Fig. 16.1
Vero4DRT system
16.2 Specification of Vero4DRT
A system overview of Vero4DRT is shown in Fig. 16.2. The characteristic components of the system are an O-ring gantry, a beam delivery system, an imaging system and a precise robotic couch system. The rigid O-ring gantry supports both the subsystems of beam delivery and imaging, that allows accurate beam delivery and image guidance. The O-ring can skew around the vertical axis, which realizes non-coplanar irradiation without couch movement that may cause an intrafractional setup error.
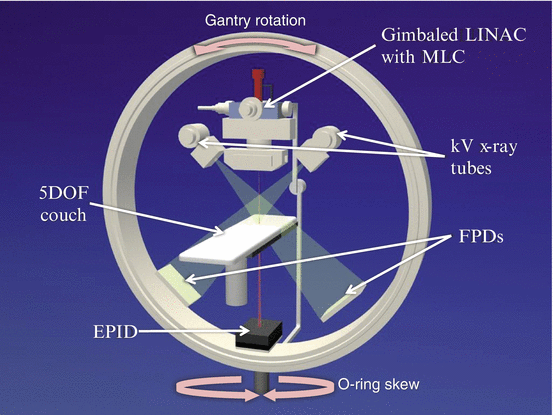
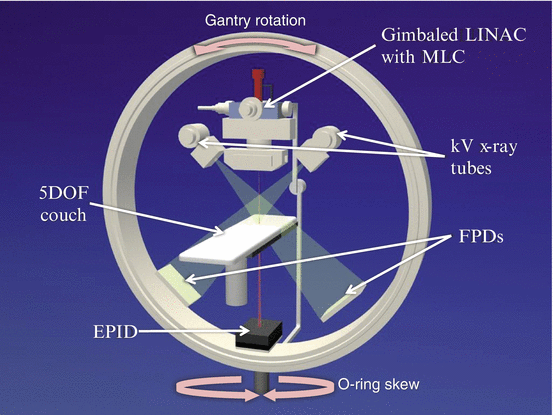
Fig. 16.2
Internal configuration of the Vero4DRT. Abbreviations: LINAC linear accelerator, MLC multi-leaf collimator, DOF degree of freedom, FPD flat panel detector, EPID electric portal imaging device
The beam delivery subsystem consists of an ultra-small C-band linear accelerator (LINAC), multi-leaf collimators (MLC) and a gimbals mechanism. The LINAC produces 6-megavolt (MV) photon beam with a maximal dose rate of 500 cGy/min. The MLC has 30 pairs of leaves of 5-mm thickness at the isocenter and allows a maximal field size of 15 × 15 cm2. The gimbals can swing the treatment beam along the two orthogonal gimbals (pan and tilt rotations) up to ± 2.4 degrees, which corresponds to ± 4.2 cm in each direction on the isocenter plane perpendicular to the beam. The gimbals mechanism realizes high accuracy in positioning of static treatment beam and DTT irradiation for a moving target.
The imaging subsystem includes two sets of kV x-ray on-board imaging (OBI) and an electric portal imaging device (EPID). The OBI provides orthogonal radiographs or cone-beam computed tomography (CBCT) for image-guided setup. The ExacTrac system (BrainLab AG) is integrated with the OBI system. The EPID visualizes a treatment field with a treatment beam through the patient body. Both of the imaging systems are used for real-time monitoring of treated position in DTT.
The robotic couch system can compensate setup errors with 5 degrees of freedom (translations along vertical, coronal and sagittal axes; and rotations of roll and pitch). The remaining yaw rotation is compensated with the O-ring skew. An infrared (IR) camera system helps precise localization of the couch. The IR camera also works for monitoring of respiratory phases via markers on the abdomen during DTT radiotherapy.
16.3 History of Development
The project for the development of Vero4DRT had started in 2000 in MHI. MHI, already having succeeded to introduce an ultrasmall C-band LINAC at the time [7], tried to develop a new radiotherapy machine with this LINAC on to gimbals mechanism, thus possessing the ability to track and irradiate a moving target. To realize this objective, MHI have started the collaboration with Kyoto University (Kyoto, Japan), Institute of Biomedical Research and Innovation (IBRI; Kobe, Japan) as medical partners. Machine design and concepts were discussed and decided in the collaboration team including functional enhancement of image guidance, high precision setup, and DTT. Some prototype machines were made and tested between 2002 and 2005.
The clinical version of the IGRT system was developed in 2006. The system was firstly approved by the US Food and Drug Administration in August 2007, and then by the Japanese Ministry of Health, Labor, and Welfare in January 2008 as “MHI-TM2000”. The CE Mark certification in Europe was acquired in 2010. Outside Japan, the unit is marketed as the “Vero” through BrainLAB AG as an original equipment manufacturer (OEM) supply partner.
The first application of the MHI-TM2000 to clinical treatment had been done in May 2008 at IBRI [8]. The clinical treatment initially applied to palliation of symptoms with metastasis to the bone or the lymph node. Then, the application was extended to high-precision radiotherapy with curative intent including SBRT for the lung using static beams and intensity modulated radiotherapy (IMRT) for the prostate. Kyoto University started treatment with the system in October 2010.
Based on the initial clinical experiences and physics evaluations, DTT with real-time monitoring was started with SBRT for lung tumor in September 2011 at Kyoto University [9] and in December 2011 at IBRI. DTT-SBRT for the liver was initiated in 2012 at UZ Brussel, followed by Kyoto University in March 2013. After success of DTT-SBRT, MHI changed the market name for the system in Japan from “MHI-TM2000” to “Vero4DRT”. DTT was also combined with IMRT. Kyoto University performed DTT-IMRT for pancreatic cancer in June 2013 [10, 11]. This is the world’s first case treated with tumor tracking IMRT.
16.4 Physics Evaluation and Clinical Application
16.4.1 Physics Evaluation
For introduction of the new treatment technique to the clinics, extensive basic evaluation has been done. Table 16.1 summarizes results of the physics evaluations for the Vero4DRT system. The evaluated items include accuracy in static beam delivery [12, 13], specification of MLC [14], accuracy in image guidance [15], errors in dynamic tracking irradiation [12, 16–21] and commissioning process [22].
Table 16.1
Summary of physics evaluations for the Vero4DRT system
Evaluation | Results | Author (year) | |
---|---|---|---|
Static beam | Positioning accuracy (RMS) | 0.08 mm (pan axis), 0.10 mm (tilt axis) | Kamino (2006) [12] |
IC radius in starshot | 0.12–0.43 mm (gantry), 0.01–0.08 mm (O-ring) | Depuydt (2012) [13] | |
MLC | Field size | <0.5 mm | Nakamura (2010) [14] |
Leaf position | 0.0 ± 0.1 mm | ||
Leakage | <0.21 % (interleaf), <0.12 % (intraleaf) | ||
Image guidance | Error at 50-mm offcenter | 0.2 mm (kV x-ray), 1.0 mm (CBCT) | Miyabe (2011) [15] |
Tracking | Beam positioning error | <0.6 mm | Kamino (2006) [12] |
(Direct tracking) | Motion blurring effects | significantly reduced | Takayama (2009) [16] |
System lag | 47.7 ms (pan), 47.6 ms (tilt) | Depuydt (2011) [17] | |
Tracking error (90-percentile) | 0.54 mm | ||
Tracking error (RMS) | 1.2 mm (prediction), 0.1 mm (mechanical), 1.2 mm (total) | Mukumoto (2012) [18] | |
Tracking | Tracking error (SD) | 0.55 mm (pan direction), 0.95 mm (tilt direction) | Depuydt (2013) [19] |
(Model based tracking) | 4D modeling error (SD) | 0.1–1.0 mm (LR), 0.1–1.6 mm (AP), 0.2–1.3 mm (SI) | Akimoto (2013) [20] |
Tracking error (95-percentile) | <1 mm | Mukumoto (2013) [21] | |
Other | Commissioning process | Solberg (2014) [22] |
16.4.2 Initial Clinical Application
In the first phase of the clinical application, ten palliative treatments were performed to the patients with bone metastases and/or lymph node metastases at IBRI [8]. The purpose of the phase was to check the usability and the limitation of the system functions. The typical IGRT session for palliation took less than 10 min including patient setup, image-guidance, verification, and beam delivery. Through the first phase, an IGRT flow suitable for the system was established. In the next phase, high-precision radiation treatments including IMRT for the prostate and SBRT for the lung were performed. Image-guided setup verification after couch correction demonstrated that the setup error was mostly within 1 mm. Accuracies in image guidance, couch movement and beam delivery were confirmed in the clinical situations.
16.4.3 Dynamic Tracking Treatment
Based on the physics and clinical evaluations as described above, DTT with the Vero4DRT was considered to be feasible. We conducted a prospective feasibility study on DTT-SBRT for the lung after approval from the institutional review board and a revision of the software in the Vero.
Details on our procedures for DTT were available in the previous paper [23]. Figure 16.3 summarizes the treatment steps. Spherical gold markers with a diameter of 1.5 mm (FMR-201CR; Olympus Medical Systems, Tokyo, Japan) were placed around the tumor under bronchoscope guidance prior to treatment planning. A simulation with 4D CT was performed 1 week after the marker placement. An internal target volume (ITV) for tracking was delineated on a breath-hold CT at end-exhale followed by modification to compensate intrafractional variations between the fiducials and the tumor using the 4D CT [24]. The 4D modeling, which correlates abdominal motion with tumor position during respiration, was performed in the planning procedure and in each treatment fraction. Planning target volume (PTV) for tracking was defined as the ITV plus additional internal margins and setup error. The additional internal margins were defined individually for each patient, considering the 4D modeling errors and baseline drift of the abdominal position. Prescription dose was 48 or 56 Gy in 4 fractions at the isocenter. Treatment beam irradiation was performed with the gimbaled x-ray head toward predicted position based on the 4D model and the abdominal wall. During the irradiation, the tumor and the fiducials were monitored with OBI and EPID.
