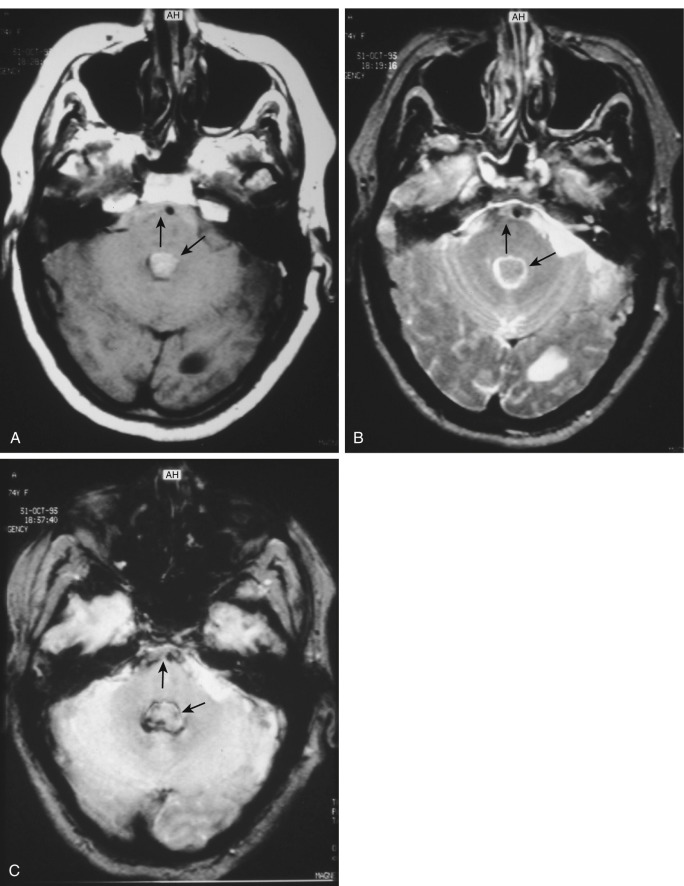
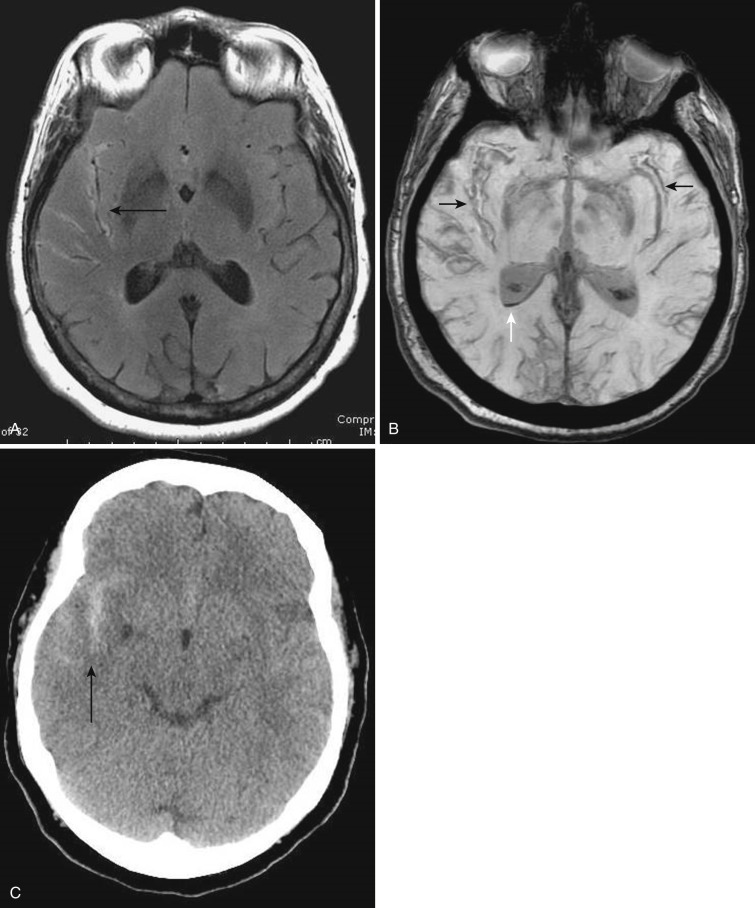
Cerebral Aneurysm.
MRI is superior to CT for the localization of cerebral aneurysms, their relationship with adjacent structures, and associated changes in neighboring brain tissue (see Fig. 12-19 ).
Thin-section MRI examination can often identify cerebral aneurysms over 3 mm in diameter ; identification may be easier if the aneurysm is unruptured. It may be difficult, however, to differentiate normal vascular tortuous anatomy or bone such as the anterior clinoid process from a cerebral aneurysm. When large enough, cerebral aneurysms can be positively identified on MRI because of flow artifacts or characteristic findings with the use of different pulse sequences. Phase-encoding artifact due to flow-producing signal mismapped in the phase-encoding direction can be visualized on a variety of pulse sequences ( Fig. 12-27 ; see Fig. 12-19 ). Other flow effects are also often helpful to identify cerebral aneurysms. These include a swirling appearance inside the aneurysm due to spin dephasing, rephasing, and turbulence; even-echo or gradient rephasing; diastolic pseudogating; slow flow; variable intraluminal flow rates; entry slice enhancement; and signal void due to rapidly flowing blood visualized on most pulse sequences ( Figs. 12-28 and 12-29 ).
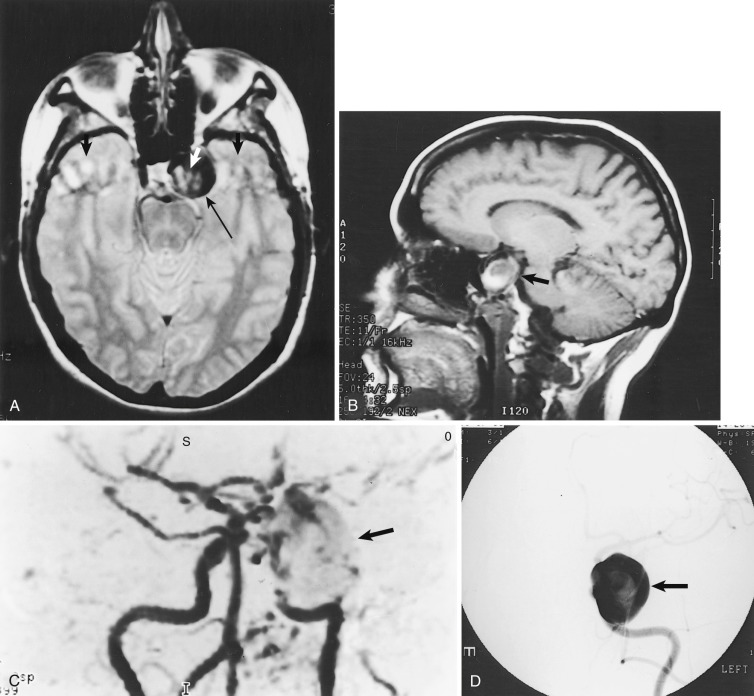
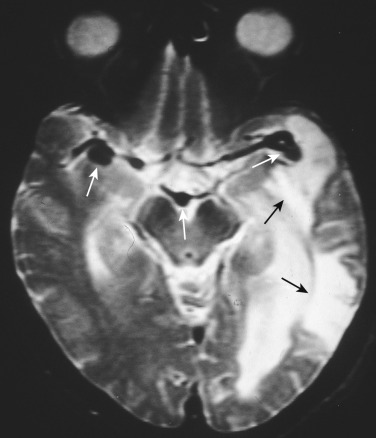
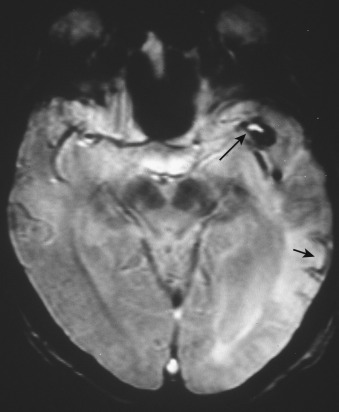
Contrast enhancement of the aneurysm highlights its central lumen and can exaggerate the aforementioned artifacts, particularly the phase mismapping effects (see Fig. 12-77 ). For instance, these clues can often reliably diagnose cavernous-carotid segment aneurysms and differentiate them from pituitary masses.
A partially thrombosed aneurysm contains peripheral layers of clot of different ages with an onion-like appearance, usually with the oldest clot located most peripherally. MRI may identify intramural thrombus in dissecting pseudoaneurysms and fusiform aneurysms ( Figs. 12-30 and 12-31 ). The signal intensities of intraluminal clot correspond to the expected age of hemorrhage on MRI according to the magnetic field strength employed. Fibrosis and cellular infiltration can accompany the clot, typically reducing signal intensity, and perianeurysmal edema may be present to a variable extent. Completely thrombosed aneurysms or aneurysms with extremely slow flow can rarely mimic tumors, including sellar and parasellar masses (see Fig. 12-27 ). Catheter cerebral angiography may be necessary in these problematic cases to prevent “surprises” during craniotomy and potentially catastrophic hemorrhage during transsphenoidal or other operations.
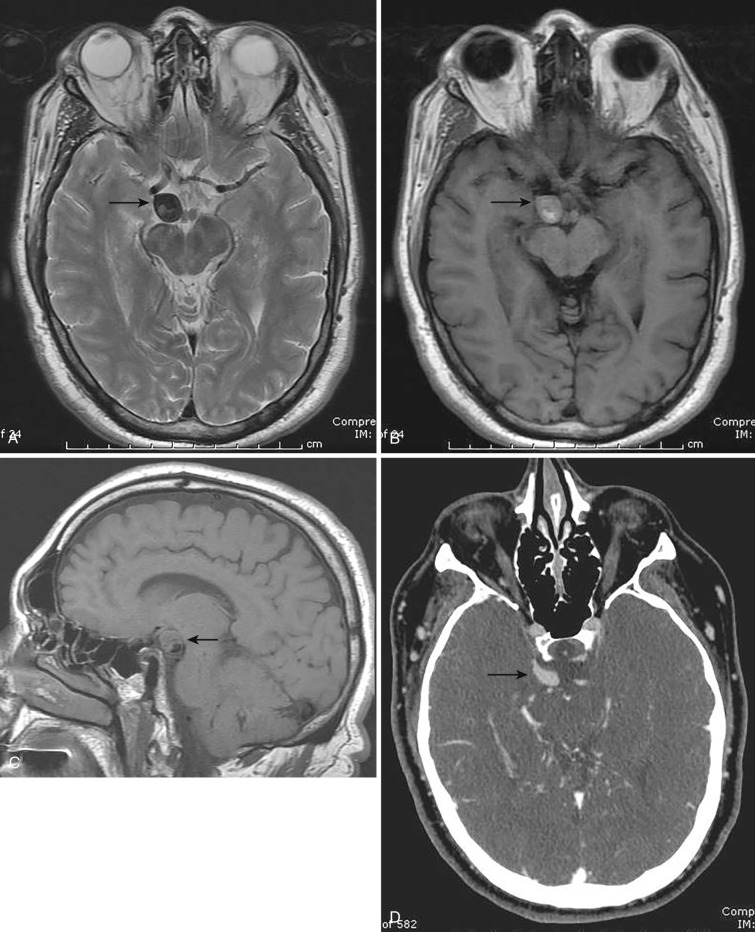
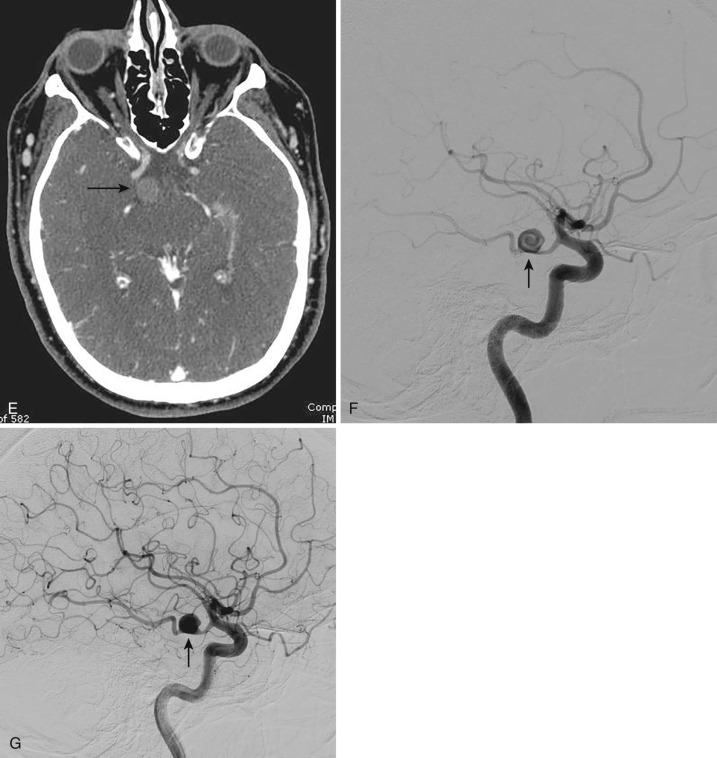
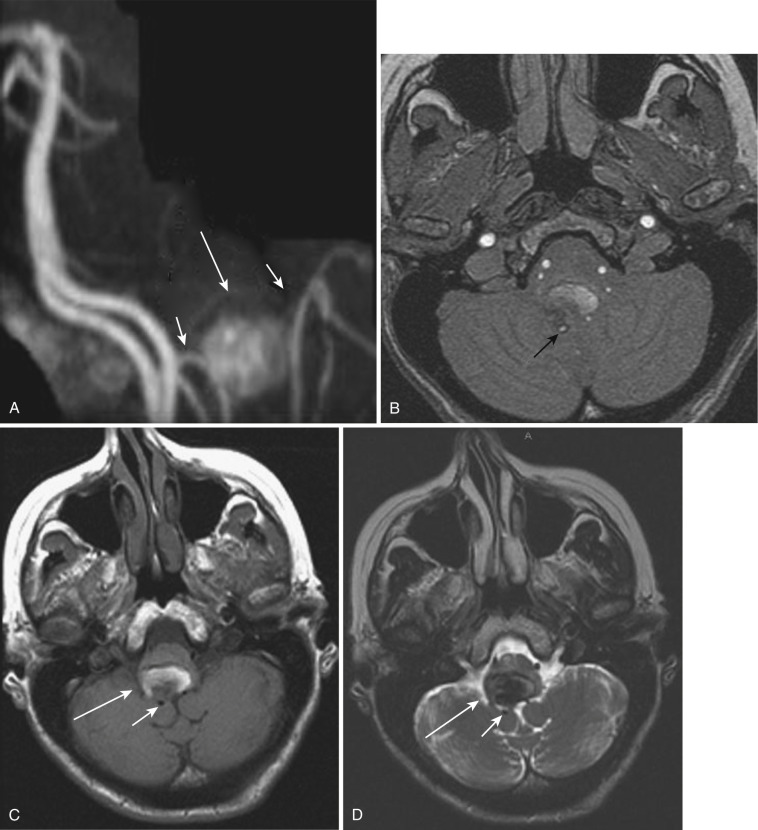
