Sana Salehi1, Farshad Moradi2, Doina Piciu3, Hojjat Ahmadzadehfar4, and Ali Gholamrezanezhad1 1 Department of Radiology, Keck School of Medicine, University of Southern California, Los Angeles, CA, USA 2 Department of Radiology, Division of Nuclear Medicine, Stanford, CA, USA 3 Department of Endocrine Tumors and Nuclear Medicine, Institute of Oncology Ion Chiricuta and University of Medicine Iuliu Hatieganu, Cluj‐Napoca, Romania 4 Department of Nuclear Medicine, Klinikum Westfalen, Dortmund, Germany Integration of functional/metabolic imaging and conventional imaging techniques in many situations can help diagnosticians to better detect or characterize pathologies. In this chapter, we describe the imaging features of endocrine diseases obtained by available modalities, discuss the preferred diagnostic approach and their indications, and compare the manifestation of specific lesions in various imaging techniques. The thyroid gland is normally situated in the lower neck at midline, anterior and anterolateral to the larynx and upper tracheal rings, and comprises two lobes (typically 5 ×3 × 2 cm in adults) which are linked together by an isthmus. Common carotid arteries and sternocleidomastoid muscles are located laterally to the gland [1, 2]. Thyroid nodules are common findings among the adult population with an incidence detected on thyroid ultrasound of up to 70%. However, only a small percentage of them have clinical significance. In recent years, detection of incidental thyroid nodules has increased due to extensive access to imaging modalities and improvement in imaging techniques [3–5]. When a thyroid nodule is detected, it is important to rule out malignancy (in 4–6.5%), and assess their function and possible compression effects [3, 6]. The first step in the evaluation of a patient diagnosed with a thyroid nodule is to obtain a comprehensive medical history and physical examination. Rapid growth, prior head and neck irradiation, history of certain syndromes (e.g. Cowden, multiple endocrine neoplasia type 2), family history of thyroid cancer, cervical lymphadenopathy, or vocal cord paralysis are associated with malignancy [5]. The next step is to assess thyroid function. Frequently, nodular goiter (defined as thyroid enlargement associated with thyroid nodules) has a normal function, with serum thyroid hormones levels within the normal ranges. The normal, high or low thyroid stimulating hormone (TSH) serum levels do not exclude cancer. In individuals with low TSH evaluation includes thyroid scintigraphy/radionuclide scan [5, 7]. The association of thyroid nodules with lymphocytic autoimmune thyroiditis might increase the risk of malignancy, thus serum thyroid antibodies (antithyroglobulin and thyroid peroxidase antibody) should be determined [8, 9]. The next step in the evaluation of a thyroid nodule is thyroid ultrasound. A normal thyroid gland on ultrasound appears homogenous and somewhat hyperechoic in comparison with adjacent tissues. Ultrasound imaging is indicated in all individuals with suspected or known thyroid nodule to confirm the existence of the nodule, search for further nodules and lymph nodes, define the ultrasound features of the nodule and assess its extension [1, 3, 10], and guide fine needle aspiration (FNA) when biopsy is indicated. In different scenarios, the sensitivity and specificity of this diagnostic method can vary from 65% to 98% and from 55% to 100%, respectively [11, 12]. According to the American Thyroid Association Guideline [7], indications for FNA biopsy are nodules greater than 1 cm in size which have characteristics suspicious of malignancy, such as hypoechoic parts, irregularity of the borders, rim calcification, or microcalcification. Nevertheless, a significant number of biopsies remain inconclusive [1, 6, 7, 10]. According to the ACR Thyroid Imaging, Reporting and Data System (TI‐RADS), alarming features of an incidental thyroid nodule on ultrasound that require supplementary evaluation by dedicated thyroid ultrasound consist of notable hypoechogenicity, microcalcification, irregular margins, lobulated margins, “taller‐than‐wide” outline on transverse view, and the presence of latero‐cervical lymph nodes with microcalcification [13]. Isoechoic or hyperechoic nodules, with smooth margins and without calcification, have low chances of malignancy [14]. Thyroid elastography is not routinely used worldwide and its use is by far less frequent than standard B‐mode and Doppler ultrasound [15]. In patients with a thyroid nodule accompanied by a low serum TSH value, thyroid scintigraphy is recommended to evaluate whether or not the nodule is functioning [3], and also in case of multinodular goiter to orientate the FNA, especially in areas with iodine deficiency. Planar or three‐dimensional single photon emission tomography (SPECT) images are obtained 6–24 hours after radioiodine (typically sodium 123I) or 20 minutes after intravenous administration of 99mTc Pt. Nodules are characterized based on radiotracer uptake on thyroid scintigraphy as hot (higher uptake than the normal thyroid tissue), warm (equal uptake) or cold (lower uptake) [7]. Hyperfunctioning nodules have high uptake whereas background uptake in normal thyroid tissue is typically suppressed depending on TSH levels [16–18]. In contrast, background thyroid uptake is diffusely increased in Graves’ disease (Figure 16.1), which can have concomitant nodules in about a third of cases [19]. Hot nodules in adults are unlikely to be malignant but this feature does not exclude malignancy. Warm and cold nodules require FNA to exclude malignancy based on sonographic features. Discordant nodules (hot on pertechnetate and cold on iodine scintigraphy) are rare and suggest an organification defect which can appear in malignancy. Radioiodine uptake at 24 hours is useful for assessment of multinodular goiter and candidates for radioiodine therapy. Thyroid scintigraphy and uptake are useful in the differential diagnostic and assessment of acute/subacute thyroiditis and in the detection of ectopic thyroid tissue. Although side‐by‐side comparison of ultrasound (US) and SPECT, and integration of the results is usually beneficial for evaluation of thyroid nodules, in some conditions it can yield uncertain results. SPECT/US fusion imaging has proven to be helpful in cases with indeterminate findings and cytologic FNA results [20]. Pentavalent 99mTc‐dimercaptosuccinic acid (DMSA) scintigraphy has been historically used for diagnosis and follow‐up of patients with thyroid medullary carcinoma which arises from para‐follicular thyroid C cells [21]. 99mTc‐sesta‐methoxyisobutylisonitrile (99mTc‐MIBI/sestamibi) is not specific for thyroid cancers; tracer uptake is proportionate to the mitochondrial respiratory chain activity. Uptake in thyroid neoplasms, including medullary thyroid carcinoma, can be incidentally seen in sestamibi parathyroid scans or myocardial perfusion imaging studies [22, 23] (Figure 16.2). Figure 16.1 Radioiodine thyroid planar scintigraphy anterior–posterior views and uptake in two patients with clinical hyperthyroidism, severely suppressed TSH, and goiter. (a) 87‐year‐old female with multinodular goiter on ultrasound. The overall uptake is within the normal range (10–30% at 24 hours), but there is focal uptake in a right thyroid nodule consistent with an autonomous functional adenoma while uptake in the rest of the gland is moderately suppressed. (b) 55‐year‐old female with diffusely increased uptake throughout the thyroid gland consistent with Grave’s disease. There is increasing use of tumor scintigraphy using 99mTc‐MIBI, especially in some European countries for assessing thyroid nodules. According to various publications, the negative predictive value of a 99mTc‐MIBI scintigraphy of an iso or hypofunctioning thyroid nodule in 99mTc‐pertechnetate scintigraphy is more than 90%, while the positive predictive value is approximately 15–20% [24, 25]. Based on the available data, the following indications for 99mTc‐MIBI scintigraphy are sensible and justified [24]: In amiodarone induced thyrotoxicosis (AIT), a 99mTc‐MIBI scan is an essential tool in the differential diagnosis of different forms. The scan will show a normal or intense uptake of the radiotracer in AIT I form that will benefit from antithyroid drugs and has no indication for corticosteroids, and a decrease or complete lack of uptake in AIT II, where the inflammation is dominant and corticotherapy is mandatory. In form AIT III, both early and delayed images show low/faint 99mTc‐MIBI uptake. Positron emission tomography (PET) is not routinely used for evaluation of benign thyroid diseases. However, in some cases a thyroid pathology may be incidentally found while undergoing PET/computed tomography (CT) or PET/magnetic resonance imaging (MRI) for other diagnostic purposes [22]. Incidental focal increased 18F‐FDG uptake is linked with high incidence of thyroid malignancy (27–47%) [26], especially when the maximum standardized uptake value (SUVmax) is higher than 6 (Figure 16.3). Diffuse metabolic activity is usually suggestive of an inflammatory condition such as Hashimoto thyroiditis [26] (Figure 16.4). With the increasing role of immunotherapy in cancer, the early response to therapy may be evaluated by molecular PET/CT imaging. Development of thyroiditis may particularly represent an early response indicator to immunotherapy and PET‐detectable immunotherapy response adverse effects (IRAE) is useful for prediction of a favorable outcome [27]. The same reaction might also be observed in adrenal glands, showing an adrenalitis posttherapy (Figure 16.5). Incidentally detected lesions on 18F‐FDG PET/CT can be evaluated by ultrasound and FNA biopsy. In cases with diffuse uptake, measurement of TSH and thyroid antibody is suggested [26, 28]. Thyroid adenomas may demonstrate focal uptake on 68Ga‐DOTA‐DPhe1,Tyr3‐octreotate (DOTATATE) PET/CT or other 68Ga‐labeled somatostatin analogues (SSA) [29]. SSA and 18F‐DOPA PET/CT are also useful for assessment of medullary thyroid carcinoma, which is discussed in the neuroendocrine section [30, 31]. Figure 16.2 99mTc‐MIBI scintigraphy for differentiation of malignant thyroid lesions in a 40‐year‐old female with a cold nodule on 99mTc thyroid scan (a). Early (b) and delayed (c) phase 99mTc‐MIBI scintigraphy demonstrate obvious retention of 99mTc‐MIBI. FNA biopsy of the patient indicated a benign lesion, but the excision biopsy showed papillary thyroid carcinoma. Figure 16.3 18F‐FDG PET/CT coronal view (a) and pelvic axial view (d) of a patient with cervix carcinoma during follow‐up showing multiple metastatic lymph nodes in the pelvic region (white arrows) and pathologic increased uptake (SUVmax 18.1) in the right thyroid lobe (red arrow, axial view b) with right latero‐cervical lymphadenopathy (red arrow, axial cervical view c). The histology after thyroidectomy revealed synchronous papillary thyroid carcinoma with lymph node metastases. 18F‐FDG PET is an essential tool in the evaluation of patients with differentiated thyroid cancer (DTC), detectable serum thyroglobulin level, and negative radioiodine scintigraphy (TENIS syndrome). In such patients, 18F‐FDG PET/CT is complementary to 124I‐PET (when available), which is more sensitive than scintigraphy and SPECT for assessment of iodine avidity [32]. There is an increasing detection rate of F18‐FDG PET/CT in radioiodine negative patients with Tg levels higher than 10 ng/mL [33], being a marker of the aggressiveness of the tumor; its role is also very important in case of early assessment, staging, and response evaluation in cases with an aggressive form of DTC, in medullary thyroid cancers, and in anaplastic forms. 68Ga‐PSMA PET/CT is being investigated in metastatic thyroid cancer and appears to detect both iodine avid and nonavid lesions with high sensitivity [34]. Figure 16.4 18F‐FDG PET/CT coronal view. Diffuse increased 18F‐FDG uptake suggestive of lymphocytic chronic thyroiditis. Figure 16.5 Coronal view 18F‐FDG PET/CT in a 25‐year‐old male patient with left axillary metastatic malignant melanoma, showing increased diffuse uptake in liver (hepatitis) and thyroid (thyroiditis) suggestive of adverse effects to immune checkpoint inhibitor therapy. In patients with medullary thyroid carcinoma, the detection rate of PET for recurrent or metastatic disease correlates with post‐thyroidectomy serum calcitonin levels [35, 36]. Differentiated medullary thyroid cancer has high uptake on 18F‐DOPA or 68Ga‐SSA PET/CT, whereas high 18F‐FDG uptake is associated with worse prognosis and dedifferentiated cancers [36, 37]. 124I‐PET/US fusion imaging has lately been presented for assessment of thyroid nodules and is believed to have some advantages over conventional diagnostic methods and 99mTc‐SPECT/US fusion imaging in terms of functional assessment of thyroid nodules. However, there are some limitations, such as an increase in radiation exposure and patient selection, that have to be considered [38]. Although CT and MRI yield detailed structural information, they have limited utility in the evaluation of thyroid nodules. However, they can be very useful in demonstrating the local extent of the malignancy invasion and distant metastasis [39]. CT and MRI can be helpful in localization of the parathyroid glands before operation. If CT is indicated for assessment of the thyroid gland, it should be performed without contrast [2]. If the intravenous contrast is necessary for the imaging and the patient is going to undergo scintigraphy or radioiodine therapy, then MRI can be used as a substitute for a CT scan. The thyroid gland appears hyperintense and homogenous on T2 MRI images. In some pathologies, such as multinodular goiter, the thyroid may appear heterogeneous. Pathologic lymph nodes can be hyper enhanced on T1 images [2]. The incidence of DTC has been increasing and it is forecast to be one of the four most common malignancies by 2030 [40]. DTC is divided into papillary and follicular carcinomas, based on its histological features [41]. The first treatment step after diagnosis of DTCs is surgical thyroidectomy and removal of accessible metastatic sites. Thyroid remnant ablation using radioactive 131I is considered following surgery for localized disease depending on risk of recurrence. Radioiodine therapy with 131I after thyroidectomy is often indicated for metastatic disease as either adjuvant or primary therapy. In some cases, like unresectable tumors or radioiodine refractory disease, further treatments such as radiotherapy and chemotherapy may be necessary. Thyroid hormone substitution, in some cases in suppressive doses, needs to be administrated. A lifelong follow‐up is performed for these patients by periodic ultrasounds, radioiodine imaging, and measurement of thyroglobulin levels [41, 42]. Planar scintigraphy (with or without SPECT) using 123I or 131I is the standard of care in the postoperative follow‐up of patients with DTC (Figure 16.6). 131I is used for detection and ablation of the remnants of the thyroid gland after surgical removal of the gland. Despite their major diagnostic and therapeutic roles, these imaging modalities do not provide the detailed anatomic information needed to localize and characterize pathologies. Recently, combination of these modalities with a CT scanner (PET/CT and SPECT/CT) has led to the development of hybrid imaging methods that overcome the limitations of molecular imaging and enhance the diagnostic accuracy [41, 43–45]. In patients in whom recurrence of the disease is suspected, thyroglobulin values are increased, and no uptake is seen in the 131I scan, 18F‐FDG PET/CT can be a useful modality in locating the recurrence spot with sensitivity and specificity of up to 85% and 90%, respectively [44]. However, this technique may have some disadvantages, including a significant number of false‐negative cases (up to 40%) [44]. 18F‐FDG‐PET/CT is generally indicated in patients with aggressive DTC (Figure 16.7), dedifferentiated or anaplastic thyroid carcinomas (Figure 16.8), and patients with a negative whole‐body scan for preoperative evaluation and staging [46]. The integration of SPECT and CT, hybrid SPECT/CT, provides more precise information in comparison with conventional planar imaging and leads to an improvement in imaging interpretation and staging of the disease [42]. SPECT/CT has an incremental role in the staging and treatment response evaluation, being able to differentiate the sites of radioiodine pathologic uptake after the administration of radioiodine in both diagnostic whole‐body scans and post‐therapeutic whole‐body scans (e.g. the uptake in the parotid gland versus metastatic latero‐cervical lymph nodes). SPECT/CT has been shown to improve the specificity of the imaging by providing both functional and anatomical data and accurate attenuation correction [47]. Figure 16.9 demonstrates the essential role of SPECT/CT in the follow‐up of DTC: the 131I whole‐body scans presented in Figure 16.9a,b show pathologic uptake of radioiodine within the lungs and a focal uptake in the dorsal spine (black arrow), although it is impossible to determine the exact location in which dorsal vertebra (a). In the posterior–anterior view (b) there is also a faint uptake in the skull that might be easily omitted or misinterpreted as focal nasal uptake (red arrow). The SPECT/CT images presented in Figure 16.9c,d show that the pathologic cranial uptake is related to an occipital metastatic osteolytic lesion. The same hybrid image was obtained for the spine, demonstrating a metastatic lesion in the 12th dorsal vertebra, confirmed by biopsy as metastases from papillary thyroid carcinoma. Figure 16.6 An 18‐year‐old female with papillary thyroid cancer. 123I whole‐body scan was performed following thyroidectomy. Anterior and posterior Whole body scintigraphy (a, b) show residual uptake in the thyroidectomy bed, bilateral nodal uptake in the neck. and diffuse uptake in the lungs consistent with metastatic involvement. The chest CT (c, d) demonstrates very small scattered pulmonary nodules, better seen in the maximum intensity projection (d). Figure 16.7 Axial image of the chest of 18F‐FDG PET/CT in a 62‐year‐old man with follicular thyroid carcinoma radically treated by surgery and radioiodine therapy. The hybrid image PET/CT (a) shows sternal and rib metastatic lesions, despite the negative 131I whole‐body scan (b). Figure 16.8 18F‐FDG PET/CT in a 90‐year‐old female with newly diagnosed anaplastic thyroid carcinoma. Intense uptake is present in the thyroid mass, which invades the right tracheal wall (axial cervical CT image, upper left image) as well as metastatic lymph nodes (white arrow lower left image of PET/CT axial view) and multiple lung metastases (black arrowhead, MIP coronal image). Figure 16.9 Post‐therapeutic 131I whole‐body scans (a, antero‐posterior view; b, postero‐anterior view) in a case of aggressive papillary thyroid carcinoma showing pathologic radioiodine uptake in the lungs and dorsal spine (black arrow), and faint uptake in the skull. The CT sagittal view (c) and the fused sagittal SPECT/CT image (d) show that the pathologic cranial uptake is related to a skull metastatic osteolytic lesion (red arrows). PET/MRI of the thyroid has been reported to be more advantageous than PET/CT in the detection of iodine‐positive lesions. However, it has not been shown to be superior to PET/CT in differentiation of the lesions [48]. Other advantages of PET/MRI over PET/CT include its detailed soft tissue demonstration and absence of ionizing radiation. Reducing the ionizing radiation can be of great importance in patients who undergo frequent evaluations [49] or in pediatric patients. Parathyroid glands can have diverse shapes, location, size, or number among different individuals. Approximately, 80–97% of the population have four parathyroid glands and about 19% have fewer or supernumerary glands [50, 51]. Up to 16% of these glands are ectopically positioned in the neck and mediastinum [51]. The superior glands originate from the fourth pharyngeal pouch and are usually located at the cricothyroid junction. The inferior glands, however, are derived from the third pharyngeal pouch and can have more variable locations, but are frequently found at the posterolateral or anterolateral side of the lower end of the thyroid gland. These glands may have ectopic locations such as the mediastinum and thymus. They are normally ovoid‐shaped and their size varies from 4 to 6 mm in length and 2 to 4 mm in width. The inferior thyroid artery usually maintains the blood supply to the parathyroid glands. However, in a small percentage of the population, the superior thyroid artery contributes to supplying blood to the superior parathyroid glands [51–53]. Primary hyperparathyroidism is diagnosed based on biochemical testing. Imaging has a role in localizing pathology in patients who are considered for surgical management. Parathyroid carcinoma is rare and may be incidentally detected in the workup of hyperparathyroidism or present with neck mass of metastatic disease to lungs, cervical lymph nodes, liver, or bone. Carcinoma should be suspected in patients presenting with parathyroid crisis. Ultrasonography is useful for assessment of parathyroid pathologies and biopsy guidance but may be limited if the pathology is retropharyngeal, retroesophageal, or mediastinal. The combination of nuclear scintigraphy and ultrasound can be very helpful in preoperative localization [54–56]. More false‐positive results can be seen with ultrasound than scintigraphy [57]. Contrast‐enhanced CT has low sensitivity in the detection of parathyroid diseases. Some studies have reported MRI to have a sensitivity of up to 88%, which is considered to be as high as that of 99mTc‐sestamibi scintigraphy [53, 54, 58]. However, the signal characteristics of parathyroid adenoma and cervical lymph nodes overlap and MRI is not usually considered the first modality for preoperative detection of the parathyroid lesions [59]. In some institutions, multiphase CT (4D‐CT) is used even as the first‐line modality and is reported to be superior to other conventional imaging modalities [55, 60]. When combined with ultrasound, the sensitivity of this modality in localization of the parathyroid adenoma can be up to 94% [61]. Normal parathyroid is not expected to be visible on ultrasound imaging and is not distinguishable from adjacent tissues. However, parathyroid adenoma or hyperplasia typically appear as enlarged ovoid and hypoechoic lesions in comparison with the thyroid gland, which can be attributed to their lipid‐rich and solid structure [62, 63]. There is also a distinct and hyperechoic capsule surrounding the gland that can be indiscernible because of its low thickness. Anechoic segments with posterior acoustic enhancement or echogenicity analogous to the thyroid are interpreted as cystic degeneration, which can develop in some parathyroid lesions [55, 62, 64]. On the other hand, segments of enhanced echogenicity within the glands should be interpreted as fibrosis, calcification, adipose tissue, or hemorrhage. The “polar vessel sign” can be used for distinguishing lymph nodes from the parathyroid glands. This refers to the presence of an engorged vessel connected to the pole of the ovoid‐shaped parathyroid gland on power Doppler sonography [55, 62, 64, 65]. Another feature that can be used for differentiation of the lymph nodes is their hyperechoic, fatty hilum on ultrasound imaging, which is not present in parathyroid glands [62]. 4D‐CT has higher sensitivity compared to ultrasound and sestamibi scanning and outperforms them in precise localization of the gland in the accurate quadrant (70% vs. 30%) [66]. An enlarged or adenomatous parathyroid appears as a soft tissue nodule with lobulated margins, which has the maximum enhancement during the arterial phase. The contrast material washes out gradually over the course of imaging. This finding is used to discriminate these glands from lymph nodes since they increasingly enhance throughout the imaging. Similar to the ultrasound imaging, on 4D‐CT cystic degeneration within the gland and the polar vessel sign also favors this diagnosis [55, 67]. On noncontrast‐enhanced CT images, parathyroid adenomas have low attenuation [67]. On T1‐weighted images, adenomatous and hyperplastic pathologies appear as low/intermediate intensity lesions. However, on T2‐weighted images, they manifest as high‐signal lesions that have a fairly homogenous or marbled manifestation (approximately 65%) [59]. The signal intensity decreases in the presence of fibrosis and old hemorrhage, and increases with recent hemorrhage on T1 and T2 sequences [55]. A marbled appearance on T2‐weighted images can be attributable to hemorrhage, fibrosis, and cholesterol clefts, which are usually present in large adenomas. Overall, parathyroid adenomas tend to appear as hyperintensity (relative to the thyroid gland) on T2‐weighted images and have elongated morphology. They also have a higher enhancement relative to the thyroid, and earlier and more intense enhancement in comparison with the lymph nodes [59]. The first‐line modality for the evaluation of primary hyperparathyroidism is parathyroid scintigraphy, performed either with single (99mTc‐MIBI) or dual (99mTc‐pertechentat and 99mTc‐MIBI) tracer protocol in one day with early and delay acquisition or through a two‐day protocol. 99mTc‐sestamibi (99mTc) is the primary radiotracer utilized in scintigraphy of the parathyroid glands. 99mTc‐tetrofosmin can also be used for dual‐isotope parathyroid imaging. However, unlike sestamibi, there is no differential washout between thyroid and parathyroid tissue. 99mTc scintigraphy can be used for evaluation of a variety of parathyroid pathologies, including eutopic disease, ectopic glands, solitary, double, or multiple adenomas, multiple endocrine neoplasia (MEN), lipoadenoma, hyperfunctioning parathyroid, etc. However, this modality is mainly used for preoperative assessment of persistent or recurrent hyperparathyroidism as well as presurgical exploration [53]. Dual‐isotope, single‐phase or dual‐phase, or single‐isotope scintigraphy studies can be performed in parathyroid imaging. SPECT imaging has shown some advantages compared to planar and is nowadays used in many institutions [68, 69]. Primary hyperthyroidism (PHPT) is a clinical diagnosis that refers to the overproduction of the parathyroid hormone (PTH) which affects the normal homeostasis of calcium. This condition may develop as a result of parathyroid adenoma (most common), hyperplasia of the gland, and carcinoma (least common, <1%) and its treatment is parathyroidectomy [70]. Imaging is usually performed in cases with a confirmed clinical diagnosis for accurate localization of the pathology before surgery. Presurgical localization of the pathologic gland is performed to strategize the least invasive approach for the parathyroidectomy and avoid aggressive bilateral neck exploration [71]. Parathyroid 99mTc‐sestamibi scintigraphy, as the currently preferred technique, with a sensitivity of up to 88%, can help with more precise localization of the lesion, especially ectopic glands in the mediastinum, and can be even more informative when combined with grayscale CT (SPECT‐CT) [55, 71] (Figures 16.10 and 16.11). Enhanced focal uptake and extended retention of 99mTc‐sestamibi can both indicate abnormality in the parathyroid glands. In other words, in the early phase, the parathyroid gland appears as a focal uptake on a normal uptake background. In the delayed phase, there is a more significant reduction in the background tissue uptake compared to the hyperfunctioning glands [55]. Figure 16.12 shows macroadenoma of the inferior right parathyroid gland occurring in a patient with breast cancer, severe hypercalcemia Ca (14.2 mg/dL) and PTH (323.4 pg/mL). The surgical specimen confirmed the diagnostic of parathyroid adenoma. PET and PET/CT have been introduced for parathyroid imaging purposes. 11C‐methionine and 18F‐fluorocholine are common radiopharmaceuticals used in hybrid imaging of the parathyroid [55]. 11C‐methionine PET has lower sensitivity compared to standard 99mTc‐MIBI scintigraphy for the detection of the pathologies adjacent to the thyroid gland [55, 72] and is less used due to the characteristics of 11C, which has a very short half‐life (20.4 minutes). In contrast, 18F‐fluorocholine PET/CT and PET/MR have higher accuracy compared to ultrasound, SPECT, or MRI for localizing parathyroid pathology [57, 73]. A detection rate of over 90% has been reported [74]. Using nuclear imaging allows the surgeon to perform radioguided surgery, a minimally invasive and low‐risk procedure. Figure 16.10 SPECT‐CT of the parathyroid. The planar MIBI scintigraphy of a 61‐year‐old patient with primary hyperparathyroidism and concomitant multinodular goiter at 20 minutes (center column of upper row) and 120 minutes (right column of upper row), showing positive activity in the right lower lobe of the thyroid and equivocal activity in the left lower lobe of the thyroid. The SPECT views (middle row) show localization of the parathyroid adenoma in the posterior of the lower right lobe of the thyroid. The SPECT‐CT imaging in the lower row shows the precise localization of the parathyroid adenoma on the right side of the tracheoesophageal groove. The left column of the upper row is a CT image of the same patient. In surgery, an 8.5 mm in diameter adenoma on the right side of the tracheoesophageal groove was excised. The left lower activity on planar views was a thyroid nodule. The pituitary gland is sited inside the sella turcica within the sphenoid bone. Cavernous sinus forms the lateral walls. This space contains cranial nerves 3, 4, and 6, and the first and second branches of the trigeminal nerve. The pituitary gland is linked to the hypothalamus through the pituitary stalk, which is located adjacent to the anterior portion of the third ventricle [75]. The posterior lobe is partially covered by the anterior lobe, and the pars intermedia, which is considered as an intermediate lobe, is located between the two of them. Nonfunctional Rathke’s cysts tend to develop at this site [75, 76]. The average height of the pituitary gland is reported to be between 3 and 8 mm [75]. Less than 4 mm is considered small, but this is not always pathologic and can be related to small sella turcica and advanced age. This finding is commonly seen in primary hypopituitarism [77]. The height of the gland can be larger in females (especially during the sixth decade of life), during adolescence, and most significantly during pregnancy (up to 10 mm in height) [75]. Impinging effects on the chiasm and intracranial hypotension are two recognized conditions associated with an enlarged pituitary gland [77]. Figure 16.11 Parathyroid adenoma with brown tumor. A 37‐year‐old woman presented with left shoulder pain and a history of left arm fracture due to a minor trauma 2 months ago. Whole‐body bone scan (a) shows multiple foci of 99mTc‐MDP uptake throughout the skeleton and prominently in the skull. The X‐ray of the skull (b, c) shows a salt and pepper appearance, especially in the parietal and temporal bones. Based on the parathyroid and thyroid scans, laboratory tests, and radiological findings, the best diagnosis was primary hyperparathyroidism due to parathyroid adenoma. The diagnosis of parathyroid adenoma was confirmed after surgery. Figure 16.12 99mTc‐MIBI SPECT/CT, coronal, sagittal, and axial views (a, CT; b, nuclear SPECT; c, fused SPECT/CT), showing a macroadenoma of the right inferior parathyroid (red arrow) confirmed by the surgical specimen (d). In pregnant women and neonates, the pituitary gland appears hyperintense on T1‐weighted sequences. However, about 6 weeks (up to 3 months) after birth, on T1‐weighted MRI, the anterior pituitary gland demonstrates a signal similar to brain tissue and the posterior pituitary preserves the bright signal [75, 77], which is brighter than other parts of the gland [78] (known as the posterior pituitary bright spot). The posterior pituitary bright spot sign is lost in the majority of patients with diabetes insipidus [79, 80]. MRI is often the first‐line and most vital imaging modality in pituitary evaluation due to its sophisticated soft tissue depiction. The MRI sections used for this purpose should be thinner than 2 mm, with no intervening gap. Thin T1‐weighted sequences before and after gadolinium contrast enhancement, in the coronal and sagittal planes, are considered a reliable technique for the detection of pituitary lesions and assessment of their size, shape, signal homogeneity, and adjacent structures. However, small lesions may not be easily detected using rudimentary MRI techniques. T2‐weighted images may be useful in some cases for additional evaluation [75, 81]. Some other MRI sequences have been introduced as an alternative for T1 and T2, but their extensive clinical usage is still limited [82–84]. Sophisticated MRI technology is helpful in the detection of small lesions and ruling out the differential diagnosis in large ones by yielding an elevated signal‐to‐noise ratio [85, 86]. Contrast‐enhanced MRI should be obtained immediately after the bolus contrast administration. Normal tissues usually enhance before the tumoral tissues and this can be used as a helpful diagnostic criterion. On noncontrast MRI in T1 and T2 standard spin‐echo images, the normal anterior pituitary gland appears as an isointense/gray structure. The normal posterior pituitary gland appears as a high signal in the T1 sequence and hypointense on T2‐weighted images. Dynamic MRI as a broadly acceptable technique is helpful in cases in which the differentiation of the pathology from normal tissue is difficult on contrast‐enhanced T1 sequences [87, 88]. Pituitary adenomas enhance slower than normal pituitary tissue in 70–90% of cases and are hypointense on dynamic T1 contrast‐enhanced sequences [89]. Although MRI is the first‐line modality of pituitary imaging, CT may be more precise in the detection of calcification and bone details. Also, in cases where MRI is contraindicated, or the patient suffers from claustrophobia, CT is indicated [77, 86]. The CT images should be obtained without contrast and immediately postcontrast, and the thickness of the axial planes should be 0.625–1.3 mm along with coronal and sagittal reformation [77]. The application of molecular imaging for evaluation of pituitary abnormalities is very limited due to the inability of these modalities to visualize pituitary adenomas. 18F‐FDG PET/CT, PET/MRI, dopamine imaging, and somatostatin receptor imaging (68Ga‐SSA PET or SSA scintigraphy) are useful in a small number of conditions, including characterization of pituitary lesions to aid with differential diagnosis, detection, and localization of ectopic pituitary hormone production, or in metastatic disease [90]. Somatostatin receptor scintigraphy has been introduced in cases of recurrent pituitary tumors for differentiation of the tumor tissue from scar tissue [91]. PET has been recommended for the identification of germ cell tumors and their differentiation from hypophysitis [92, 93]. Pituitary adenomas are the most common cause of incidental intense focal uptake in the pituitary gland seen on 18F‐FDG PET for unrelated indications. There is no actual indication for 18F‐FDG PET/CT in the evaluation of primary hypophysis pathology. However, 18F‐FDG PET/CT might discover in hypophysis an incidental high uptake during the scan for other malignancies; incidental abnormal F18‐FDG uptake may represent primary malignant or benign tumors, physiological uptake or metastases of a previously known cancer, which might occur in <0.04% of PET/CT scans [94] (Figure 16.13). 18
16
Correlative Imaging in Endocrine Diseases
Thyroid Imaging
Thyroid Anatomy and Approach to Thyroid Nodules
Ultrasound Imaging and FNA Biopsy
Thyroid Scintigraphy
99mTc SPECT/US Fusion Imaging
Pentavalent 99mTc‐DMSA or 99mTc‐MIBI Scintigraphy
The Role of 99mTc‐MIBI in the Differentiation of Amiodarone‐Induced Thyroid Disease (Type I, II, or III)
PET and Hybrid Imaging PET/CT or PET/MRI
124I‐PET/US Fusion Imaging
CT and MRI in Evaluation of the Thyroid Pathologies
DTC and Use of Hybrid Molecular Imaging
Parathyroid Imaging
Overview of the Embryology and Anatomy of Parathyroid Glands
Overview of the Imaging Modalities used for Evaluation of Parathyroid
Correlative Imaging Approach in the Detection of Parathyroid Lesions
Ultrasound
4D‐CT
MRI
99mTc‐sestamibi Planar Scintigraphy and SPECT
PET in the Evaluation of the Parathyroid Glands
Pituitary Imaging
Normal Anatomy of the Pituitary Gland
Role of Various Imaging Modalities in the Evaluation of Pituitary Pathology
Stay updated, free articles. Join our Telegram channel

Full access? Get Clinical Tree
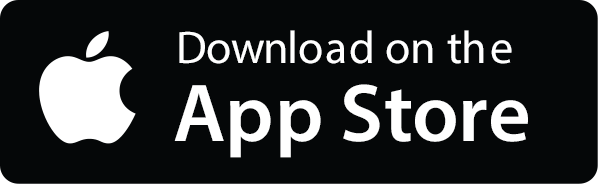
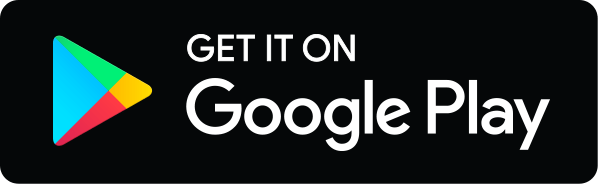