© Springer-Verlag Berlin Heidelberg 2012
Richard P. Baum (ed.)Therapeutic Nuclear MedicineMedical Radiology10.1007/174_2012_698Pharma Considerations for Timely Development of Therapeutic Radiopharmaceuticals
(1)
Bracco Research USA Ltd, ⌦, 305 College Road East, Princeton, NJ 08540, USA
Abstract
The practical aspects of systemic radiotherapy development are a hybrid between that of more traditional oncology drug development and aspects of radiation oncology. Systemic radiotherapeutics (those directly administered in an unsealed source form) are drugs and are regulated as such whereas brachytherapy (sealed) sources are regulated as devices. The regulatory development path for drugs is well defined with some special provisions for oncology drugs. Systemic radiotherapeutics are accommodated by the existing regulations quite well but there are some differences. The regulations leading to drug approval are one of the major drivers of the differences between early academic or commercial development and later efforts. To understand these differences it is necessary to understand the effect of the drivers. This chapter will outline the pathways and the differences that are taken into consideration by pharma when developing a systemic radiotherapeutic agent.
1 “Standard” drug development pathways
Drug development can be separated into two main parts, that of discovery, during which a lead drug candidate is identified and brought to a state ready for the next part, which is one of (clinical) development. The discovery part is dominated by ‘science’ and not regulated in the early stages but, as first-in-man (FIM) approaches, regulatory constraints must be understood and addressed. In contrast, the development part is dominated by regulation and ‘science’ must take a back seat if the process is to be completed efficiently. This is not to say that science is irrelevant in the development stage, on the contrary. The advent of personalized medicine coupled with more knowledge of individual patient or lesion characteristics has changed the goals of early clinical studies, especially in oncology, from merely reproducing the animal results in man to finding the best way to select and treat real patients. Overlapping the end of discovery and the beginning of the development part lies a period of translation which has elements of both.
The discovery phase traditionally includes target identification, proof of mechanism (POM), hit-to-lead progression, proof of concept (POC), lead optimization, and drug candidate selection. From a regulatory viewpoint the clinical part traditionally encompassed three phases (I, II & III) but recently an additional microdosing phase (sometimes called phase 0) has been inserted prior to phase I.
Traditional (Phase I) studies are safety studies to explore the maximum tolerated dose (MTD) and the initial administrations are performed using doses that are low relative to the no observable adverse event level (NOAEL) found in animal testing. For microdosing studies the initial administrations are even lower. The selection and justification of the initial doses in either case requires practical knowledge of toxic effects and an understanding of the dose response relationship. Thus, a new chemical entity (NCE) cannot (should not) be introduced into man without an assessment of the risk/benefit ratio which requires knowledge of its safety profile in animals. It should be obvious that in order to accurately translate the animal results into the expected behavior in man, the quality of the material used in each case should be known and be similar. This raises the concept of Good Laboratory Practices (GLPs) which is a system of control and documentation for key procedures that assures the veracity of the results (OECD 1999). A similar set of controls is in place for manufacturing, Good Manufacturing Practices (GMP), and for clinical studies, Good Clinical Practices (GCP) FDA (2009). These Practices are continually being refined and, when used, the most up to date (current) Practices are required e.g., cGMP.
It is not normally expected that phase I studies will yield real clinical responses as in most cases multiple administrations will be required. The optimal dosing regimen is usually explored during phase II studies.
The major costs for developing all drugs occur in discovery and in phase II/III and filing as reported by the US Food and Drug Administration (FDA 2004). Whereas the costs of discovery are large (about 35 % of the total development costs), they have remained fairly stable over the years, most likely because success or failure is driven by science, not regulation. In contrast, the heavily regulated clinical part shows a much larger incremental increase in costs. This is a reflection of the increased requirements by authorities that regulate approval (and more recently by those that regulate reimbursement). However, it is more difficult to accurately capture the discovery costs for compounds that are initially developed in academia or (multiple) small companies and which are then bought by a different pharma company for subsequent development and approval. This model is becoming much more common in the pharmaceutical business.
2 Target Identification and Validation
Zimmerman (see “Economic and Industrial Considerations for Marketing of Therapeutic Radiopharmaceuticals”) has discussed the selection process for the radionuclide and the targeting moiety and Wester (see “Molecular Targets for Nuclear Medicine Theray”) has described the target themselves. Ideally, the target should not be present in normal tissue but should be present in the cancer tissue at a sufficient level to concentrate the desired radioactivity. Some presence in normal tissue is acceptable but it may reduce the therapeutic index, although in many cases the excretory organs are the critical dose-limiting normal tissues. There is no one desirable type and placement of the target in the cancer tissue. In some cases, for instance when using short range Auger electron or alpha emitters, it is necessary that the radioactivity be close to the molecular target (DNA). One would thus desire the target to be inside the cell (with some nonspecific means of internalizing the radiopharmaceutical), from a physical point of view and with selectivity determined by the target, or on the cell membrane with internalization subsequent to specific binding. For higher energy gamma or beta emitters it may also be desirable to achieve internalization to increase the retention time. It is not essential that the target be on a cancer cell, the significant range of some emissions does allow the target to be in the stroma with destruction of close cancer cells by the bystander effect. Such is the case when using bone seeking radiotherapeutics such as 153Sm EDTMP, 89SrCl2 or 223RaCl2. This may present an advantage in that access to the stroma may be easier than to a cancer cell and the concentration of target can be higher. The bystander effect also, of course, means that the target need not be on every cancer cell in a tumor. This too is an advantage due to the heterogeneity of tumor cellularity and may be particularly important for killing tumor stem cells. These may not have the receptors or growth characteristics that allow targeting common to the daughter cancer cells.
The first barrier that an intravenously administered radiotherapeutic meets is the lining of the vasculature. This can be a significant impediment to larger entities including antibodies but it also represents an interesting place to target if sufficient selectivity can be obtained for tumor-associated vasculature. Targets associated with angiogenesis may fit the bill and it has been shown that endothelial cells are susceptible to radiation damage (Garcia-Barros 2003).
Target validation in this context relates to knowledge of the distribution of the target in normal and cancerous tissue. When considering well known targets this is more a question of validating existing information using the constraints required by systemic radiotherapy. Zimmerman (see “Economic and Industrial Considerations for Marketing of Therapeutic Radiopharmaceuticals”) has discussed factors to be taken into consideration to match the physical and chemical characteristics of the radionuclide with the proposed pharmacokinetics which in turn determine how accessible the proposed target must be. For a relatively unknown target the tissue distribution needs to be generated. This can present problems depending on the type of target. For instance, it may be inappropriate to use immunohistochemical techniques to probe tissues if one is considering an active uptake mechanism because antibodies normally identify presence of antigen, not activity of the antigen. Thus, an antibody may bind to both an active and mutated form of a G protein-coupled receptor (GPCR)—which occur frequently in cancer cells lacking internalization as a result of the mutation. For instance, some cancer mutations change a receptor to an always-on mode that not only negates the need for activation with a ligand, but also obviates the need for internalization to remove the ligand to stop signaling. This could lead to inappropriate validation of the target with subsequent failure of the proof of concept. (vide infra). In such a case, it is better to employ a detectable compound related to the known ligand for the proposed target e.g., octreotide for the somatostatin receptors. Under such circumstances frozen tissue and living cells may be required to generate all the answers needed, paraffin fixed tissue is rarely suitable. It is also very important to examine both human and animal tissue: the former to validate the final target and the latter to identify suitable animal models. Herein, lies a problem for there are often significant species differences between the reactivity of antibodies and to a lesser extent peptides and small molecule ligands to natural targets.
A final aspect of target validation from the pharma point of view relates to the potential market for a drug. Sufficient data need to be available to document the presence of target in multiple human samples from tumors with sufficient numbers to provide a financial return. Initial data can be somewhat sketchy but by the time translation is reached, when significant funds are at stake, the data need to be sufficient to support further spending. (see “Economic and Industrial Considerations for Marketing of Therapeutic Radiopharmaceuticals”) In this regard, there is often a disconnect between the best population in which to demonstrate efficacy in a clinical environment and that to support a commercial indication.
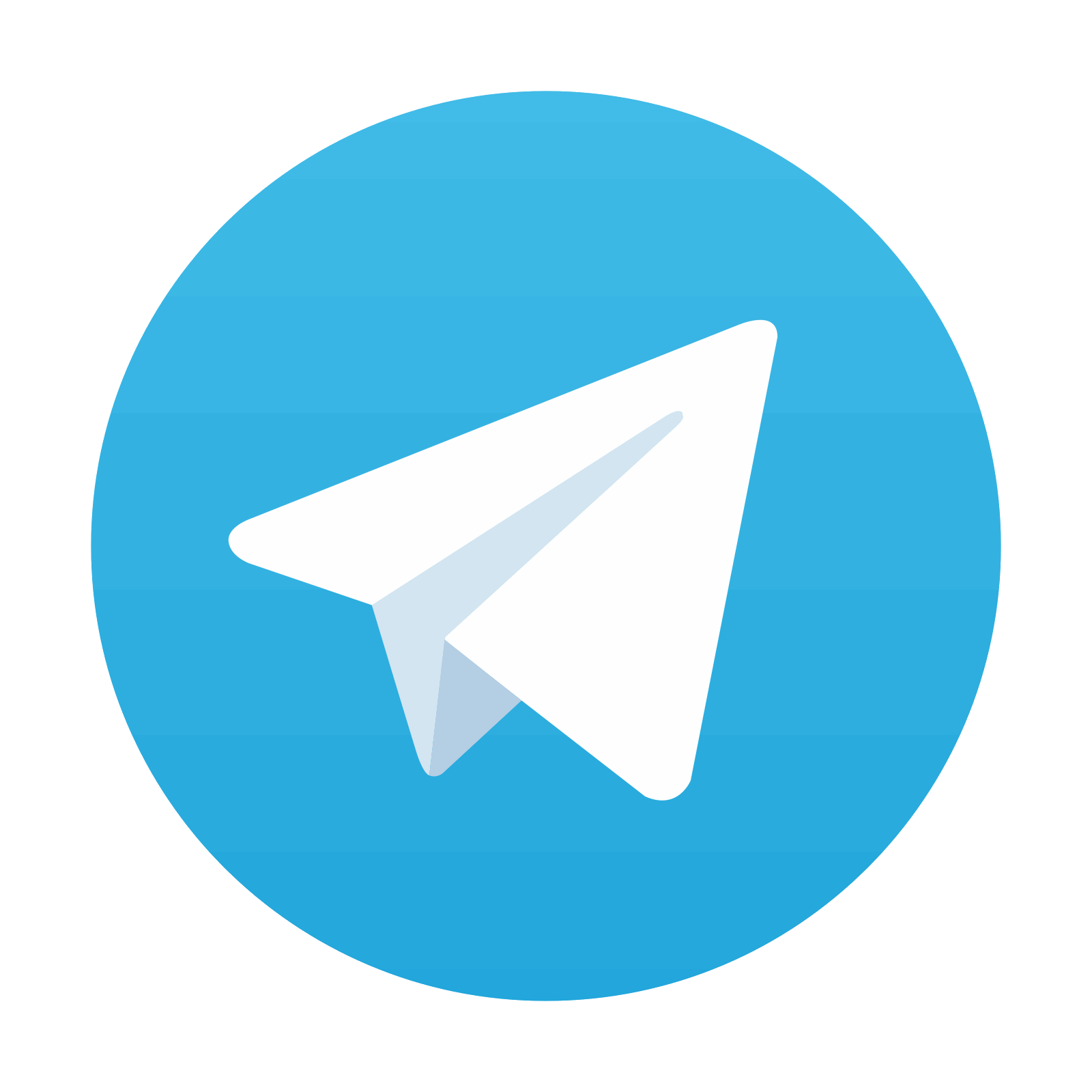
Stay updated, free articles. Join our Telegram channel

Full access? Get Clinical Tree
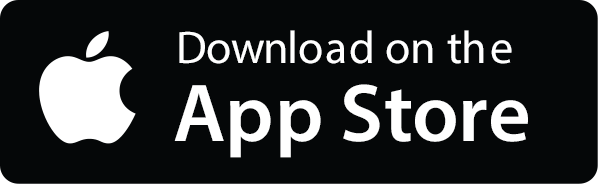
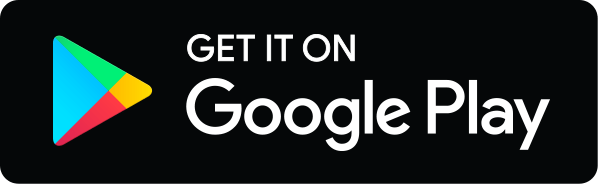