Slice thickness
1 mm
Interval
10 mm (in premature infants we may use 5 mm)
15–20 mm (in follow-up controls)
kVp
<30 kg 90
≥30 kg 100–120
mAs
<30 kg 25–40
≥30 kg 25
Seconds
0.6–1.0
FOV
15–40
Filter
High-spatial-frequency algorithm (bone)
Use standard for mediastinum
When examining children, the potential size effects of radiation exposure should always be kept in mind (Lucaya et al. 2000). With use of data obtained in A-bomb survivors, it has been predicted that delivery of 1 Rad (0.01 Gy) of radiation to a woman breast before the age of 35 fractionally increases her risk of breast cancer by 13.6 % over the expected spontaneous rate for the general population. (Land et al. 1993). This is one of the main reasons why we strongly support the use of low-dose techniques for children. Combining HRCT scans at 20 mm intervals with low-dose scans (90 kV and 40 mAs) would result in an average skin dose comparable to that associated with chest radiography (Mayo et al. 1993). Moreover, with properly performed HRCT, one can manage to study the lung with even less radiation to the female breast than with conventional radiography. When examining children with HRCT, we try to either skip the area around the nipple or use shielding. Selective breast shielding has been recommended to achieve significant radiation protection (Fricke et al. 2003). It is particularly useful when performing standard HRCT studies and does not significantly affect image quality (Fig. 1). The use of bismuth shields when performing volumetric exams has been recently questioned. Proponents (Kim et al. 2010) point to significant dose reduction to the breast, whereas opponents (Geleijns et al. 2010) argue that the shields may be counterproductive (McCollough et al. 2012b).
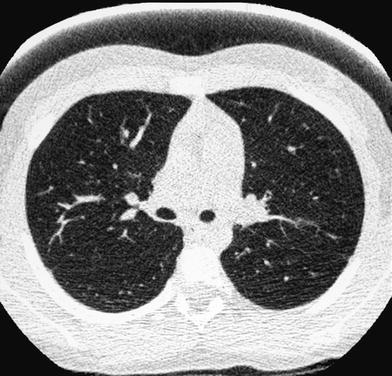
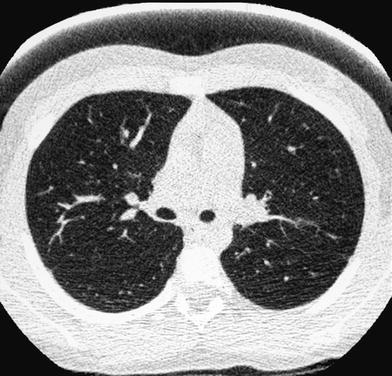
Fig. 1
A 10-year-old girl. HRCT of the lungs performed using a 1 mm-thick bismuth-coated latex shield over both nipples. Notice there are no significant artifacts
In children under 8 years of age, the mean attenuation value of normal lung ranges from −500 to −700 HU and in those 8 years or older it is about −800 HU, which is similar to the attenuation value in healthy adults (−700 to −800 HU). It should be emphasized that there are no “correct” or ideal windows settings for demonstrating lung anatomy, to be used when photographing an HRCT study. A wide window setting (width of 1500 HU at a center of −500 HU) is recommended for optimally displaying lung parenchyma (Klusmann and Owens 2009). Often the precise windows width and levels chosen are a matter of personal preference. However, it is important that at least one lung window setting be used consistently in all patients. If this is not done, it is difficult to develop an understanding of what appearances are normal and abnormal, in order to compare cases and to compare sequential examinations in the same patient. Level and width settings of 50/350 are best for evaluating the mediastinum and hila (Webb et al. 1996). The recommended scanning parameters for HRCT of the chest in children are shown in Table 1.
Since the diagnostic sensitivity and specificity of HRCT of the lungs are superior to those of conventional chest X-rays, the indications for HRCT in children have been increasing. Therefore, we should ensure that the examination is the least aggressive possible. For this purpose, scans should be tailored to the specific clinical problem, the number of sections and exposure parameters should be decreased as much as possible, low-dose techniques should be used routinely and scout views spared. To tailor the examination to diagnostic needs, the radiologist should know the patient’s clinical features and previous imaging findings. Furthermore, to obtain the greatest diagnostic information that HRCT can provide, the radiologist should directly supervise the study and decide whether additional or special slices (prone, lateral, decubitus, expiratory, etc.) are required. This approach avoids unnecessary examinations and virtually eliminates incomplete studies. Table 2 shows the main indications of HRCT in children.
Table 2
HRCT: Indications
Screening of patients with repeated respiratory infections |
Bronchiectasis |
Cystic fibrosis |
Bronchopulmonary dysplasia |
Severe asthma |
Bronchiolitis obliterans |
Diffuse pulmonary disease |
Control of some malformations |
Recent developments in radiation dose reduction strategies, which include advances in data acquisition, image reconstruction, and postprocessing algorithms, hold promise for reducing volumetric CT dose (McCollough et al. 2012a; Dougeni et al. 2012). Initial reports suggest that iterative reconstruction may reduce noise and enable substantial reductions in radiation exposure of up to 30–50 % (Sodickson 2012).
The potential for further dose reduction in CT is evident. However, these advances are not universally available on all scanner systems. Moreover, modern multidetector CT scanners made by the various manufacturers differ from one another on essential points (e.g., scanner geometry, detector design, and dose efficiency and collimation technique). This makes comparison between scanners and protocols almost impossible. (Nievelstein et al. 2010). The complexity of new CT scanners adds new challenges. Therefore, substantial research should be focused on this line in the coming years.
Noncontiguous HRCT of the chest is a valuable low-dose tool used to provide information about the pattern and anatomical distribution of lung pathology, determine lung response following therapy, and guide clinical management.
Needless to say, the routine use of low-dose techniques is mandatory to minimize the potential side effects of ionizing radiation exposure. This is extremely important for extending the indications in children, so they can benefit from the excellent diagnostic information it provides.
3 Sedation
Another important measure aimed at reducing the aggressiveness of HRCT is to avoid anesthesia and use of sedation as little as possible. In our practice, we have never used general anesthesia for HRCT in children. Up to 2008 we had reduced the use of sedation in children less than 6 years of age to merely 1.5 %. Since 2008 none of the 1600 HRCT performed have required sedation. We resort to all sorts of tricks to keep the nonsedated patients still. We make sure they are warm and allow the parents to hold their hands and talk to them. To attract a fidgety child’s attention, we project and move a spot of light, known TV cartoons onto the gantry or play music throw the gantry speakers. It is also helpful to offer them a bottle with glucose water.
In the past, we used to keep our patients NPO (nothing in mouth) for 4 h before the exam in children younger than 1 year and for 6 h in those 1 year or older because of aspiration risk related to sedation. Nowadays, none of our patients are kept NPO and those who, once in the gantry, behave poorly are rescheduled. Since well-fed infants behave better than hungry ones, this policy has been helpful in reducing our sedation rate to zero. However, as sedation or anesthesia may be needed in hospitals where CT equipment is not as fast as ours or where the technical team is not used to dealing with children, we are including our previous sedation protocols.
We used chloral hydrate p.o. at a dose of 50–75 mg/kg, with a maximum dosage of 2000 mg. Children are given an initial dose of 50 mg/kg and are kept in the sedation area. If after 20–30 min the patient has not fallen asleep, a second dose of chloral hydrate, usually half the initial dose (25 mg/kg), is given. Exceptionally, we may go up to a total dose of 100 mg/kg. The onset of action is usually within 25–30 min and the duration of sedation is 30–40 min. Chloral hydrate has a bitter taste that children dislike. Attempts to conceal it with sweeteners like cherry syrup are not very helpful. Although the taste improves somewhat, it still remains unpleasant. Furthermore, the total volume of fluid to be administered increases and dose control becomes difficult. Consequently, we always use undiluted chloral hydrate administered directly by syringe or with a nipple connected to a syringe. With time and patience most children swallow it well. Although we use chloral hydrate mainly in children under 2 years of age, it can also be use in older patients.
Chloral is successful sedative in 95–99 % of children (Karian et al. 1999; Pererira et al. 1993) and has a very low rate of side effects. Transient respiratory depression (oxygen desaturation 10 % below baseline for a patient for more than 15 s, despite repositioning of the head and neck to clear airway) is the most common during or after sedation, yet it occurs in less than 1 % of patients. Delayed complications such as vomiting, irritability, and mild respiratory difficulty are also rare (Egelhoff et al. 1997).
Many different models are used in different institutions to provide effective, safe sedation (Chung et al. 2000; Rooks et al. 2003; Shankar 2008; Macias and Chumpitazi 2011; Mason et al. 2011; Henry et al. 2012; Heng Vong et al. 2012). Pentobarbital and chloral hydrate remain the most widely used sedatives for CT. Use of multidrug sedation regimens should be avoided (Sanborn et al. 2005). The radiologist cannot be responsible for sedation. The person providing sedation and monitoring the patient should have proper training to assure patient safety and should not be the person responsible for the imaging procedure (Shankar 2008). All sedated patients are given oxygen by mask or nasal prong to increase pulmonary oxygen reserves and permit prolonged apnea or airway obstruction without hypoxia. Oxygen should be administered to all patients receiving sedative medications with the possible exception of neonates at risk of retinopathy of prematurity, in which case a neonatologist should be consulted. There are no rules about the amount of supplemental oxygen that a patient requires; rather, administration of any amount improves the margin of safety. Thus, there is no legitimate reason to not administer oxygen routinely when patients are sedated (Fisher 1990). Continuous monitoring of the vital signs (at least every 5 min) must be performed and recorded during each use of sedation.
The physiologic measurements, we monitor include oxygenation (with pulse oximetry), heart rate, respiratory rate, and temperature. The alarm on the pulse oximeter is usually set at 90 % oxygen saturation, but any decrease below 95 % is immediately investigated. The majority of apparent desaturations are due to patient motion and loss of sensor contact. A small number of patients, however, demonstrate significant decreases in pO2. Most of this are transient and are quickly corrected by repositioning the head and extended the neck. Occasionally, a patient requires suctioning of the oral cavity. A suction device and size-appropriate recovery equipment must be on hand during each sedation procedure. Children who have medical conditions that compromise the airway require special attention with respect to cardiopulmonary monitoring and airway management. These children may not be appropriate candidates for sedation by personnel who do not routinely deal with pediatric airway management and cardiopulmonary resuscitation. Children who fall into this monitoring category include those with anatomic airway anomalies (craniofacial defects), those with airways disease such as obstructive adenotonsillar hyperplasia, acute respiratory infection, uncontrolled asthma, and those with significant cardiopulmonary, neurologic and hepatorenal disorders. Life-threatening airway obstruction or respiratory depression with hypoxia can occur in these children (Vade et al. 1995). Certain patients are difficult to sedate, such as children with mental retardation, patients receiving chemotherapy or antiseizure medication and those habituated to sedation (Hubbard et al. 1992).
Once the examination is over, all sedated patients are discharged home or transported to the inpatient wards, when they meet the postanesthesia care unit discharge criteria recommended by the American Academy of Pediatrics (American Academy of Pediatrics Committee on Drugs 1992):
1.
Cardiovascular function and airway patency are satisfactory and stable.
2.
The patient is easily arousable, and protective reflexes are intact.
3.
The patient can talk (if age-appropriated).
4.
The patient can sit unaided (if age-appropriated).
5.
The state of hydratation is adequate.
6.
For a very young or handicapped child, incapable of expected responses, the presedation level of responsiveness or a level as close as possible to the normal level for that child should be achieved.
Parents are instructed not to feed the children until their level of consciousness and motor function have returned to presedation ranges. When examining critically ill patients, we require the assistance of a pediatrician from the intensive care unit. After the examination is completed, these patients are returned to their wards immediately under the supervision of the specialist. Other sedation regimes (see chapter on Helical CT) are practically never required for HRCT.
4 Special Techniques
4.1 “Focused” Chest CT
In patients with known “localized” lung disorders we recommend a “focused” technique, performing 1 mm slices at 10 mm intervals through the abnormal area of the lung. The rest of the lung is not scanned. We believe that study of the entire lung should not be performed in patients being controlled for known localized disease whose clinical symptoms and/or chest radiographs do not suggest progression to other lobes. The “focused” scan is used in the following-up of bronchiectasis, right middle lobe syndrome, cystic emphysema (Fig. 2), cavitated pneumonia, and some pulmonary malformations not considered tributary of surgical treatment. In many of these cases, three or four low-dose HRCT slices will provide more information with less radiation dose to the female breast than PA and lateral radiographs. As always with HRCT, we try to skip the scout of view to save on radiation exposure, though occasionally, and particularly when we want to reduce the exam to a mere of two or three slices, we may use it. We center the exam with the light collimator. When we want to explore the right middle lobe, lingular segments, and both lower lobes, we start the study midway between the sternal manubrium and the xifoid. When examining the upper lobes, we start the study at the level of the clavicles and stop at the inferior border of the abnormal lobe.
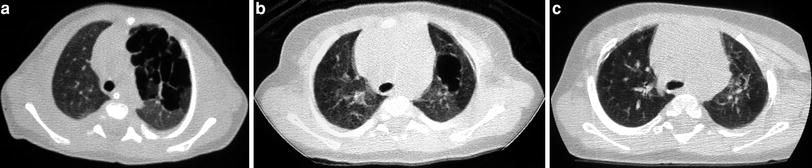
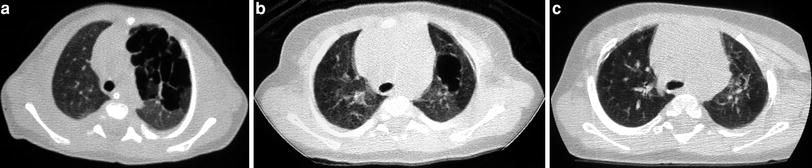
Fig. 2
A 2-week-old premature baby with RDS treated with mechanical ventilation developed localized pulmonary emphysema in left upper lobe. (a) Three months later (b) the lesions have decreased in size. At the age of 10 months (c) CT is normal
4.2 “Limited Slices” Chest CT
The “limited slices” technique, consisting of 1 mm slices at 20 mm intervals, is a type of “sampling” technique that can be used for studying generalized lung disorders. Radiation dose is halved with this technique, making it particularly useful for follow-up of patients with chronic lung disorders who require repeated examinations. The main indication for limited slice is in the control of patients with cystic fibrosis, bronchopulmonary dysplasia (Fig. 3), histiocytosis X, alveolar proteinosis, and interstitial pneumonias.
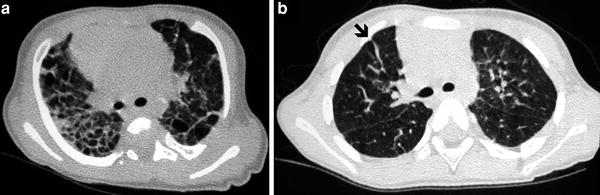
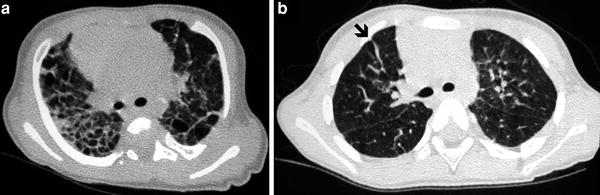
Fig. 3
Bronchopulmonary dysplasia at the age of 2 months (a) shows marked septal thickening, parenchymal bands (arrow), and multiple hyperlucent areas. Repeated HRCT at the age of 2 years (b) shows a mosaic pattern and some residual parenchymal bands (arrow)
In our experience, use of both “focused” and “limited-slice” techniques has increased steadily over the last few years, particularly when examining female patients with chronic lung disorders. In addition to provide reliable diagnostic information, these techniques permit a reduction in radiation exposure to the breasts. If radiographs are still required in this group of patients, we obtain the AP or PA views only. The lateral projection is not routinely performed.
4.3 Expiratory Slices: Lateral Decubitus and Prone Views
Expiratory slices are extremely helpful when examining patients suspected of having airway abnormalities or patients with a history of repeated pulmonary infections who are found to have a normal o questionably normal inspiratory CT exam (Lucaya et al. 1999) (Fig. 4). They are also useful when the inspiratory sections demonstrate a mosaic pattern, characterized by visible differences in lung attenuation. Since children do not usually suffer pulmonary thromboembolic disorders, a mosaic pattern is almost always due to small airway disease with obstruction.
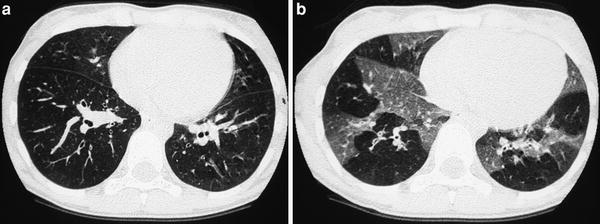
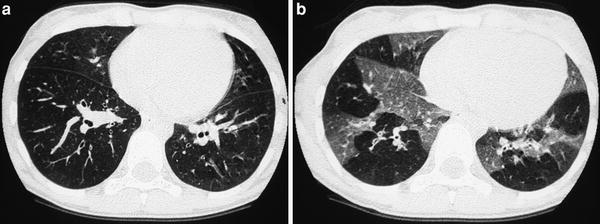
Fig. 4
A 9-year-old boy with severe asthma. Inspiratory scan (a) shows questionable mosaic pattern, which is evident on expiratory scan (b)
When the examining child presents clinical or radiological features commonly associated with small airway disease, we routinely complete the HRCT examination with three additional expiratory slices at three equally-spaced levels, one in the upper, one in the middle, and one in the lower lobes. We use the table level information provided by the inspiratory exam to center these slices. Whereas the “level” of the upper lobes does not change significantly on expiration, the middle lobe, lingular segments, and particularly the lower lobes will “move upwards” significantly, from 2 to 5 cm, depending on the size of the patient. To obtain good expiratory scans it is mandatory to spend some time teaching the child how to exhale well.
A useful method for obtaining expiratory scans in uncooperative children is to use the lateral decubitus technique (Capitanio and Kirkpatrick 1972; Lucaya et al. 1999; Choi et al. 2002). The patients are scanned in both lateral decubitus positions. With the child on his side, the dependent hemithorax is splinted and movement of the thoracic cage is restricted on that side. When movement of the hemithorax is limited, the lung on the dependent side tends to be underaerated. Conversely, the hemithorax facing upwards is not restricted and the lung is well aerated. If air trapping is present, either diffusely or patchy, the affected lung lobe or segment will remain hyperlucent when that side of the thorax is in the dependent position (Fig. 5). occasionally, in noncooperative patients with normal chest X-rays and known diseases, such as cystic fibrosis or severe asthma, in whom the initial HRCT abnormality is usually of a mosaic pattern secondary to air trapping, we will limit their initial HRCT exam to three scans in each lateral decubitus position.
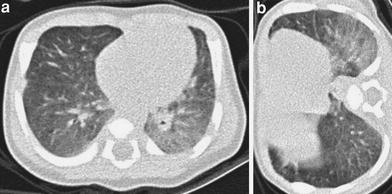
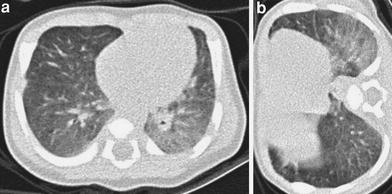
Fig. 5
HRCT in a 23-day-old baby with meconial aspiration and sepsis. Supine scan (a) shows a ground-glass opacity in the left lower lobe, which persists in the right lateral decubitus scan (b)
This simple technique can also be used when trying to obtain good inspiratory examinations in noncooperative patients. As mentioned, the lung facing upwards is usually well aerated. Awareness of this fact is particularly helpful when examining noncooperative patients whose supine scans show a ground-glass pattern consistent either with lung disease or with normal lung on expiration. When the lungs are normal, the ground-glass pattern will no longer persist in the lung facing upwards (Fig. 6). This same principle of gravity-dependent aeration is the rationale for using prone views to obtain good inspiratory scans of the lower lobes (Figs. 7, 17).
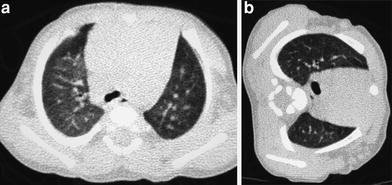
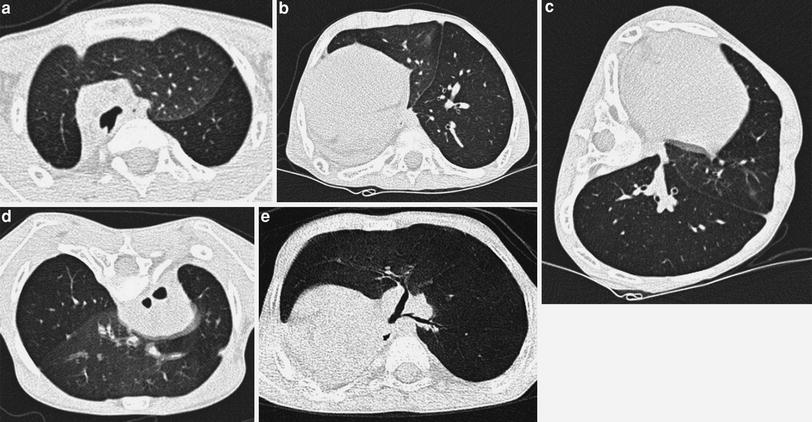
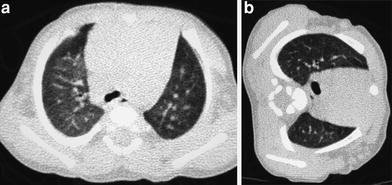
Fig. 6
A 4-month-old infant with leukemia, fever, and questionable pneumonia. Supine HRCT (a) shows ground glass in right upper lobe, which is no longer identified in the left lateral decubitus view (b)
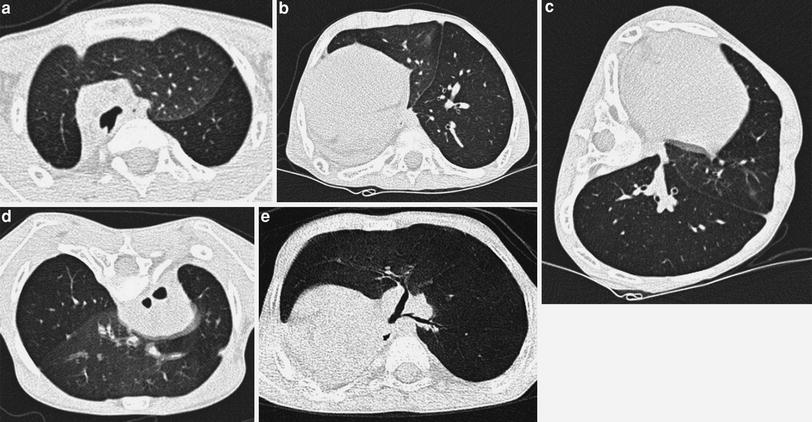
Fig. 7
A 6-year-old boy with charge syndrome who underwent right pneumonectomy due to repeated infections and bronchiectasis, with progressive respiratory difficulties. HRCT in supine decubitus position shows left lung herniation to the contralateral hemithorax and left lower lobe air trapping (a, b) which is confirmed in the lateral (c) and prone (d) views. Volumetric CT (e) shows stenosis of the lower lobe bronchus
5 Anatomy
The lung is supported by a network of connective tissue fibers known as the lung interstitium (Fig. 8). For the purpose of interpreting HRCT images and identifying abnormal findings, the interstitium can be thought of as having several components. The peribronchovascular interstitium is a system of fibers that invests the large bronchi and pulmonary arteries in the parahilar regions and forms a continuum with the centrilobular interstitium, surrounding the small centrilobular bronchi, arteries, and lymphatic vessels. The subpleural interstitium is located beneath the visceral pleura and envelops the lung in a fibrous sac from which connective tissue septa (interlobular septa) invaginate into the lung parenchyma. The pulmonary veins and lymphatic vessels travel in the interlobular septa. The last component is the intralobular interstitium, a network of thin fibers in the walls of the alveoli bridging the gap between the centrilobular interstitium and the interlobular septa o subpleural interstitium (Weibel 1979).
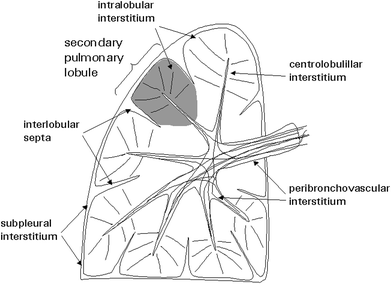
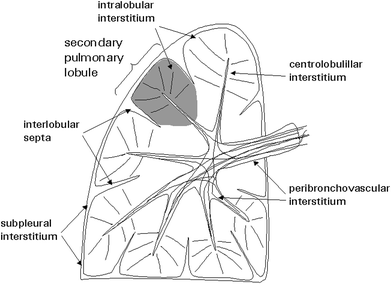
Fig. 8
Components of the lung interstitium and secondary pulmonary lobule. [Modified and reprinted with permission from Webb et al. (1996)]
The secondary pulmonary lobule is the smallest lung unit delineated by connective tissue septa and the smallest functional unit that can be directly visualized by HRCT (Fig. 9). With a diameter of 1–2.5 mm, it can have polyhedric or prismatic shape, but more frequently resembles a truncated pyramid. Each secondary lobule has a central supporting tissue (centrilobular interstitium) containing a small bronchiole, pulmonary artery, and lymphatic vessel (bronchovascular bundle) and is marginated by interlobular septa that contains pulmonary veins and lymphatic branches. The substance of the secondary lobule, surrounding the lobular core and contained within the interlobular septa, consists of a variable number of lung acinis (ranging from 3 to 24) and the associated capillary bed, supplied by small airways and branches of the pulmonary arteries and veins (Giovagnorio and Cavallo 1995). Secondary lobules are difficult to visualize in HRCT scans of children except in patients with abnormal septal thickening (Figs. 10, 11, 27, 28, 45, and 46).
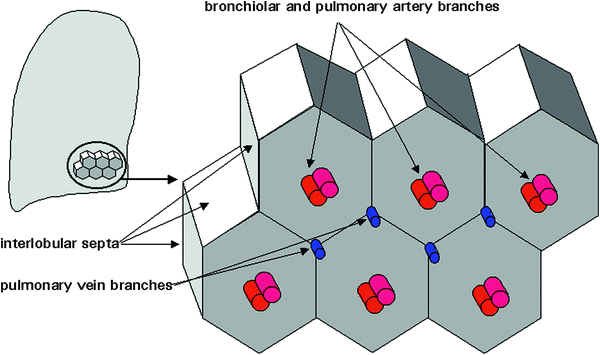
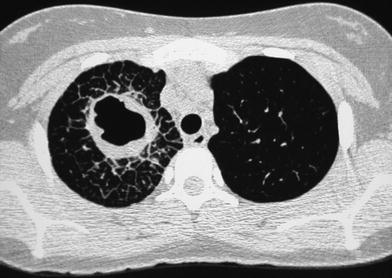

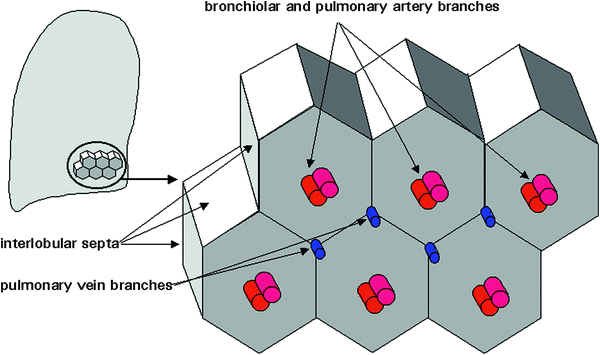
Fig. 9
The secondary pulmonary lobule, as defined by Miller. [Modified and reprinted with permission from Webb et al. (1996)]
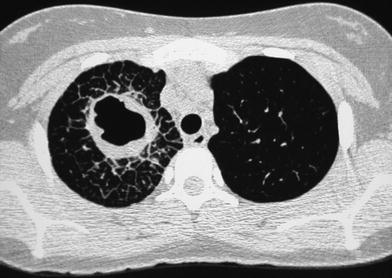
Fig. 10
A 15-year-old girl with fever and cough of 10 days duration, treated with oral antibiotics. HRCT demonstrates a cavity with thick, irregular wall in the right upper lobe. There is marked interlobular septal thickening around the lesion. Cultures were negatives. The patient responded to intravenous antibiotic therapy

Fig. 11
Septal thickening delineating the secondary pulmonary lobule in a 2-year-old patient with congenital atresia of the pulmonary veins (a). A 3-year-old boy with stenosis of the left upper pulmonary vein secondary to radiofrequency catheter ablation of the pulmonary veins for paroxysmal tachycardia (b) and a 5-year-old girl with pulmonary hypertension secondary to veno-occlusive disease (c)
The terminal bronchiole and the artery supplying the lobule are located in its center and give off smaller branches at intervals along their courses. On HRCT scans the vessels can be seen as linear, branching, or dot-like structures near the center of the secondary pulmonary lobule extending to within 5–10 mm of the pleural surface; the smallest arteries resolved are as small as 0.2 mm. Normal intralobular bronchioles cannot be identified because their walls are less than 0.15 mm thick. Bronchiolar abnormalities could be detected only when there was thickening of the bronchiolar wall, peribronchiolar inflammation, fibrosis, or bronchioloectasis, with or without filling of the dilated bronchiole with secretions. The sensitivity of last-generation CT scanners, which enables detection of abnormalities even in asymptomatic patients, has created new challenges for the radiologist. Sometimes there is no clear boundary between the normal and abnormal thin-section CT appearance of the lungs and the same finding may be unimportant in one situation, but clinically important in another (Hansell 2010). This is particularly relevant in neonates and young infants who do not follow breath-holding commands and in whom the presence of a diffuse ground-glass pattern most often reflects a normal lung on expiration. The attenuation of the normal air-containing lung varies with the phase of respiration and with the region of the lung being examined. With the child in a supine position, attenuation is usually higher posteriorly (lower lobes) than anteriorly (right middle lobe and lingular segments). This is due to physiologic hyperemia and the tendency of the dependent lobes to be incompletely expanded. This gravity-dependent density is accentuated at partial expiration, is reversible with full inspiration or with the patient in a prone position, and is most frequently observed in scans of children not following breath-holding commands whose studies are practically never performed on full inspiration. The opposite situation, i.e., anterior lobes denser than the dependent lobes, is always abnormal or air trapping in the dependent lobes (Fig. 12).
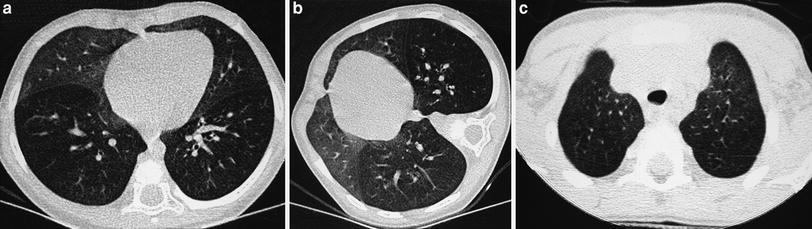
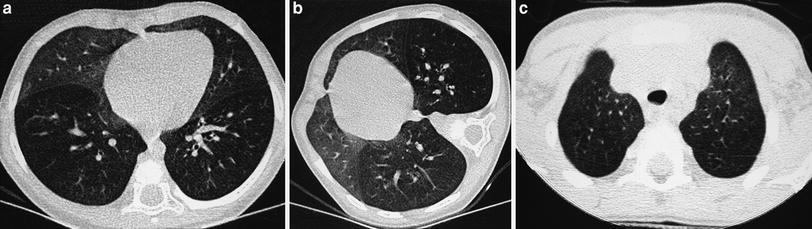
Fig. 12
Reversal of normal aeration pattern in a 15-month-old girl with biopsy-proven neuroendocrine cell hyperplasia. Axial scan (a) shows the reversal pattern. The right lateral decubitus scan confirms air trapping in right lower lobe (b). Left decubitus scan (not shown) demonstrated air trapping in the left lower lobe. Similar yet milder findings in a 4-year-old boy with cystic fibrosis (c)
6 HRCT Features of Lung Disease
The most common HRCT features of lung disease in children are grouped in Fig. 13.
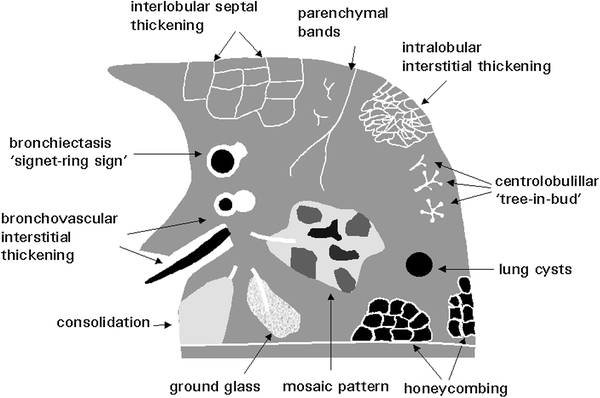
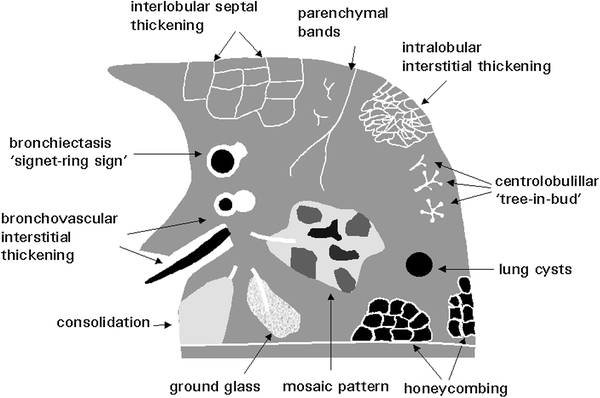
Fig. 13
HRCT features of lung disease. [Modified and reprinted with permission from Webb et al. (1996)]
6.1 Ground-Glass Opacity
Ground-glass opacity (GGO) refers to hazy increased attenuation of the lung with preservation of the bronchial and vascular margins, caused by partial filling of the airspaces, interstitial thickening, and partial collapse of alveoli, normal expiration, or increased capillary volume. It is sometimes associated with air bronchograms and can be patchy, resulting in a mosaic pattern of lung attenuation (Collins and Stern 1997). Lung attenuation normally increases with expiration. This increased attenuation can mask underlying GGO from infiltrative lung disease if the expiratory nature of the examination is not recognized. The tracheal configuration changes from round on inspiration to flat or crescent-shaped on expiration and can be used to determine at what phase of respiration HRCT scan was performed (Collins and Stern 1997).
Recognition of GGO is based on subjective assessment of the lung attenuation. When uniform GGO is observed in scans of children not following breath-holding commands, it probably corresponds to normal lung on expiration. Lateral decubitus views can help in this regard (Figs. 5, 6 and 12). When the GGO is patchy, it can cause a mosaic pattern of lung attenuation, which in children is usually due to small airway disease, with the GGO corresponding to areas of normal lung on expiration (Fig. 4b). Again, lateral decubitus views will help to establish whether the mosaic pattern corresponds to patchy ground-glass secondary to lung disease or to patchy air trapping. Assessment of true GGO in the scans of children following breath-holding commands is significantly easier. Its presence in these patients, whose scans are usually obtained in full inspiration, is always abnormal and is due to airspace or interstitial disease or both. The differential diagnosis of pathological GGO in the pediatric age group is extensive, with infectious pneumonia of any etiology being its most common cause (Fig. 14). GGO can also be seen in pulmonary edema (Fig. 15), hemorrhage, leukemic infiltration of the lung, lung contusion (Fig. 16), acute lung transplant rejection, graft-versus-host disease after allogeneic stem cell transplantation (Song et al. 2012), adult respiratory distress syndrome, collagen disease (Garcia-peña et al. 2011), extrinsic allergic alveolitis, interstitial pneumonia (Figs. 17, 18a, b) (Newman et al. 2001; Canakis et al. 2002; Popler et al. 2012; Lee 2013), drug toxicity (Fig. 18c), sarcoidosis, alveolar proteinosis (Fig. 45), bronchiolitis obliterans organizing pneumonia, idiopathic pulmonary fibrosis, and following bronchoalveolar lavage.
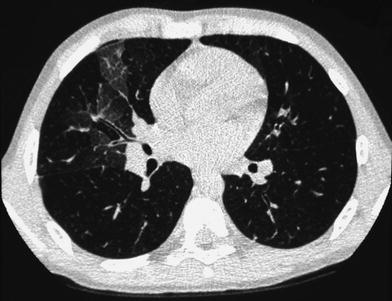
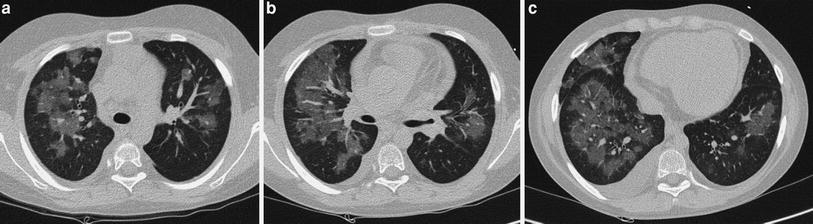
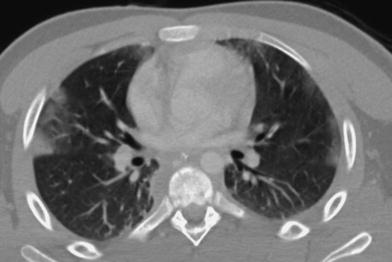
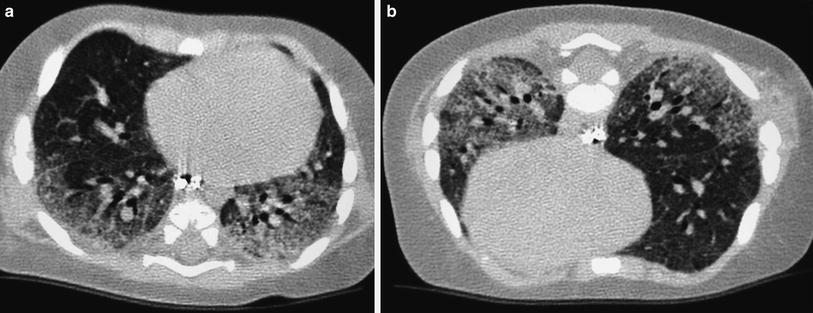
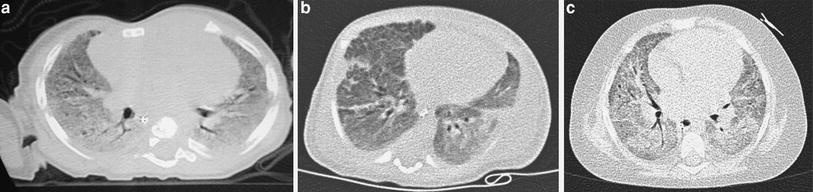
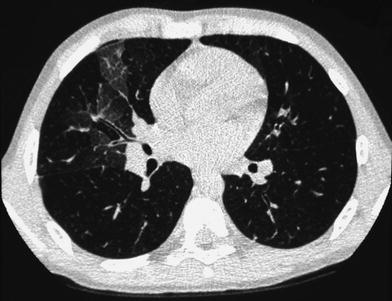
Fig. 14
Ground-glass pattern due to infectious right middle lobe pneumonia in a 9-year-old girl
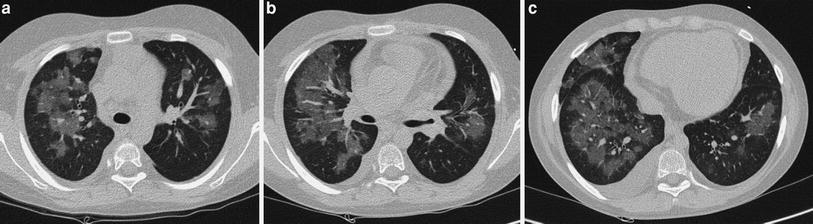
Fig. 15
a–c A 10-year-old boy with pulmonary edema. HRCT shows ground-glass opacities with a peribronchovascular distribution and pleural effusion
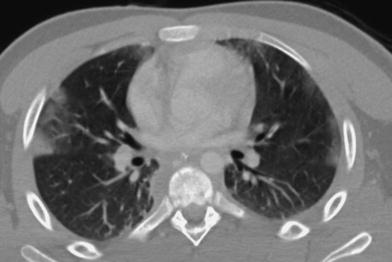
Fig. 16
A 15-year-old boy sustained lung contusions after a high-energy traumatism. Follow-up HRCT shows multiple peripheral areas of ground glass in both lungs. Also note thickened posterior mediastinum, secondary to thoracic duct rupture, which had been seen on previous volumetric CT
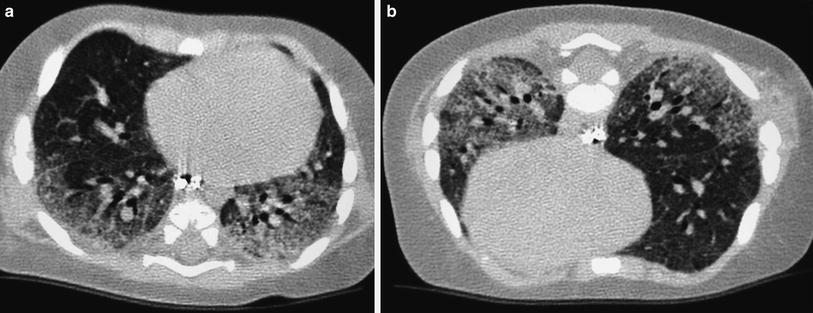
Fig. 17
Chronic pneumonitis of infancy in a 4-month-old boy. Supine scan shows ground glass in both lower lobes (a). This finding reminded unchanged in prone scans (b)
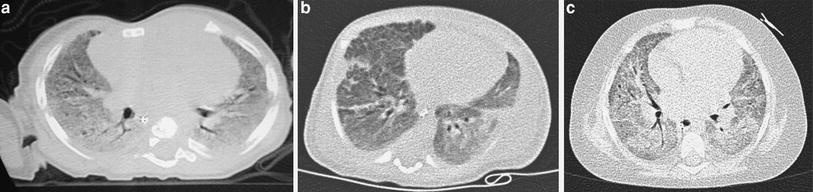
Fig. 18
Generalized ground-glass pattern. Chronic pneumonitis of infancy in a 6-month-old boy (a), biopsy-proven alveolar dysplasia in a 2-month-old girl (b), and bleomycin toxicity in 4-year-old girl with a yolk sac tumor (c)
6.2 Consolidation
A homogeneous increase in pulmonary parenchymal attenuation that obscures the margins of vessels and airway walls is referred to as consolidation. Air bronchograms may be present. By definition, diseases that produce consolidation are characterized by a replacement of alveolar air by fluid, cells, tissue, or other material. The differential diagnosis of consolidation overlaps that of GGO and, in fact, it is common to find a mixture of both findings. Pneumonia of any etiology, pulmonary edema or hemorrhage, and lung contusion are the most common causes of lung consolidation in children.
6.3 Pulmonary Nodule
Pulmonary nodules are focal, rounded opacities of varying size, which can be well or ill defined. They have been described as either airspace or interstitial nodules, but it is more practical to classify them according to their size and distribution. The term micronodule is reserved for round opacities less than 3 mm in diameter. The anatomic distribution may be classified as centrilobular, lymphatic, or random (Fig. 19).
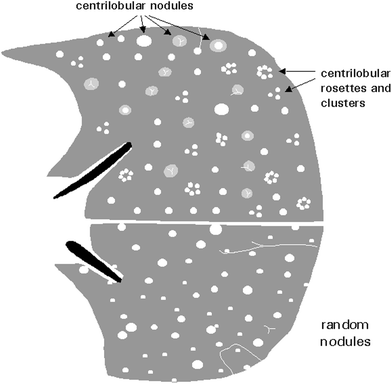
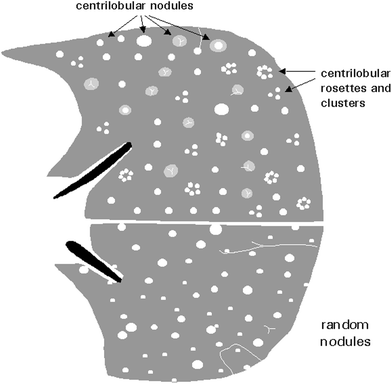
Fig. 19
Appearance of centrilobular and randomly distributed nodules [Modified and reprinted with permission from Webb et al. (1996)]
Centrilobular nodules are located in the region of the bronchio arteriolar core of secondary pulmonary lobules. On HRCT they are adjacent to, surround, or obscure the centrilobular arteries, and are centered or clustered 5–10 mm from the periphery of the lobe, pleural surface, or interlobular septa. Most centrilobular nodules in children are secondary to bronchiolar disease that also involves the peribronchiolar interstitium. They are common in cystic fibrosis (Fig. 20), bronchiectasis, infectious bronchitis, and bronchogenic spread of tuberculosis. They may also be present in immotile cilia syndrome (Fig. 21), hypersensivity pneumonia, asthma (especially when there is superimposed infection), histiocytosis, lymphocitic interstitial pneumonitis (LIP) in immunocompromised patients, congenital pulmonary lymphangiectasia, bronchiolitis obliterans, Wegener’s granulomatosis (Levine et al. 2007), and pulmonary hemosiderosis (Gruden et al. 1994).
