Fig. 3.1
Diffusion MRI of one normal volunteer. The images show: a nondiffusion weighted image, b diffusion weighted image (b-value = 1,000 mm2/s), c mean diffusivity, d axial diffusivity, e radial diffusivity, and f fractional anisotropy. It should be noted that the contrast in the nondiffusion-weighted image (a) is intrinsically T2 weighted. The contrast in diffusion-weighted image (b) is potentially negative such that regions with high ADC appear dark but bright in the mean diffusivity (c). The intensity in fractional anisotropy is high in white matter where the water diffusion in the neuron fibers results in increased diffusion anisotropy
Tractography
There has been a strong interest in using DTI to perform in vivo tractography, since its invention. The technique assumes the direction of the principal diffusion in DTI is collinear to that in the neuron fibers. A voxel-by-voxel connection can be delineated from a seeding point and propagated along the direction of the principal diffusion. Early work by Conturo et al. [17] successfully demonstrated the fibers along the curvature of the splenium and extended into the occipital and parietal lobes. Various tracking algorithms have since been proposed and many freeware applications are currently available [18–20].
Tractography has been used in presurgical planning to guide surgical margins away from the involvement of neuron fibers for improved surgical outcome [21, 22]. Tractography can be used to differentiate infiltration from cytotoxic edema in order to assess the patient prognosis after successful surgery [23, 24]. It has also been used to visualize neural network integrity and changes in connectivity in neurodegenerative disorders [25, 26].
Diffusion Imaging in Movement Disorders
Morphological studies of neurological movement disorders using MRI commonly report various extents of atrophy in relevant brain regions [31, 32]. Since functional alteration may precede the observable anatomical changes, diffusion imaging was expected to bring new insight into the underlying mechanism of the disease and provide improved diagnosis and prognosis information. In the following section, we will describe recent findings in the major parkinsonian disorders, including idiopathic Parkinson disease (iPD), progressive supranuclear palsy (PSP), and multiple system atrophy (MSA) .
Idiopathic Parkinson Disease
The motor symptoms of idiopathic Parkinson disease have been attributed to dopaminergic neuronal death within the basal ganglia. There is a growing interest in imaging Parkinson disease with diffusion MRI, because of the potentially increased extracellular space due to cell death that may be detectible by ADC [33]. In a whole-brain analysis of patients with de novo iPD, an increased FA was noted and was more profound in the akinetic rigid subtype of iPD [34].
Region of interest (ROI) -based studies have usually focused on the basal ganglia. The findings from DTI are still controversial. In a case-control study, Chan et al. [35] reported no significant differences except in substantia nigra where FA was significantly reduced in patients and overlap was present between patients and controls. In contrast, Vaillancourt et al. [36] showed that FA in the rostral part of substantial nigra can separate the patients with iPD from normal, with a sensitivity and specificity of 100 %.
Using a voxel-wise analysis, additional brain regions that could be affected were reported, including decreased FA in bilateral frontal lobes, the supplementary motor area, the presupplementary motor area, and the cingulum [37]. White matter involvement has also been detected in patients using TBSS, which suggests an altered neural network integrity involving basal ganglia regions and extending up to the supplementary motor area in the patients [38].
Progressive Supranuclear Palsy (PSP)
The neuropathology of progressive supranuclear palsy (PSP) is related to neuronal and glial lesions of tau protein, with preferential involvement of the basal ganglia, midbrain, pons, and medulla [39, 40]. In basal ganglia and the surrounding white matter, a reduced FA and an increased MD have been noted; both being related to an increase in RD. This observation was attributed to reduced myelination [41]. DWI can also discriminate patients with PSP from iPD, with an increased ADC located in putamen, globus pallidus, and caudate nucleus in PSP [42].
White matter-tract degeneration was also reported [43], noticeably in the superior cerebellar peduncles and corpus callosum, where the measured FA correlated with disease severity. Similar regions were reported by Knake et al. [44] using TBSS. The changes may be related to an increase of RD and the authors attribute the image findings to axonal and/or myelin alterations.
Multiple System Atrophy (MSA)
Patients of multiple system atrophy (MSA) show varying degrees of atrophy in the central nervous system including the cerebellum, cerebellar peduncles, pons, medulla, and putamen, together with excessive iron deposition within the striatum [45]. DTI studies of the basal ganglia have found increased MD and a reduced FA when compared with normals, particularly in the putamen [46]. ADC is also significantly higher in pons, cerebellum, and putamen [47]. The increased ADC in putamen helped distinguish MSA from iPD and healthy volunteers [48]. ADC values in putamen of patients of the parkinsonian subtype of MSA (MSA-P) were positively correlated with clinical severity as assessed by both the Unified Multiple System Atrophy Rating Scale and the Motor section of the Unified Parkinson’s Disease Rating Scale (UPDRS) [49]. Other regions with increased ADC included the middle cerebellar peduncles and cerebellum. Reductions in FA have also been found in the pyramidal tract, middle cerebellar peduncles, and white matter of the cerebellum [50]. It should be noted that white matter abnormalities were both supra and infratentorial [45]. Patterns of degeneration include progressive involvement of the corticopontocerebellar tract, selective loss of the transverse pontocerebellar tract, and preservation of the corticospinal tracts [51].
Limitations of Diffusion Tensor Imaging (DTI)
Although clinically successful, DTI comes with many limitations [52]. In clinical practice, no accepted model exists regarding changes observed in gray matter. Therefore, it is difficult to interpret the findings involved in cortical or deep gray matter regions, which are particularly relevant to movement disorders [41]. Efforts to better resolve the multiple fiber directions in a voxel of interest have included diffusion spectrum imaging [53] and q-ball imaging [54]. While these newer methods have been successful, the directional resolution was often achieved with prolonged acquisition times.
Diffusion Kurtosis Imaging (DKI)
Diffusion in a biological environment can be significantly different from that of a free and homogeneous medium. Therefore, the distribution of water diffusion maybe deviated from normality. It has been observed that the signal attenuation curve induced by water diffusion is no longer an ideal monoexponential shape, and was often modeled by the weighted sum of two exponential curves, a phenomena referred to as biexponential decay [55]. The observed signal decay was assumed to be a summary effect from different contributions in the intracellular and extracellular compartments.
DKI attempts to quantify the extent of deviation from Gaussian distribution. Kurtosis is a statistical term used to describe the degree of deviation from a Gaussian distribution in a population. If the distribution is more peaked in the center but reduced in both tails, the kurtosis will increase even though the mean remains unchanged. On the other hand, if the distribution is flat in the centre but increased in tails, the kurtosis decreases.
The measured diffusion kurtosis is along the specific gradient direction of interest. Usually, images with multiple different b-values are acquired. Both the diffusion coefficient and the diffusion kurtosis can then be calculated using curve fitting. The apparent diffusion kurtosis experiment can be further extended to a full kurtosis tensor in a manner similar to DTI . The diffusion kurtosis tensor is a matrix of 81 elements (3 by 3 by 3 by 3) [56]. Because of symmetry, at least 15 nonlinear diffusion-encoding directions are required. Indices can be derived from the full kurtosis tensor, including the mean kurtosis, axial kurtosis, radial kurtosis, and kurtosis of FA. Jensen et al. [56] recommend an acquisition scheme for the reconstruction of the full DKI tensor using 3 b-values of 0, 1,000, and 2,000 mm2/s and 30 diffusion-encoding directions.
Diffusion Kurtosis Imaging in Idiopathic Parkinson disease (iPD)
A recent study of iPD using DKI has brought new interest to the topic. Wang et al. [57] showed that patients with probable iPD have a significantly increased diffusion kurtosis in all regions of basal ganglia when compared with the age-matched healthy controls. The mean diffusion kurtosis was calculated from a series of diffusion-weighted images with b-values ranging from 0 to 4,000 mm2/s. The mean kurtosis was compared with the corresponding MD and FA for diagnostic performance. The results indicated that the area under curve from DKI in all regions within basal ganglia outperformed conventional diffusion tensor. The sensitivity and specificity of mean kurtosis values in the substantia nigra were of 87 and 92 %, respectively. The best diagnostic predictors were the ipsilateral substantia nigra and putamen, where the areas under the receiver-operating characteristic (ROC) curves were both 95 %. Although statistically significant, the area under the ROC curve in the corresponding FA was only 65 %.
The study showed that mean kurtosis in all basal ganglia regions were significantly higher in patients with iPD as compared with the healthy subjects and could distinguish these two groups. DKI compares favorable with current dopamine transporter-imaging methods that require the use of radioactive ligands. Figure 3.2 demonstrates the contrast of DKI is good enough for visual diagnosis. The increased sensitivity and specificity of DKI, with it intrinsically non-invasive nature, could compensate for the extended acquisition time when compared to DTI. Using the modern echo planar imaging technique and for a whole brain coverage, this additional time could be less than minutes. In conclusion, DKI can lead to a substantial improvement in the MRI-based diagnosis of iPD.
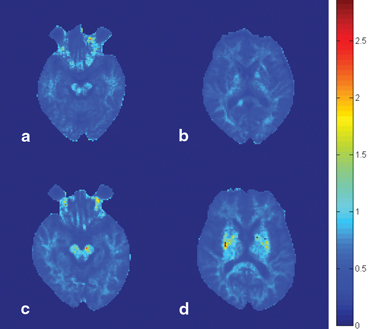
Fig. 3.2
Diffusion kurtosis imaging in the diagnosis of Parkinson disease. The images show the mean diffusion kurtosis from a normal volunteer (a and b) and a patient with Parkinson disease (c and d). A significant increase in diffusion kurtosis can be noted in the substantia nigra (c) and striatum (d), compared with the corresponding locations in the normal volunteer (a and b, respectively)
Conclusions and Future Directions
Diffusion magnetic resonance imaging has been widely used in studies of neurological disorders with demonstrated success, specifically in applications related to white matter. The application of these exciting new methods to neurological movement disorders provide novel ways to explore brain structure for clinical diagnostic purposes and a better understanding of pathophysiology. DKI has provided a preliminary success in the diagnosis of Parkinson disease . In the future, characterization of restricted diffusion in the brain in general and gray matter in particular will be of interest, since it may shed new insights into the microstructural environmental changes that take place in neurodegeneration.
References
1.
Stejskal D. Use of spin echoes in a pulsed magnetic-field gradient to study anisotropic, restricted diffusion and flow. J Chem Phys. 1965;43(10):3597–603.CrossRef
2.
Moseley ME, et al. Diffusion-weighted MR imaging of anisotropic water diffusion in cat central nervous system. Radiology. 1990;176(2):439–45.PubMed
< div class='tao-gold-member'>
Only gold members can continue reading. Log In or Register a > to continue
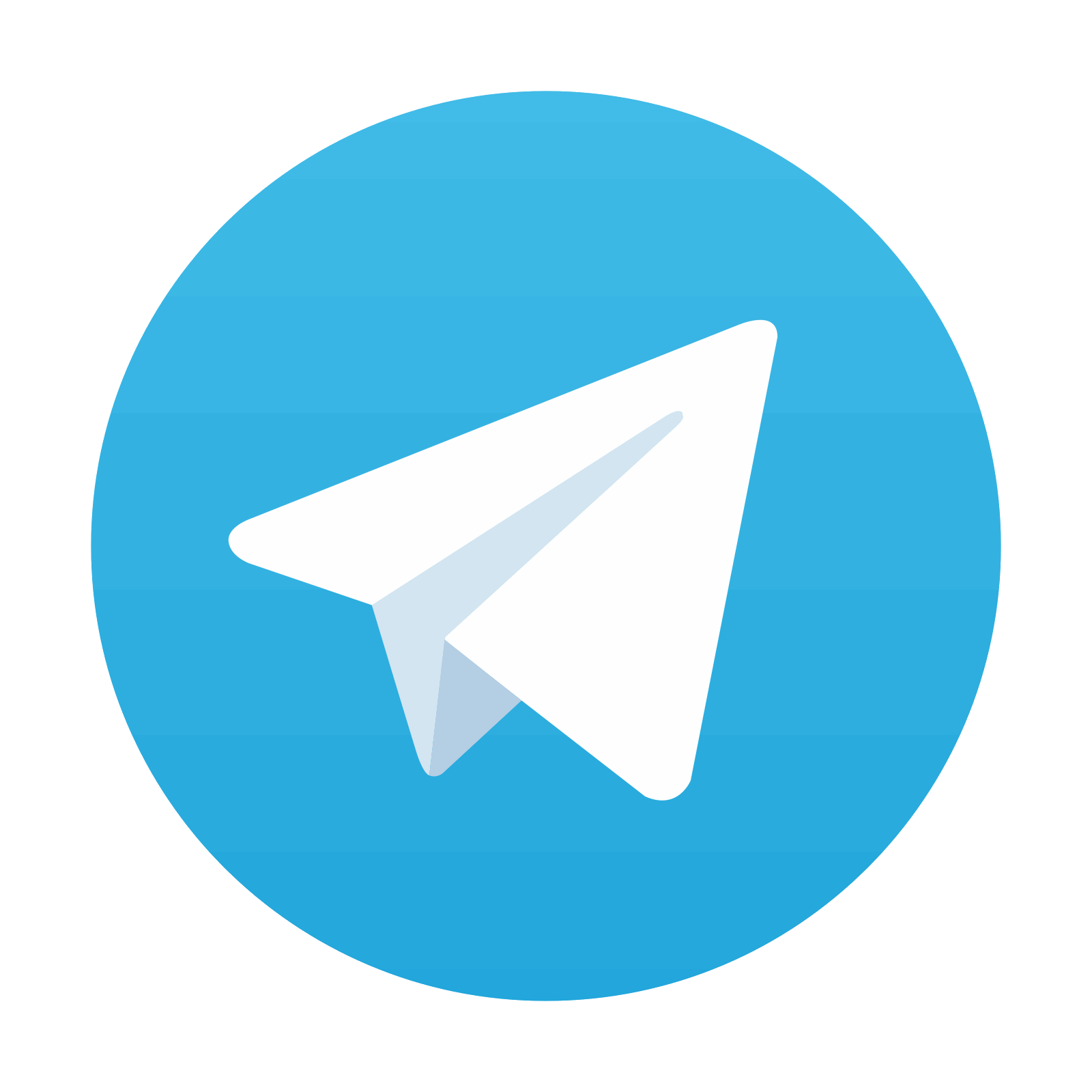
Stay updated, free articles. Join our Telegram channel

Full access? Get Clinical Tree
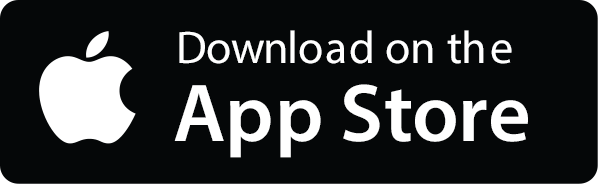
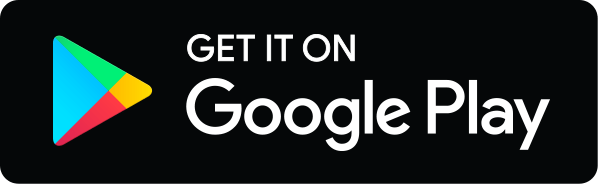