14 As a result of improvements in diagnosis, surgical techniques, and management of congenital heart disease (CHD), the number of children with CHD surviving into adulthood has increased significantly over the past 50 years. It is estimated that there are now 800,000 adults in the United States living with simple CHD and 600,000 with moderate and complex cardiac lesions.1 Although initial detection, follow-up, and treatment of CHD is largely performed by transthoracic echocardiography or transesophageal echocardiography (TEE), limitations with regard to spatial resolution, ultrasound windows in adults (especially post-surgery) and associated extracardiac anomalies render these techniques less valuable in adults than in children. Similarly, magnetic resonance imaging (MRI), although an excellent imaging modality that spares radiation exposure, may be contraindicated in those who are claustrophobic or dependent on an MRI-incompatible implanted medical device, such as a pacemaker.2 Applications of electrocardiographic (ECG)-gated cardiac CT have expanded dramatically over the past decade from assessment of coronary calcium and coronary stenosis to evaluation of cardiac anatomy, function, and structural abnormalities. When echocardiography is limited or MRI is contraindicated, cardiac CT can provide a wealth of information about the location and size of congenital cardiac defects, as well as an estimate of the degree of shunting. The full field of view obtained with high-spatial-resolution tomo-graphic images acquired within a single breath-hold is well suited to depict surgical reconstructions and the myriad vascular anastomoses present in adult patients with complex CHD. This is particularly important in the pre-operative or preprocedural planning of interventional percutaneous or surgical procedures.3 Using CT technology available in the 1980s, several groups reported the detection of interatrial shunts.4,5 Since then, faster gantry rotation speed, increased numbers of detectors, and dual-source CT scanners have allowed improved spatial and temporal resolution in cardiac CT. The advent of multiphase contrast injectors and high-concentration contrast agents (350–370 mg/mL of iodine) have dramatically improved the image quality for delineation of fine structures and shunts (ie, atrial baffles in d-transposition of the great vessels). Typical acquisition protocols for CHD require at least a 64-row multidetector CT scanner with high temporal resolution. Elimination of cardiac motion artifacts is accomplished by ECG gating. Oral or intravenous β-blockers that reduce heart rate can improve image quality but should be used with caution in patients with pulmonary hypertension, reduced ventricular function, or cardiac conduction abnormalities (ie, heart block with ventral septal defect [VSD] or tetralogy of Fallot [TOF]). Although prospective ECG gating with limited imaging during mid-late diastole may reduce radiation exposure, the dynamic nature of atrial septal defect (ASD) or VSD size may need to be assessed throughout the cardiac cycle. As with all applications of cardiac CT, it is important to tailor the examination to deliver the minimum radiation dose that will answer the clinical question. This is especially important in young individuals who, because of their disease, have often been subjected to multiple procedures using ionizing radiation. A good two-phase power injector that allows two independent rates of contrast delivery is important for evaluation of CHD. Rapid delivery of an adequately dense-contrast bolus is needed to opacify the cardiac chambers. Dense-contrast material must be flushed out of the superior vena cava to avoid beam hardening artifact. When evaluating for a left-to-right shunt, it is important to flush contrast out of the right heart with a saline chaser to achieve an adequate contrast gradient between the two sides of the heart. In a typical cardiac examination using a 64-slice scanner, about 75 mL of iodinated contrast is injected in the right antecubital fossa at 5 mL per second, followed by a 30 to 50 mL saline chaser at 3 to 4 mL per second. In the presence of anomalous venous connections, the site of contrast injection (Fig. 14.1) may be key to the identification of different cardiac or surgical shunts. Information regarding the direction of shunting in CHD is often determined from a dynamic contrast-enhanced cardiac CT. Shunt directionality may be demonstrated by comparing the density of right- and left-sided chambers at 5 and 30 seconds after contrast injection.6 A triple-phase contrast protocol using 60 mL of full-strength contrast followed by 40 mL of dilute contrast (50:50 with saline) and a 50 mL of saline flush may be useful for demonstrating shunt directionality.7 Furthermore, the orientation of a contrast jet on dynamic cardiac CT can be helpful in the differentiation of a secundum ASD from a patent foramen8 and in the evaluation of other complex CHD. Fig. 14.1 Tailored contrast injection to demonstrate bilateral superior vena cavas (SVCs). Dual-contrast injection with right-arm power injection and hand injection in the left arm. (A) Oblique coronal view demonstrates opacification of the right-sided SVC (arrowhead) with a second opacified structure coursing around the pulmonary artery (arrow). (B) Oblique sagittal view demonstrates that this second opacified structure empties into the coronary sinus (CS), identifying it as a persistent left SVC (arrow). Prior knowledge of anatomic variations may be necessary to tailor the injection technique properly for cardiac CT. ASD is the most common congenital heart anomaly in adults, after bicuspid aortic valve and mitral valve prolapse, comprising 30% of all congenital heart anomalies in adults older than 40 years.2 This figure may underestimate the actual number of ASDs in adults because many are hemodynamically insignificant and found incidentally or on autopsy. Four main types of ASD have been described in the literature: ostium secundum, ostium primum, sinus venosus, and coronary sinus. Although ostium primum, sinus venosus, and coronary sinus ASDs have no gender predilection, ostium secundum defects, the most common form of ASD, are two to three times more common in women.1 Ostium secundum and ostium primum ASDs result from the variable growth and arrest of the septum primum and septum secundum during embryologic development. Sinus venosus and coronary sinus ASDs result from abnormal formation of the atriocaval junctions and coronary sinus, respectively. Patent foramen ovale (PFO) is frequently included in the discussion of ASD as a cause for interatrial shunt, despite the lack of a structural abnormality in the interatrial septum.7,8 ASDs may be associated with other anomalies, such as anomalous pulmonary venous connection or persistent left superior vena cava. Undetected ASD can persist into adulthood and may manifest based on symptoms or an incidental finding at echocardiography. Symptomatic ASDs are usually characterized by exercise intolerance and exertional dyspnea. Additionally, longstanding ASD can result in atrial dilatation with subsequent atrial arrhythmia resulting in chest pain and shortness of breath. Less commonly, patients can present with cyanosis or paradoxical embolus. As a result of such symptoms, patients may be evaluated in the emergent setting by CT with the unexpected finding of an ASD. The secundum subtype accounts for 70% of ASDs.9 Less than 10% of secundum ASDs are associated with an anomalous pulmonary venous connection.10 The typical location for secundum ASD is in the region of the foramen ovale in the mid to cephalic portion of the interatrial septum (Fig. 14.2). Characterization of the quantity of tissue around the rim of the ASD is critical for the assessment of percutaneous therapeutic options (see following and Chapter 12). Secundum ASDs arise from disproportionate resorption of the septum primum or incomplete development of septum secundum such that the foramen ovale is incompletely covered. Excessive perforation of the septum primum may also contribute to an enlarged ostium secundum and foramen ovale. In development of the atrial septum, small fenestrations develop in the septum secundum that coalesce to form foramen secundum. Occasionally, the fenestrations do not coalesce and the septum secundum is structurally weakened, resulting in a septal aneurysm (Fig. 14.3). This form of fenestrated ASD may be challenging to define, but the septal aneurysm is often large and markedly distorts the septal architecture. Fig. 14.2 Large secundum atrial septum defect (ASD). (A) ASD is depicted by arrows in this axial view. (B) Sagittal view demonstrates a small superior rim (arrow) with an absent inferior rim (arrow?). (C) Short axis through the ASD (arrows) with imaging of the aortic outflow demonstrates the close relationship of the superior ASD lip to the aortic root (SupAo). (D) Three-dimensional volume-rendered image cut to visualize the ASD looking from the left atrium into the right atrium (RA) at the mitral valve level. IVC, inferior vena cava; SVC, superior vena cava. Secundum ASDs are rarely symptomatic during infancy and early childhood; secundum ASDs are detected during childhood because of a murmur. When secundum ASDs persist into adulthood, left-to-right shunting can progress to right ventricular failure. Whether or not an ASD produces symptoms depends largely on the size of the defect. ASD defects smaller than 5 mm in diameter generally result in a negligible shunt, whereas larger ASDs (i.e., >) often result in hemodynamically significant shunting.1 Over time, enlargement of the atria, right ventricle (RV), and pulmonary arteries results from increased flow volume (see Figs. 9.18, 9.19, and 9.20). Longstanding secundum ASDs can produce chronic atrial dilatation, leading to atrial dysrhythmia and symptoms of easy fatigability and dyspnea, with ultimate progression to pulmonary arterial hypertension. Patients with hemodynamically significant shunts who develop right ventricular failure and cardiac arrhythmias develop marked symptoms with death occurring in the fourth to sixth decades of life.1 Fig. 14.3 Large atrial septal aneurysm with multiple fenestrations. (A) Axial image demonstrates a large interatrial septal aneurysm with bowing of the septum into the right atrium. Several small fenestrations are identified based on contrast extension into the right atrium (arrows). (B) Sagittal image again demonstrates contrast extension into the right atrium from the inferior aspect of the interatrial septal aneurysm (arrow). Primum ASDs account for 20% of ASDs and arise from incomplete fusion of the free edge of the septum primum with the endocardial cushions. Primum defects are found at the caudal aspect of atrial septum near the atrioventricular (AV) septum and AV valve planes. When endocardial cushion abnormalities are present, there is abnormal development of the mitral valve; cleft mitral valve is commonly associated with primum ASDs.11 Absence of the atrial septum at the level of the valve plane may result in prolapse of a mitral leaflet into the RV (Fig. 14.4). Sinus venosus ASDs account for 10% of all ASDs. Superior sinus venosus ASDs arise from abnormal development of the superior atriocaval junction such that the posterior and superior margins of the right atrial free wall are absent, resulting in a superior vena cava that may override both atria (see Fig. 9.23). Sinus venosus ASDs involving the inferior atriocaval junction are possible (see Fig. 9.24), although the superior type is far more common. Nearly all cases of superior sinus venosus ASDs are associated with anomalous drainage of the right upper pulmonary vein (Fig. 14.5).12 Sinus venosus ASD is found in about 42% of patients with partial anomalous venous return from the right upper lobe.13 Coronary sinus anomalies have been classified into four main types: enlarged coronary sinus with or without left-to-right coronary sinus shunt, absent coronary sinus (with an ASD in the expected region of the coronary sinus), atresia of the right coronary sinus ostium, and hypoplastic coronary sinus.14 The rare coronary sinus ASD results from abnormal regression of the paired vitelline, umbilical, and cardinal veins, resulting in a partial or complete absence of the roof of the coronary sinus, which communicates directly with the left atrium (Fig. 14.6). The coronary sinus ASD is often associated with a persistent left superior vena cava (PLSVC). A morphologic classification of unroofed coronary sinus describes four subtypes: completely unroofed coronary sinus with PLSVC, completely unroofed coronary sinus without PLSVC, partially unroofed midportion of the coronary sinus, and partially unroofed terminal portion of the coronary sinus.15 In about 10 to 25% of the general population, the foramen ovale fails to close normally.16 PFO represents failure of the valve of the foramen ovale to fuse with the interatrial septum. Because there is no abnormality of the interatrial septum, PFO is not considered an ASD. A small study of 20 patients, including six patients with PFO, demonstrated the presence of a left atrial flap in the expected region of the septum primum in all six patients with PFO confirmed by TEE. Adding two additional CT criteria, the presence of a continuous column of contrast connecting the flap to the right atrium and a contrast jet into the right atrium, resulted in greater specificity for the CT diagnosis but reduced the sensitivity for PFO detection to 66%.17 Fig. 14.4 Large primum atrial septal defect. (A) Axial image demonstrates a large defect (between the arrows) where the primum atrial septum should be present. Note the partial prolapse of the anterior mitral valve leaflet into the right ventricle. (B) Sagittal image again demonstrates absence of the atrial septum in its expected location (arrows) between the right atrium and left atrium (C) Coronal image again demonstrates the lack of an interatrial septum (arrows). With the advent of closure devices,18–21 most ASDs are now closed percutaneously.22–26 Successful percutaneous closure of an ASD requires a clear understanding of the defect anatomy,11 including the definition of specific rims (infero-posterior, near the inferior vena cava and anterosuperior, around the aorta) on which the closure device is secured. Inadequate rim tissue is a predictor of poor procedural success. In a study of large ASDs (> diameter), a small posterior rim was the most important limiting factor for successful device implantation.27 In addition, proximity of the ASD to structures such as the aortic root, pulmonary veins, or coronary sinus may significantly limit placement of occluder devices because of potential vascular obstruction or occlusion.24,28 Cardiac CT is well suited for pre-operative planning in the placement of an ASD occluder device as well as for post-procedure assessment (Fig. 14.7). In the pre-operative assessment, CT demonstrates the location and size of the ASD as well as its relationship to adjacent structures. The presence of multiple ASD fenestrations may also be detected (see Fig. 9.17). In the post-procedure assessment, CT provides a unique method to evaluate device placement along with the surrounding anatomic structures as an adjunct to echocardiography in symptomatic patients.29 CT may demonstrate residual shunts or other complications such as obstruction of vascular structures, erosion through the atrial wall, or erosion into the aortic root. Fig. 14.5 Sinus venosus atrial septal defect. (A) Axial image demonstrates a communication between the right atrium (RA) and left atrium (LA) with anomalous pulmonary veins (arrows) emptying into the superior vena cava (SVC) at the level of the defect. (B) Sagittal view demonstrates that the SVC overrides both the RA and LA (arrow). (C) Coronary image demonstrates two pulmonary veins (arrows) emptying into the SVC. IVC, inferior vena cava. Fig. 14.6 Unroofed coronary sinus atiral septal defect. (A) Oblique image through the crux of the heart demonstrates a dilated coronary sinus (CS) leading into the right atrium (RA) but not clearly defined from the left atrium (LA). (B) Coronal view again demonstrates a dilated CS leading into the right atrium (RA).Unroofed coronary sinus atrial spiral defect. (C) Oblique view demonstrates a communication (arrow) between the CS and the LA. (D) Volumetric rendering demonstrates a persistent left superior vena cava (LSVC) draining into the LA (upper arrow) as well as the CS draining into the left atrium (lower arrow). Both of these connections shunt deoxygenated blood into the LA. VSDs are classified into four main types: (1) AV canal type, (2) muscular, (3) conoventricular, and (4) conal septal. AV canal type defects occur in the posterior ventricular septum at the inlet of the ventricle. Muscular ventricular septal defects occur in the muscular septum between the ventricular trabeculations, may present with multiple defects, and may have many different locations (Fig. 14.8). Conoventricular defects, often classified as perimembranous VSDs ( see Fig. 9.26), lie between the ventricular septum and the conal septum (infracristal, or below the crista supraventricularis). They may be primarily membranous or associated with malalignment of the conal septum, wherein they involve more than just membranous septum. Conal septal (or supracristal) defects are the least common and are located in the septum between the aortic and pulmonary valves. A high perimembranous VSD may be difficult to distinguish from a supracristal defect by CT (see Fig. 9.27 and Fig. 14.9). Complex defects may span different portions of the ventricular septum and may also involve the atrial septum.30,31 The severity of shunting through a VSD, the degree of LV enlargement, and patient symptoms determine whether the defect should be closed using surgical or percutaneous techniques. Infracristal and muscular defects may naturally become smaller or spontaneously close over time. Large VSDs and supracristal defects are unlikely to become smaller over time. VSDs are most often surgically patched through the tricuspid valve with either fabric or pericardium or, less commonly, may be directly oversewn. Transcatheter occlusion of VSD is an evolving technology32 mostly defined in muscular defects. High membranous defects often are in close proximity to the aortic valve and the septal leaflet of the tricuspid valve ( see Figs. 12.11 and 12.12). Placement of a percutaneous closure device in a high membranous defect may result in impaired valve function such that surgical management of larger shunts in this region may be preferable. Fig. 14.7 Amplatzer atrial septal defect (ASD) closure device. (A) Axial image demonstrates a closure device (arrow) between the left atrium and the enlarged right atrium. (B) Modified three-chamber view again demonstrates a closure device (arrow) between the left atrium and the enlarged right atrium. The superior rim of the device is adjacent to the aortic root (AO). (C) Oblique view demonstrates the splay of the device around the aortic root at the superior rim of the ASD. (D) Volumetric rendering shows the device orientation adjacent to the mitral valve annulus and just inferior to the aorta. Fig. 14.8 Muscular ventricular septal defect (VSD). (A) Short-axis view demonstrates a defect in the inferior septum (arrow). (B) Axial image again demonstrates the inferoseptal VSD (arrow) with a windsock of tissue extending into the right ventricle. (C) Cross-section of the VSD in short axis (arrow) allows measurement of the VSD diameter. Fig. 14.9 High conoventricular (perimembranous) ventral septal defect (VSD). (A) Long-axis view of the left ventricle (LV) and right ventricle (RV) demonstrates the VSD (arrow) into the right ventricular outflow tract. (B) Short-axis view again demonstrates the VSD between the left ventricular outflow tract (LVOT) and upper RV. LA, left atrium; RA, right atrium. Echocardiography demonstrates most VSDs but is limited in visualization of supracristal defects.31 Cardiac MRI can clearly demonstrate VSDs and provide quantitation of any shunt. For optimal evaluation of a VSD by CT, the left ventricle should be well opacified with relatively little opacification of the RV to permit visualization of intracardiac shunting (Fig. 14.8). Multiplanar reformatting is key to defining accurately the VSD anatomy, particularly for smaller defects or high perimembranous septal defects (Fig. 14.9). AV canal type defects may result in a common AV canal with an associated ASD or valve leaflet abnormalities. Clefts may be present in the septal leaflet of the tricuspid valve or the anterior leaflet of the mitral valve. Any abnormal attachment of the AV valves should be noted. Ventricular volumes and ejection fractions should be assessed. Initially left ventricular dilatation may occur with larger left-to-right shunts, but with time right ventricular dilatation results from the volume overload and right ventricular hypertrophy develops secondary to pulmonary hypertension and elevated right-sided pressures (see discussion of right-sided volume and pressure overload and figures in Chapter 9). Large VSDs can result in pulmonary arterial hypertension which, if untreated, may progress to Eisenmenger syndrome with reversal of the interventricular shunt (right → left). Conoventricular and conal septal defects may be associated with prolapse of the right or noncoronary cusp of the aortic valve into the defect, leading to aortic insufficiency.32 VSDs can also be associated with right or left ventricular outlet narrowing. Based on these associations with VSDs, CT assessment should include evaluation of the VSD location and size along with a review of chamber size and morphology, valvular anatomy, and careful assessment of the outflow tracts below the semilunar valves. Post-operative evaluation of VSD repair, although not routine, should focus on any leak around the patch or through the defect if primary closure was performed. TOF is the most common form of cyanotic CHD and is traditionally characterized by four abnormalities: (1) pulmonary stenosis, (2) overriding aorta, (3) VSD, and (4) right ventricular hypertrophy (Fig. 14.10), which is not an initial manifestation of TOF but usually develops after several weeks of life. It is thought that the tetralogy is actually caused by a single developmental abnormality (ie, under-development of the subpulmonary infundibulum).33 As patients grow, infundibular stenosis becomes progressively more obstructive. The most severe variant involves complete atresia of the pulmonary valve. The pulmonic valve is commonly bicuspid or unicuspid. Also, the central pulmonary arteries may be hypoplastic, diffusely or focally stenotic, discontinuous, or even absent. Pulmonary blood flow may be reliant on a patent ductus arteriosus, bronchial arteries, or aortopulmonary collateral vessels.33 Fig. 14.10 Tetralogy of Fallot with large ventricular septal defect (VSD). (A) Axial image demonstrates a large VSD (*) with overriding aorta (arrows). (B) Coronal image demonstrates mild narrowing of the pulmonary outflow tract (arrow). (C)
Congenital Heart Disease in the Adult
Congestive Heart Disease Cardiac CT Methodology
Atrial Septal Defects
Atrial Septal Defect Types
Ostium Secundum
Ostium Primum
Sinus Venosus
Coronary Sinus
Patent Foramen Ovale
Pre-Procedural Planning
Ventricular Septal Defects
Tetralogy of Fallot
Stay updated, free articles. Join our Telegram channel

Full access? Get Clinical Tree
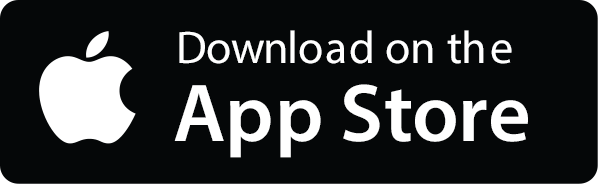
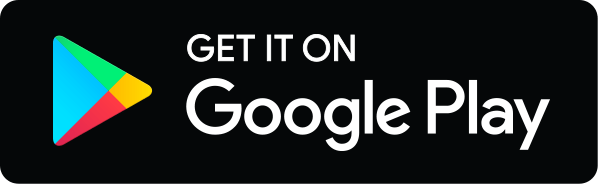