Laura Evangelista, Paolo Artoli, Paola Bartoletti, Antonio Bignotto, Federica Menegatti, Marco Frigo, Stefania Antonia Sperti, Laura Vendramin, and Diego Cecchin Nuclear Medicine Unit, Department of Medicine, University of Padua, Padua, Italy Positron emission tomography/magnetic resonance imaging (PET/MRI) represents a complex imaging modality able to combine the anatomical and functional information provided by MRI and the functional/molecular data provided by PET. However, its interpretation requires coordination of many professional figures, from technologists to physicians (both radiologists and nuclear medicine physicians). Furthermore, specific knowledge about the clinical information obtained from PET and MRI is important in order to select appropriate patients who may benefit from this hybrid imaging modality. Anamnesis and clinical data should be always accurately taken before the examination because a lot of patients can suffer from claustrophobia. Moreover, some conditions are not compatible with MRI scanners, such as the presence of metal or a pacemaker. Whole‐body PET/MRI is usually performed in all patients with oncological requests. It is usually performed using the most common MRI sequences (T1‐weighted and T2‐weighted sequences), with or without the adjunction of contrast‐enhancement (such as gadolinium‐based agents). Moreover, additional sequences can be added, such as diffusion weighted imaging (DWI), for the assessment of the apparent diffusion coefficient (ADC). Often, additional segmental images are made in the case of specific clinical requests, for example for liver metastases, head and neck cancer, and similar. Nononcological disease, such as cardiac sarcoidosis and brain dementia, requires dedicated protocols for the analysis of the movements or for specific anatomical assessments, respectively. Finally, for the correction of PET images, the Dixon or Caipirinha sequences are used. In the following sections we address the use of PET/MRI, mainly with 18F‐fluorodeoxyglucose (18F‐FDG), in oncological and nononcological conditions based on the daily clinical experiences we have amassed. Gliomas are the most common primary brain tumors in children and adults, consisting of a heterogeneous group of neoplastic diseases arising from the supporting cells of the central nervous system (CNS). Computed tomography (CT) is frequently obtained prior to MRI for the initial assessment of a suspected intracranial mass lesion. The limitations of CT compared to MRI include inferior soft tissue characterization, posterior fossa beam hardening artifact, and the use of ionizing radiation. Conventional gadolinium‐enhanced MRI is the modality of choice for the evaluation and management of intracranial masses. It is pivotal for the differential diagnosis of brain tumors as it provides information about both their size and location, and also gives an estimate of blood–brain barrier breakdown by the degree of gadolinium contrast enhancement. However, it has some limitations in differentiating high‐ from low‐grade tumors [1] and in distinguishing disease recurrence from post‐therapy changes [2]. Moreover, radiological assessment of the true extent of glioma by conventional MRI is unsatisfactory, as enhancing MR abnormalities can reflect either oedema or tumor infiltration, therefore brain tumor tissue may extend beyond areas with gadolinium enhancement [3]. Advanced MRI modalities have been proposed to determine all information that cannot be obtained through standard anatomic MRI. The most widely studied and most commonly employed of these modalities are spectroscopy [4], perfusion, and DWI MRI. This latter sequence is sensitive to the motion of water molecules in three dimensions within tissue and diffusion tensor imaging acquires water diffusion information using a minimum of six diffusion‐encoded directions. Diffusion tensor imaging (DTI) has been shown to differentiate between low‐ and high‐grade glioma, and to distinguish glioblastoma from metastases. It also delineates the margins of primary brain tumors better than conventional MRI alone [5, 6]. Functional MRI (fMRI) is primarily used for presurgical evaluation to localize regions of motor activation and language activation that lie nearby or within a lesion [7]. The information provided by fMRI is often coupled with diffusion tractography to optimize presurgical planning with the goal of maximizing the extent and safety of tumor resection while minimizing postoperative neurological deficits [8]. Hybrid PET/MRI combines, in a single scan, the high spatial resolution and soft tissue characterization of gadolinium‐enhanced and advanced MRI with metabolic information from PET. The acquisition is performed with simultaneous PET and MRI scanning in the magnetic resonance head coil. The first step is a localization scan followed by a T1‐weighted sequence of volumetric interpolated breath‐hold examination (VIBE) two‐point‐Dixon gradient echo, used to create a tissue model for attenuation correction and to reconstruct the attenuation‐corrected PET images. Standard anatomic imaging of the brain typically includes two‐dimensional precontrast T1‐ and T2‐weighted spin‐echo, fluid‐attenuated inversion recovery (FLAIR), and postcontrast T1‐weighted spin‐echo sequences in at least two orthogonal planes. Many protocols also include advanced MRI modalities, such as DWI or DTI. Different radiotracers are available for PET brain imaging, and some papers have reported the advantages of PET/MRI with them [9, 10]. 18F‐FDG is the most commonly used radiopharmaceutical for its high specificity in differentiating the presence of high‐grade tumors, characterized by pathologically increased accumulation of tracer above the level of gray matter and for the possibility of distinguishing postradiation necrosis from local recurrence, often characterized by accumulation at the same level of the surrounding unaffected white matter [11]. Figure 29.1 shows a patient with a brain tumor detected by 18F‐FDG PET/MRI. The major advantage of amino acid analog PET tracers is their relatively high uptake in tumor tissue and comparatively low uptake in the normal brain, with significant tumor‐to‐background contrast. The uptake of these radiolabeled amino acids is based on the expression of L‐amino acid transporter (LAT)‐1 and LAT2 on the surface of tumor cells with nondependence on blood–brain barrier permeability; for this reason, amino acid probes are able to depict noncontrast‐enhancing brain tumor regions with a clear advantage over other PET tracers [12]. 11C‐methionine (MET) is the most widely studied tracer in this group, but its use is restricted to centers with an on‐site cyclotron by the short half‐life of 11C (20 minutes) compared to 18F (110 minutes) [13]. For this reason, 18F‐labeled tracers have been developed, including 18F‐fluoroethyl‐L‐tyrosine (FET) and the amino acid analog tracer 3,4‐dihydroxy‐6‐18F‐fluoro‐ L‐phenylalanine (FDOPA). FET PET has been studied for delineating the extent of gliomas in order to guide tissue sampling and treatment planning, for detection of tumor recurrence, and for prognostication in low‐grade glioma. In a recent study including a small prospective cohort of high‐grade glioma recurrences treated with bevacizumab and irinotecan, both standard and kinetic FET PET imaging were demonstrated to be superior to conventional MRI, using response assessment in neuro‐oncology (RANO) criteria, for prediction of early treatment failure [14]. Figure 29.2 shows an example of PET/MRI with FET in a recurrent glioma. FDOPA PET has been shown to be more accurate than 18F‐FDG PET for evaluation of low‐grade tumors and for distinguishing tumor recurrence from radiation necrosis [12]. However, the use of FDOPA imaging of gliomas is rare because it is associated with a high degree of accumulation in the dopaminergic system in the brain/basal ganglia and also in the putamen or substantia nigra, which might interfere with tumor delineation [10]. 18F‐fluoromisonidazole (FMISO) represents a PET imaging agent for identification of hypoxia and has an improved ability to distinguish glioblastoma (GBM) from lower grades as compared with 18F‐FDG. Similarly, 18F‐fluoroazomycin arabinoside (FAZA) seems to detect the presence of a hypoxic region within the tumors, thus guiding a personalized radiotherapy planning. Head and neck cancer ranks within the 10 most common cancers in the human body [15, 16]. The head and neck region is an anatomically complex site that performs various physiological functions and constitutes the facial morphology. Therefore, for patients with head and neck cancer, accurate localization of primary and recurrent tumor is an essential for planning optimal treatment to preserve its function and morphology as much as possible. PET MRI offers many advantages in the characterization and prognostication of head and neck malignancies thanks to the sensitivity and the negative predictive value of PET combined with the high soft tissue contrast of MRI. Figure 29.1 18F‐FDG‐PET/MRI of an intracranial mass in the left parietal lobe. The PET scan shows a high 18F‐FDG uptake in the left parietal lobe. The postcontrast T1‐weighted MRI shows an inhomogeneous postgadolinium enhancement in the same region, with a low signal for DWI images. In the initial staging of primary tumors, 18F‐FDG PET/MRI performed better than PET/CT. In particular, the superiority of T2‐weighted and nonenhanced T1‐weighted sequences over PET/CT in local staging [17] and the advantage of contrast‐enhanced T1‐weighted sequences in evaluating perineural tumor spread that is a negative prognostic risk factor have been demonstrated. However, the assessment of primitive tumors can be affected by imaging artifacts, depending on location: tumors arising in the oral cavity and oropharynx are more affected by dental artifacts (beam hardening), while tumors arising in the hypopharynx and larynx are more affected by motion artifacts from swallowing. The first artifacts can significantly compromise the interpretation of PET/CT and the second one can compromise the interpretation of PET/MRI. The appropriate hybrid imaging should therefore be assessed on the basis of clinical information. In the assessment of lymph node involvement, a slight advantage of T2‐weighted imaging over T1 has been reported, although many studies have confirmed an equal performance of PET/MRI in comparison to PET/CT [16–19]. The main challenge for PET/MRI is the evaluation of lung metastasis. Due to breathing and the duration of MRI sequences, motion can affect image quality. However, this limitation can be overcome by using a gated lung technique and/or a dedicated MR pulse sequence for the lungs that allows the detection of nodules only 3 mm in size. Nevertheless, there is evidence that PET/MRI might surpass PET/CT in the detection of concurrent occult primaries, particularly in other head and neck regions [20, 21]. The main disadvantages of PET/MRI in head and neck tumors, but also in other malignancies, are the longer acquisition time and the higher costs compared to CT. However, a full diagnostic PET/MRI scan in the head and neck can be obtained in 30–40 minutes. The acquisition protocol should be optimized using T1‐weighted axial, T1 fat saturation axial plus coronal or sagittal, and T2 fat sat axial sequences to evaluate the neck region, and evaluating the whole body using a T1 DIXON axial and a T2 fat sat coronal to assess the whole body. Whenever possible, the scan should be gadolinium contrast‐enhanced to obtain the highest diagnostic accuracy. DWI and perfusion‐weighted imaging should be performed for the evaluation of the response to therapy and for the planning of radiation treatment. Figure 29.3 shows PET/MRI of a primitive head and neck tumor detected by 18F‐FDG, postcontrast T1, and DWI‐MRI sequences. Figure 29.2 18F FET‐PET/MRI of GBM in the right temporal lobe. The PET scan shows a high FET uptake in the antero‐lateral and medial side of the surgical cord in the right temporal lobe (TBRmax mean 3.8). The postcontrast T1‐weighted MRI shows a lesion with an enhancement, associated with an alteration of the fluid‐attenuated inversion recovery (FLAIR) signal in the T2 sequences. Hepatocellular carcinoma (HCC) represents over a third of liver cancer. In recent years, 5‐year survival rates have been considerably improved due to earlier detection and curative therapies [22]. Many studies have demonstrated that MRI has excellent sensitivity and specificity for the detection and characterization of HCC compared with CT and ultrasound [23–26]. The role of 18F‐FDG PET in HCC diagnosis is not well established since the metabolism of HCC cells is highly variable. Low expression of glucose transport (GLUT)1 and GLUT2, high expression of P‐glycoprotein, and high activity of 18F‐FDG‐6‐phosphatase were demonstrated in moderately and well‐differentiated HCCs [27]. However, many authors have reported on the high sensitivity of 18F‐FDG PET in the detection of extrahepatic HCC metastases [28–32]. Moreover, 18F‐FDG PET showed strong prognostic value in HCC patients, since less differentiated and more aggressive HCCs usually have higher rates of glucose consumption [33, 34]. The combination of PET with MRI in patients with HCC seems very promising for many reasons: (i) detection of the primary tumor, thanks to the additional information provided by PET and MRI, (ii) assessment of local therapies (such as transarterial chemoembolization), and (iii) target lesion dosimetry in patients who are candidates for 90Y radioembolization. However, to date few studies using PET/MRI in patients with HCC have been available [35–38]. Figure 29.4 shows the presence of multiple areas of 18F‐FDG uptake and specific MRI signs in a patient with a multifocal HCC. Figure 29.3 18F‐FDG PET showing two focal areas of uptake in the anterior left margin of the tongue and in a small left satellite lymph node. A postcontrast T1‐VIBE‐MRI sequence shows increased enhancement in the same regions. With DWI a high signal was visible, mainly in the left lymph node, while a small diffusion signal was demonstrated in the tongue. Pancreatic ductal adenocarcinoma (PDA) accounts for 80% of pancreatic malignancies. A complete resection is the only cure, but is limited to 15–30% of patients who received an early diagnosis in a late onset of the disease [39]. Enhanced CT is the most used imaging modality for diagnosis and initial staging of PDA, but several studies have shown that MRI is superior in detecting smaller lesions. MRI using T1‐ and T2‐weighted images, DWI, ADC, and a hepato‐specific intravenous contrast is useful for the evaluation of morphologic features of primary and metastatic liver cancer. 18F‐FDG PET/CT in preoperatory staging of PDA is useful in detecting metastatic disease, with some studies showing a change in management in up to 11% of patients, avoiding unnecessary surgery owing to the detection of occult metastases [40]. Moreover, high standardized uptake values (SUVs) on pretreatment 18F‐FDG PET/CT scans correlated with a worse prognosis and a decrease in progression‐free survival [41]. After neoadjuvant therapy, anatomic imaging showed limited sensitivity for the evaluation of treatment response due to postradiation changes. PET has been found to be very helpful in this setting because a decrease in 18F‐FDG uptake may precede morphologic changes and therefore can better estimate the response to treatment. Similarly, also in the postoperative setting, the presence of surgical scarring limits the identification of PDA recurrence. Integrated PET/MRI can potentially be superior to each modality alone, combining high soft tissue contrast for MRI and metabolic information from PET. For example, 18F‐FDG PET/MRI had sensitivity equal to 93% versus 91% for MRI with hepato‐specific contrast alone for the identification of liver metastases [42]. Therefore, in the initial staging of disease, 18F‐FDG PET/MR can be used, although the small amount of evidence available and the limited availability of PET/MR scanners represent important limitations in clinical practice. Figure 29.5 shows an example of patient with pancreatic cancer staged with 18F‐FDG PET/MRI. Intraductal papillary mucinous neoplasms (IPMNs) are considered premalignant lesions, characterized by the papillary growth of ductal epithelium with mucin production. They are classified into main duct type (main pancreatic duct dilatation >5 mm), associated with 60% risk for malignant transformation, branch duct type (cystic lesion in continuity with the main pancreatic duct), associated with 25% risk for malignant transformation, and mixed IPMN. Based on the degree of dysplasia of ductal epithelium, they are classified from mild, intermediate, and high‐grade dysplasia to invasive carcinoma [43]. MRI/MR cholangiopancreatography and endoscopic ultrasound imaging showed a similar diagnostic accuracy for the diagnosis of IPMN. 18F‐FDG PET/CT has demonstrated that an increased 18F‐FDG uptake is highly specific in differentiating between benign and invasive IPMNs with a suggested SUVmax cutoff value of 2.3 [44–47]. Pedrazzoli et al., in a trial published in 2011, showed a higher accuracy of 18F‐FDG PET/CT in differentiating malignant from benign IPMN compared with 2006 international consensus guidelines criteria (sensitivity 83% vs. 93%, specificity 100% vs. 22%, accuracy 91% vs. 61) [46]. It is important to mention that 18‐FDG PET can be liable to false‐positive results due to inflammatory disease, as in pancreatitis. The timing of PET in patients under surveillance remains to be established, but it might reasonably be performed when lesions reveal morphological changes or patients become symptomatic. PET/MRI scanners have a possible role in the management of IPMN, but data in the literature are limited [48]. Figure 29.4 Top: The first three images on the left show multiple 18F‐FDG uptake in the right liver lobe. T2, T1, and DWI MRI images show a inhomogeneous signal at the level of the liver segments (S8, 11 mm in diameter; S7, 20 mm; S4b, 6 mm; S5–S8, 7 mm). Figure 29.5 80‐year‐old woman with a diagnosis of pancreatic ductal adenocarcinoma. Top, 18F‐FDG PET/MR shows a solid nodule with a high 18F‐FDG uptake in the pancreatic head. In the same region, hysointensity in T1‐weighted and hypointensity in T2‐weighted images was found. Bottom, PET/MRI images in the liver show the presence of a hyperintense lesion in T2 and a hypointense lesion in T1 of 13 mm in diameter in S3 with a moderate 18F‐FDG uptake, compatible with a distant metastasis. Pancreatic neuroendocrine tumors (PNETs) origin from endocrine cells and 80% of them express somatostatin receptors (SSRT) type 1–5. The expression of SSRT allows targeting with synthetic somatostatin for diagnostic and therapeutic purposes. Usually, PNETs are combined with small bowel neuroendocrine tumors and classified as gastroenteropancreatic NET (GEPNETs). The classification is based on mitotic count and Ki‐67 proliferation index: G1 (Ki‐67 < 3%), G2 (Ki‐67 3–20%), and G3 (Ki‐67 > 20%) [49]. Enhanced CT is usually the first study performed, showing a sensitivity of 70%. MRI with intravenous contrast triple phase (also with delayed postcontrast), DWI, and ADC sequences is useful to identify hepatic metastases. Recently, 68Ga‐labeled SSTR agonist (DOTA‐TOC, DOTA‐NOC, and DOTA‐TATE) PET has been demonstrated to be useful for staging, restaging, and selecting candidates for therapy with radiolabeled somatostatin analogues. A recent meta‐analysis of 22 studies showed a sensitivity of 97% and a specificity of 95% [50]. The largest study evaluating how 68Ga DOTA‐TATE PET/CT impacts on the therapeutic decision of this tumor type showed that it changed management in 40.9% of patients [51]. PET/MRI seems a promising tool for evaluation of GEPNETs, combining the accuracy of SSTR PET and the soft tissue contrast of MRI, therefore it can be considered definitely better in finding liver metastases, but has a lower detection rate for lung lesions. Again, evidence about this topic is scarce in the literature [52]. Prostate cancer (PCa) is the second most common cancer in men worldwide according to data from the WHO GLOBOCAN database. Acinar adenocarcinoma represents 90–95% of prostate cancers diagnosticated, usually in patients who are asymptomatic, based on abnormalities in a screening prostate‐specific antigen (PSA). MRI is considered the main imaging modality for the detection of the extent of primary tumor and local recurrence in PCa patients, but the clinical availability of integrated PET/MRI scanners has changed the diagnostic approach. The integration of anatomical and functional imaging by using different radiopharmaceuticals, such as radiolabeled choline or prostate‐specific antigen membrane (PSMA) can change the staging and restaging of these patients. Indeed, PET imaging has significantly impacted on different clinical settings, from initial staging to biochemical recurrence (BCR), and has also guided personalized treatment planning [53]. For initial staging, it has been reported that PET/MRI alters therapeutic strategies in at least 30% of patients [54]. This latter advantage is mainly due to higher diagnostic value of PET/MRI compared with multiparametric MRI (MRI) [55, 56] or PET imaging alone [57] for the detection of PCa. PET/MRI is more sensitive than mpMRI in identifying primary tumors in the peripheral zone of the prostate gland, and in revealing extracapsular extension and seminal vesicle infiltration. Moreover, especially if PSMA is used, PET/MRI shows high lesion contrast and an excellent consistency in lesion detection [58] because an intense uptake on PET combined to mpMRI anatomical changes strongly correlates with the dominant lesion in the prostate glands of men undergoing imaging before surgery [59]. Some authors documented that 68Ga‐PSMA‐11 PET/MRI provides valuable diagnostic information and improves patient selection for extended pelvic lymph node dissection by comparison with the currently used clinical nomograms [60–62]. It has also been reported that PET and PET/MRI have a considerably lower proportion of equivocal results in distinguishing aggressive from indolent disease and in the detection of extracapsular extension compared to mpMRI alone [63]. Also, in restaging settings PET/MRI proved to have a high detection rate for recurrent disease, particularly when radiolabeled PSMA was used. Moreover, it is able to identify oligometastatic disease and guide to an appropriate radiation treatment (extension of the radiation field, extension of lymph node adenectomy, etc.) allowing up to 75% of change in the patients’ management. PET/MRI in cases of biochemical disease recurrence has been demonstrated to achieve a detection rate of up to 97% [64] and is more accurate than PET/CT in detecting local recurrences. This improves the detection rate for lower PSA levels, thanks to the MRI component, which is crucial in identifying local recurrences otherwise masked by the accumulation of radiopharmaceuticals in the bladder. Figure 29.6 shows an example of a patient with recurrent PCa detected by 18F‐choline PET/MRI. The choice of MRI sequences has an important influence on the detection of local and distant metastases: metastases from prostate cancer are best detected by DWI or gadolinium‐enhanced T1‐weighted VIBE sequences and the most time‐efficient sequence with the highest lesion detection rate is gadolinium‐enhanced T1‐weighted VIBE. The detection of bone metastasis is increased by using multiple MR sequences, especially in the case of bone marrow involvement. Figure 29.6 A 62‐year‐old male with prostate cancer (histopathological characteristics: Gleason score = 6, pT3apNx, and positive margins). Three years after the radical prostatectomy, a biochemical recurrence occurred (PSA value increased from 0.01 to 5.2 ng/ml). T2_tse sequence shows a 5 × 2 mm area of recurrence in prostatic fossa with a moderate 18F‐choline uptake. Rectal cancer accounts for about one in three cases of colorectal cancer tumor, representing the most common neoplasm of the gastrointestinal tract. Current guidelines recommend MRI for local staging and contrast‐enhanced thoraco‐abdominal CT for detecting distant metastases [65]. PET/CT with 18F‐FDG is recommended in patients with a locally advanced or recurrent rectal cancer as this can identify the presence of distant metastases [66]. However, PET/MRI has shown some advantages in rectal cancer, mainly in its ability to assess the soft tissue and the relationship between the tumor and the surrounding tissue. In the definition of a primary tumor, PET/MRI can reveal mesorectal fascia involvement and also distinguish between recurrence and fibrosis post‐radiotherapy better than PET/CT [67, 68]. Figure 29.7 shows an example of a patient with rectal cancer detected by 18F‐FDG PET/MRI. For the definition of lymph node involvement, PET/MRI is able to identify the presence of small pathological lymph nodes more accurately than MRI alone thanks to the metabolic information provided by PET [68]. Lee et al. [69] found that PET/MRI significantly improves the detection of distant metastases in rectal cancer, mainly liver metastases [70]. However, it has limited use in the detection of lung lesions because of the low‐quality sequences in this region. Moreover, PET/MRI can help in the identification of response to local therapies in the liver metastases. Multiple myeloma (MM) is a neoplastic disease characterized by the presence of 10% or more of clonal bone marrow plasma cells or the presence of extramedullary sites. Moreover, at least one of the following calcium, renal, anemia and bone (CRAB) criteria must be present: (i) elevated serum calcium level (1 mg/dL above normal or over 11; C), (ii) signs of renal failure (R), with serum creatinine above 2 mg/dL or renal clearance less than 40 mL/min, (iii) hemoglobin level less than 100 g/L or greater than 20 g/L below the lower limit of normal (anemia, A), and (iv) one or more osteolytic lesions at CT, RX or PET/CT (bone, B) [71]. There are different forms of MM, such as monoclonal gammopathy of undetermined significance (MGUS), smoldering myeloma, solitary plasmacytoma of bone or extramedullary, and Waldenstrom macroglobulinemia. The definition of disease depends on serum and urinary monoclonal protein levels, clonal bone marrow plasma cells level, and the number of osteolytic lesions. In recent decades CT, 18F‐FDG‐PET/CT, and MRI have increased their value in the management of MM patients regarding diagnosis, follow‐up, planning of therapy, and response to treatment. Figure 29.7 Sagittal and coronal 18F‐FDG PET showing linear and intense uptake in the rectal wall. The core‐gistrated PET/MRI trans‐axial image shows intense 18F‐FDG uptake in the right lateral wall of the rectum. In the same area, DWI‐MRI (below, first image on the right) and post‐contrast MRI show an increased signal and increased enhancement, respectively, compatible with an invasive rectal cancer. The International Myeloma Working Group (IMWG) recommend MRI as the gold standard in the diagnosis of MM since it allows (i) the evaluation of the bone marrow involvement that is usually defined in terms of normal, focal, diffuse, and variegated/salt‐and‐pepper pattern, (ii) the identification of painful lesions for spinal cord compression, and (iii) for the differentiation between benign and malignant vertebral fractures [72]. In general, a positive MRI for MM shows the presence of lesions with a loss of signal on T1‐weighted images, an increased signal on T2‐weighted images, and a delayed contrast enhancement [73]. 18F‐FDG PET allows both marrow involvement (sensitivity 59–85%, specificity 79–92%) and extraosseous disease to be identified; moreover, it can be useful in the detection of occult lesions [74]. MRI, as well as PET/CT, can be helpful in differentiating active myeloma from smoldering myeloma. According to the National Comprehensive Cancer Network (NCCN) guidelines, an active MM is diagnosed when there is at least one focal lesion on the MRI greater than 5 mm or one focal hypermetabolic osteolytic lesion on PET/CT along with clonal cells of at least 10% in the bone marrow. Shortt et al. [75]
29
Clinical Application of PET/MRI
PET/MRI General Introduction
Application of PET/MRI in the Oncological Setting
PET/MRI in Brain Tumors
PET/MRI in Head and Neck Cancer
PET/MRI in Hepatocellular Carcinoma
PET/MRI in Pancreas Disease
PET/MRI in Prostate Cancer
PET/MRI in Rectal Cancer
PET/MRI in Multiple Myeloma
Stay updated, free articles. Join our Telegram channel

Full access? Get Clinical Tree
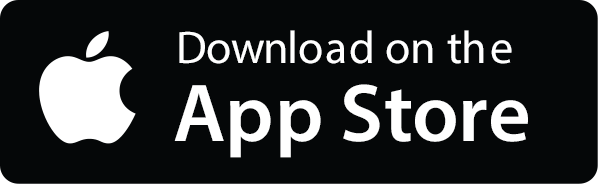
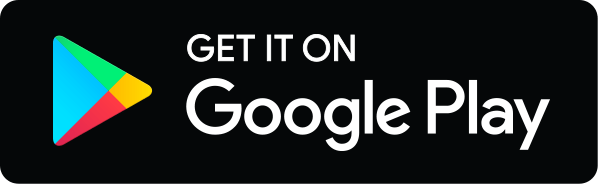