Aggressive histologic types
Diffuse large B cell lymphoma
Mantle cell lymphoma
Lymphoblastic lymphoma
CNS lymphoma
Immunoblastic lymphoma
AIDS-related lymphoma
Indolent histologic subtypes
Follicular lymphomas
Diffuse small lymphocytic lymphomas/CLL
Lymphoplasmacytic lymphomas
Marginal zone lymphomas
Patients with high risk NHL histologic types are treated with various combination therapies with intent to cure disease. Those with early stage disease often receive short course chemotherapy with localized field radiation to involved nodal anatomic groups. Patients with aggressive advanced stage disease receive combination chemotherapy. Even with the most up-to-date treatment combinations, most patients are not cured and are offered high dose chemotherapy with hematopoietic stem cell transplantation. A recent review noted that 30–50 % of patients with aggressive lymphomas achieve long-term remission with stem cell transplant (Baldissera et al. 2006). Similarly, NHL patients with lower risk histologic type tumors are not usually cured of their disease with standard therapy. Attempts to increase cure rates using higher toxicity approaches have not resulted in increased overall survival (Baldissera et al. 2006).
Radioimmunotherapy for NHL was developed as an approach to increase long-term remission and cure rates for patient in both risk categories (Corcoran et al. 1997; Moskowitz 1999; Shimoni and Nagler 2007). Because of the increased cure rates for the leukemias using high dose chemo-radiotherapy combinations and bone marrow transplantation, this conceptual approach for treating NHL with high dose radioimmunotherapy was designed. These treatment approaches were pioneered by the University of Washington/Fred Hutchison Cancer Research Center Group (UW/FHCRC) in Seattle (Eary et al. 1990; Press et al. 1990, 1993a, b, 1995). The anti-CD-20 antibody radiolabeled with I-131 was used. This therapy strategy was based on normal organ dosimetry estimation for treatment radiation dose escalation to normal organs and prediction of dose limiting toxicity. In this review, high dose radioimmunotherapy concepts are presented in the following historical perspective of this group’s early work. An updated review is presented on clinical experience by groups working in this area in subsequent sections.
2 High Dose Radioimmunotherapy Historical Perspective
The UW/FHCRC high dose radioimmunotherapy work involved development of several new concepts for radiochemistry, gamma imaging, radiation absorbed dose estimation, radiation safety, and toxicity evaluation. Careful studies of radiolabeled antibody biodistribution were performed followed by individualized radiation absorbed dose calculations for normal organs. The Phase I studies were dose seeking clinical trials where dose escalations were based on these individualized normal organ dose estimates.
2.1 Radiochemistry
The UW radiochemistry group used the standard chloramine-T radioiodination technique for antibody preparation at both low and high specific activities (Eary et al. 1990). Radiolabeled antibody was separated from reactants using a size exclusion column and collection of fractionated protein containing 1 cc samples. Tumor specific antibodies may be subject to decreases in immunoreactivity when radiolabeled at high specific activities, and high total doses are envisioned. In preparation for high dose therapy, the anti-CD-20 antibodies available were carefully tested for preservation of immunointegrity through a range of specific activities (Kishore et al. 1986). For anti-CD-20 antibodies used in the UW/FHCRC clinical trials, a radioiodination level of 10 mCi/mg was the specific activity limit. Radioiodination occurs on available protein tyrosine and histidine residues in stoichiometric ratios. At high introduced levels, the large atomic radius of radioiodine can interfere with the antibody binding region and at conformational change points in the protein that can occur with specific antigen binding. Additionally, high specific activity radioiodine causes the labeled antibody to undergo radiolysis from internally generated reactive species in the therapy dose preparation solution. These changes can lead to rapid reductions in radiolabeled antibody immunoreactivity as well. With these discoveries, the antibodies for high dose clinical trials were prepared in volumes of 0.5–1.0 l. Later, addition of free-radical scavengers were contemplated to increase the stability of the radiolabeled product.
2.2 Radiolabeled Antibody Biodistribution and Radiation Absorbed Dose Estimation
For the high dose studies, quantitative imaging techniques were required for radiolabeled antibody biodistribution determination for individualized normal organ radiation absorbed dose estimates. This was because large differences in quantity in CD20 antigen bearing lymphocyte and lymphoma populations in patients were expected, that would result in large variations in radiolabeled antibody biodistributions between patients, causing variability in normal organ radiation absorbed doses at fixed antibody protein amounts. At the outset, patients received one or more trace labeled radioiodinated anti-CD-20 antibody biodistribution studies to find the optimum antibody quantity to show an advantage in therapy by specific tumor localization. Radioiodine is difficult to image for determination of radioactivity quantity in an organ. Several studies were carried out to determine the method for quantitation in radiolabeled antibody patients. Biodistribution determination involved a series of images acquired over approximately a week. An opposing view technique was used (Eary et al. 1989, 1990; Durack and Eary 1995). Template-based images were acquired for exact patient re-positioning for outline of organs determined by pre-study Tc-99m agent imaging. The lung volumes for I-131 registration were determined and registered following a Tc-99m macroaggregated albumin (MAA) dose. The liver volume was visualized with a Tc-99m sulfur colloid dose. These organ outlines were then applied to the I-131 anterior and posterior I-131 antibody images on subsequent imaging sets. The attenuation correction for each organ measurement was made by obtaining the organ geometric mean counts per region of interest. This method was validated in a torso phantom with fillable organ cavities. In initial studies, the kidney volumes were visualized with a dose of Tc-99m DTPA, but later, kidney activity was made with the use of depth attenuation calculations on kidney activity image outlines. No attempts were made to determine tumor uptake with this method since partial volume effects created significant error. In early study of patients, tumors were biopsied and counted with comparison to a dose standard aliquot to determine radioiodine content for scaling image derived time activity curves.
These detailed image quantitative biodistribution studies showed that the I-131 anti-CD-20 antibodies showed the most favorable biodistribution for therapy when large antibody quantities were administered (Eary et al. 1989, 1997). This presumably allowed maximum binding to all CD-20 positive lymphocyte populations (tumor and normal lymphocytes). The whole-body blood clearance half times were prolonged at these doses, indicating slowed metabolism and enough blood concentration prolongation to allow for antibody tumor penetration. The antibody was noninternalizing which facilitated biodistribution for high dose therapy. Infused trace radiolabeled antibody in biopsied tumor after biodistribution doses were visualized with immunoperoxidase. These studies showed that at high infused antibody quantity, the antibody penetrated into tumor parenchyma beyond the peri-vascular location observed at lower doses. These findings helped confirm the necessity of large antibody quantity for optimal biodistribution for radioimmunotherapy.
Although radiochemistry tests of radiolabeled antibody function integrity had defined the upper limits of specific activity, there was concern that the biodistribution of the trace radiolabeled entire antibody quantity would not be predictive of the high specific activity antibody behavior in therapy. Would it be the same? The concept of high dose therapy for the Seattle group was that the normal organ radiation dose estimates were to be used to determine radioactivity and antibody amount for dose escalation and toxicity determinations. Inherent in this strategy was the assumption that the biodistribution of the I-131 trace labeled antibody dose was the same as the high dose formula since the antibody quantity infused is the determinant of biodistribution. In an early study, Eary et al, took images of patients treated at high dose anti-CD-20 I-131 antibody therapy (500–1000 mCi) after treatment to determine the specific biodistribution of these high doses. They imaged these patients daily on a prototype gamma camera with nonparalyzable electronics and a special long bore I-131 collimator designed for this purpose. These data showed that the biodistribution of the high doses paralleled that of the low dose labeled studies in these patients acquired before therapy, confirming the ability of the low dose biodistribution to be predictive of high dose therapy radiation absorbed dose estimates (Eary et al. 1994; Pollard et al. 1992, 1996).
At the same time, the dosimetry group developed modified MIRD procedures to make radiation absorbed dose estimates (Fisher et al. 1991; Rajendran et al. 2004). These required specific consistent data inputs obtained from quantitative I-131 gamma camera imaging for organ radioiodine content, blood clearance, and if available, tissue concentrations in tumor and marrow. Later, these techniques were analyzed to assess tumor dose assumptions based antibody localization (Hui et al. 1992).
2.3 Radiation Safety
High I-131 patient administered doses also required development of a number of radiation safety procedures. The high dose radiolabeling laboratory setup was designed to reside in a standard laboratory fume hood with a reinforced work floor. This hood was vented through a charcoal filter. The fume hood work space was shielded with 2.5 inch thick lead bricks and a specially fabricated steel framed lead door for replacing radiolabeling apparatus components for each synthesis. High dose radiolabeling procedures required use of a specially designed shielded reaction set-up. Antibody radiolabeling was performed in the radioiodine transport container by introduction of reactants through the vial rubber diaphragm. The reactants were added through a long tubing with a needle fitting and removed with a peristaltic pump to the separation column after radiolabeling completion. Protein containing eluate fractions were transferred into the infusion intravenous fluid bag through a long tubing with the use of the same peristaltic pump. The safe transport of the doses occurred with development of a reinforced stack of 3 inch thick split lead rings fitted to a steel framed cart. The dose containing intravenous fluid bag was suspended in this stack cavity with standard tubing fitted with a filter exiting the bottom. The entire device had a low center of gravity with the bottom approximately 6 inches off the floor. The dose was delivered to the patient with a clinical peristaltic pump for intravenous fluids set to run at a constant rate via drip monitor. All patients had double lumen central venous lines in place for treatment and eventual transplant.
The concerns of radiation safety for other patients and personnel on the hospital treatment floor were addressed by leaving the adjacent patient room un-occupied. Later, radioiodine treatment hospital floor rooms were modified with lead lined walls, floors, and ceilings. During this time, other aspects of radiation safety were investigated by McBaugh (1989). She found that low radioiodine air levels occurred in these rooms, so the special treatment rooms were modified for negative air flow with the ventilation directed through a charcoal filter. These rooms also were modified to have stainless steel surfaces to reduce masonry contamination problems. All of these designs led to successful dose escalation studies and toxicity level determinations.
2.4 Care of the Patient
The patient received the high dose radiolabeled antibody through infusion into a central line indwelling catheter. A low level infusion of normal saline was kept connected for approximately the entire patient hospital stay. The room was prepared similar to that for thyroid cancer patient treatment with surfaces covered completely, and the use of disposable eating utensils. A standard 3 ft high lead radiation shield was placed across the patient room door mostly as a barrier reminder for visitors. As per the institutional procedures, the radiation dose rate at 1 m was obtained by the Radiation Safety officer after treatment completion and daily until discharge (dose rate of 7 mrem or less at 1 m). An exposure map of the room with the patient positioned in the bed was posted on the door along with the daily radiation dose exposure rates measured at 1 m. Most patients remained in radiation isolation for approximately 1 week to 10 days. Patient care was provided by nursing staff who wore radiation badges. They performed their usual vital signs monitoring at prescribed intervals, and conducted their patient interviews from behind the waist high door shield. Staff was instructed to perform care as needed but to limit direct patient contact time. Of course, in an emergency, they were to provide necessary care with rotation of personnel to reduce individual exposure if needed. Meals were handed over to the patient at the hospital room door, and meal wastes were stored in lined containers in the patient’s room. Patients could be seen in the room at all times over the door portable shield. On occasion, research staff would supervise the patient for an outing to climb the unused six storied stairwell next to the treatment room for some exercise at off hours such as at 5 am before the hospital floor hallways became busy.
2.5 UW/FHCRC Clinical Trials
The Phase I clinical trials for high dose I-131 anti-CD-20 antibody with bone marrow transplant began at 10 Gy estimated radiation dose to the highest normal organ. This was most often lung, but in several cases was the liver. Dose escalation continued to 30.75 Gy (Press et al. 1993a, b). Patients received autologous marrow when whole-body total dose remaining reached 10 cGy. This limit was arbitrarily set to ensure marrow engraftment. The maximally tolerated dose was determined to be approximately 27 Gy to the lung. Doses above this level were associated with reversible cardiopulmonary toxicity that occurred in two patients. On average, tumor received approximately 10 times the radiation absorbed dose compared to the whole-body levels and usually twice the dose of nonmarrow critical organs such as the lung. Nonmarrow toxicities were very mild. Overall, this high radiation absorbed dose resulted in improved response rates in the patient cohorts treated. Overall response rates were 95 with 84 % complete response rates. Further analysis of these response patterns showed that patients who received 20 Gy or higher to the highest normal organ had longer progression free survival compared to patients in lower dose cohorts (Press et al. 1995). This observation confirmed the predictive capability of the quantitative dose estimation by showing a clinical dose response relationship. The phase II study that followed in patients with relapsed NHL showed an initial response rate of 86 with 79 % complete responses. A 42-month follow-up showing 68 overall 42 % progression free response (Press et al. 1995; Liu et al. 1998). Long-term follow-up in these 29 patients showed 14 of the 29 patients in remission. The late toxicity observed was elevated TSH levels in 60 % of patients (Liu et al. 1998). This occurred in spite of pre-treatment with oral iodide solution which does not produce complete thyroid uptake blockade of I-131 metabolized from slow rates of de-iodination of the radioimmunoconjugate. Two patients in the group developed second malignancies, but neither of these were myelodysplasia.
A multivariate cohort analysis comparing relapsed follicular lymphoma patients receiving high dose radioiodine radioimmunotherapy to a high dose chemotherapy/whole-body radiation therapy group, both with hematopoietic stem cell transplant was performed including patients described in the early phase studies (Gopal et al. 2003). These analyses showed improved overall (67 %) and progression free survival (48 %) for radioimmunotherapy patients compared to the conventional high dose therapy group who had overall (53 %) and progression free (29 %) survival. The 100-day mortality rate for high dose radioimmunotherapy was 3.7 % and for conventional high dose therapy was 11 %. This was interpreted as further evidence that high dose radioimmunotherapy may be a means of increasing response rates without increases in treatment regimen induced toxicities.
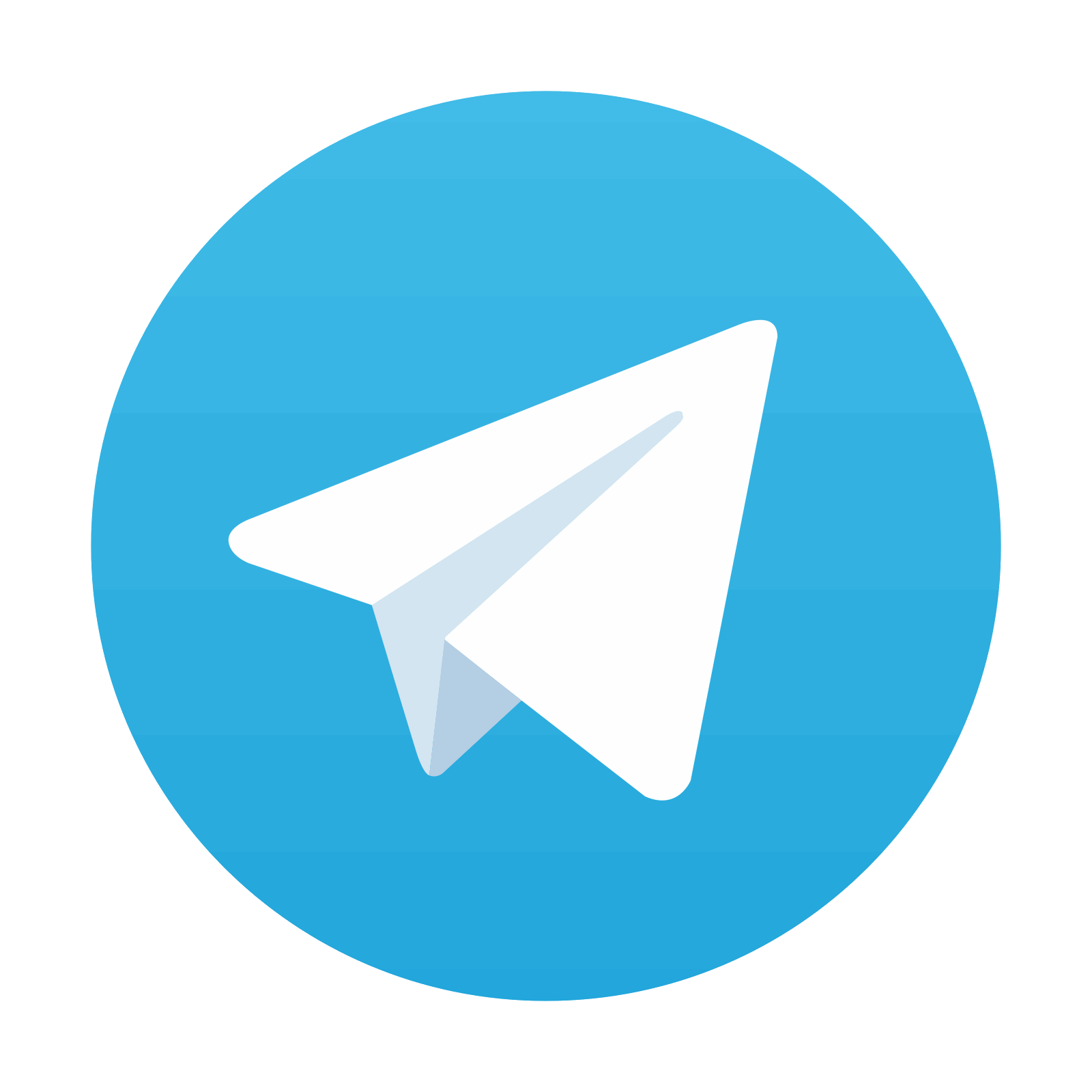
Stay updated, free articles. Join our Telegram channel

Full access? Get Clinical Tree
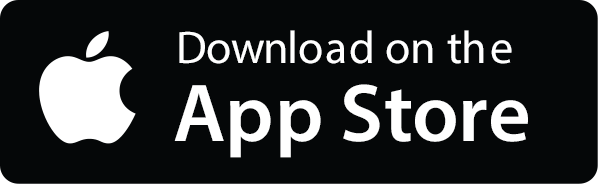
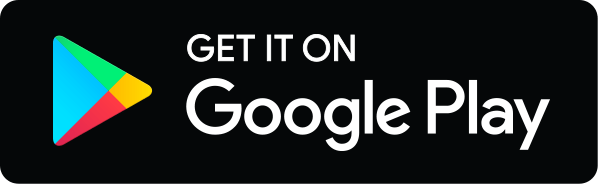