Fig. 12.1
Conjunction analysis of resting-state fMRI-based connectivity map demonstrating greater connectivity in brainstem and cerebellar structures in ET subjects compared with controls despite the absence of tremor in both groups
Positron Emission Tomography (PET)
PET is a sensitive imaging technique involving the intravenous injection of radiolabeled ligands binding to specific molecular targets. PET can provide information about the regional distribution and affinity of receptors in the brain or cerebral metabolism noninvasively and with high sensitivity in the living brain. The regional cerebral metabolic rate of glucose can be assessed using [18F]-fluoro-2-deoxyglucose (FDG). Using FDG PET, Hallett and Dubinsky [45] demonstrated increased activation in medullary structures and thalamus in ET patients, but not in the cerebellum. Other PET studies have used 15O-labeled water to measure regional cerebral blood flow and showed an increased flow bilaterally in the cerebellum with normal olivary activity in the ET group [46]. A variety of PET studies have demonstrated involvement of the motor system in ET, including contralateral thalamus, striatum, sensorimotor, and premotor cortex, as well as the red nucleus and parietal cortex [47–49].
Functional brain imaging with PET and 6-[18F]-fluoro-L-dopa (FDOPA) is commonly used to assess the nigrostriatal dopamine system in iPD. In ET, most studies have not shown significant changes in striatal FDOPA uptake. However, one study by Brooks et al. [50] showed a 10–13 % reduction in FDOPA uptake in the striatum of patients with ET when compared with controls, suggesting in some cases an overlap between the ET- and iPD-associated nigrostriatal degeneration. The pattern of striatal uptake loss varied by disorder, with predominant loss in caudate in ET and tremor-dominant iPD, while predominately reduced uptake in the putamen was seen in akinetic-rigid iPD.
PET has also been used to assess changes in the oscillatory brain network of ET after DBS. PET studies in patients with ET during tremor-suppressive Vim DBS demonstrated increased regional cerebral blood flow (rCBF) in the ipsilateral primary motor cortex [51] and in the ipsilateral supplementary motor area [52]. Vim DBS was also shown to normalize reduced cerebellar inhibition on the M1 area [53], though it remains unclear whether these findings simply reflected the reduction in tremors post-DBS.
The hyperactivity in the CTC circuits have led to the hypothesis that disturbance in γ-aminobutyric acid (GABA) , an inhibitory neurotransmitter in the CNS, is responsible for ET [54]. This is supported by the tremor suppression properties of GABAergic medications, and by decreased GABA receptor densities in the dentate nucleus of ET patients [55]. GABAergic function has been assessed using [11C]-flumazenil PET, a benzodiazepine receptor sites ligand, in a group of 8 patients with bilateral ET, as compared with 11 healthy controls. Significant increases in [11C]-flumazenil binding at the GABA(A) receptor concentrations were found in the cerebellum, ventrolateral thalamus, and lateral premotor cortex of the ET group. This impaired GABA function is thought to reflect an overactivity of the CTC circuits seen in ET [56].
While PET provides unique contributions not afforded by MRI, certain limitations continue to limit its widespread utility. These include relatively low spatial resolution, exposure to ionizing radiation, the need for an extensive infrastructure to synthesize the tracers, and the short half-life of the radionucleotides used. Furthermore, PET cannot provide anatomical information by itself and is often combined with computed tomography in order to show both metabolic functions and accurate anatomical structures.
Single-Photon Emission Computed Tomography (SPECT)
Similarities exist between PET and SPECT-based nuclear-imaging methods, though it is important to understand some basic differences. Whereas PET imaging detects the indirect production of opposite pairs of gamma rays emitted by a positron decay, SPECT imaging directly measures the gamma radiation emitted by the radiotracer . These differences have direct clinical consequences, with SPECT imaging having reduced sensitivity compared with PET. Advantages to SPECT include longer half-life radiotracers, lower cost, and reduced infrastructure required. Among a variety of SPECT radiotracers are cocaine analogues such as [123I]-β-CIT, [123I]-FP-CIT, and [18F]-DOPA. These radioligands label the presynaptic dopamine transporters and are used to quantify nigrostriatal degeneration. SPECT imaging has significant clinical value for the differentiation of patients with ET from patients with iPD based on the consistent findings of decreased ligand binding in the case of iPD.
Striatal [123I]-β-CIT binding in patients with ET was evaluated with the purpose of identifying a subclinical dopaminergic nigrostriatal loss in ET. The binding of β-CIT was shown to be in the normal range in ET patients in accordance with findings from FDOPA PET studies, suggesting that this technique can successfully distinguish between ET and early iPD [57].
[123I]-Ioflupane SPECT has also been used to evaluate dopamine transporter (DAT) binding in ET. Multiple studies report that DAT-SPECT can distinguish parkinsonism from ET given the consistent decrease in DAT ligand binding in PD. However, the DAT binding in ET was found to be reduced by 15 % in putamen and 21 % in caudate when compared with healthy subjects. This suggests that some ET patients may present mild abnormalities of striatal dopamine transporters, similar to those seen in iPD [58]. In a follow up study that assessed DAT uptake loss over time, the annual decline of DAT binding was 7.5 % in PD patients. The DAT binding was not changed in the putamen of ET patients, but showed mild reductions in the caudate nucleus [59]. Currently, the clinical utility of [123I]-Ioflupane SPECT is limited to cases when there is clinical uncertainty regarding the diagnosis of ET. Furthermore, a limitation of these studies remains the lack of a validated quantitative measure to apply clinically. Attempts to develop such a measure suggest that a striatal asymmetry index is useful in differentiating iPD from other disorders.
Magnetic Resonance Spectroscopy (MRS)
MRS is a powerful method for direct and noninvasive identification of major metabolites in the brain. MRS can make in vivo inferences about the neuropathology of ET by characterizing the relative proportions of different brain metabolites. Furthermore, measurement of concentration and synthesis rates of specific biochemical compounds can be obtained, with spatial localization, in defined areas in the brain. Typically, 1H-MRS can detect metabolites reflecting neuronal density and integrity, markers of energy metabolism or inflammation, as well as neurotransmitters (see Chap. 1 for additional details). In the setting of ET, MRS studies have detected a selective reduction of the neuronal marker N-acetyl-aspartate (NAA) in the cerebellum that correlates with the severity of tremor [60, 61]. The reduction in cerebellar NAA/Creatine (Cr) represents neuronal damage or loss and suggests, similar to other imaging studies, that ET may be a neurodegenerative disease that affects the cerebellum.
Magnetoencephalography (MEG)
MEG is a method used for mapping brain activity by recording magnetic fields occurring naturally in the brain, using superconducting quantum interference devices (SQUIDS). The availability of MEG systems and modern spatial filter-based analysis tools permits the analysis of oscillatory cerebral networks in ET and has the potential to provide new insights into the physiological mechanisms of motor control and pathophysiological mechanisms of tremor disorders.
Using a localization technique called dynamic imaging of coherent sources (DICS) to identify cerebral areas coherent with the EMG signal at tremor frequency (5–7 Hz), patients with ET showed coherence to the contralateral primary motor cortex, premotor cortex, thalamus, brainstem, and ipsilateral cerebellum [14]. MEG-EEG dynamic coherence is able to distinguish changes in the tremor network between the ET patients and voluntary mimicked tremors. Muthuraman et al. [62] identified symmetric and direct connections between thalamic regions and motor cortex, forming thalamocortical loops responsible for pathologic oscillations and providing further evidence regarding the tremor network in ET. These observations lead to the conclusion that the inferior olive, cerebellar nuclei, and the thalamus are key structures in the tremor network with cortical motor regions being intermittently entrained in the tremor rhythm in thalamocortical loops [63].
Transcranial Sonography (TCS)
TCS allows high-resolution imaging of deep brain structures and is able to identify increased echogenicity of substantianigra in iPD. Substantia nigra hyperechogenicity (SN+) on TCS is defined as an enlargement of the echogenic area of the SN in the brainstem. Previous studies have demonstrated that SN+ is associated with increased vulnerability of the nigrostriatal dopaminergic system and a risk factor for iPD. Most of the studies published thus far have shown that SN+ is a typical finding in about 90 % of patients with iPD, but not in patients with ET [64]. In ET patients, the prevalence of SN+ is in the range of healthy control subjects or slightly above [65], which may indicate an increased risk for iPD in the subgroup of ET patients. Further studies are needed to establish whether this same subgroup of ET with SN+ may go on to develop iPD. Midbrain sonography shows SN+ in 13 % of patients with ET, 91 % of PD patients, and 10 % of healthy subjects [66].
TCS sensitivity and specificity has also recently been examined alongside [123I]-FP-CIT SPECT to differentiate iPD from ET. Findings showed sensitivity and specificity of TCS for detection of iPD were 86 and 93 %, respectively, suggesting that TCS can reliably differentiate between ET and iPD [67].
Conclusions and Future Directions
The exploration of structural and functional correlates of ET using neuroimaging has led to significant progress in the past decade. Findings till date implicate both cortical, subcortical, brainstem, and cerebellar areas in the ET brain. These widespread changes also provide support for ET being a neurodegenerative disorder, though this remains highly controversial. Despite the consistent findings of cerebellar involvement in ET, much work remains in trying to separate those brain changes that are a direct consequence of ET from more nonspecific changes seen during the process of generating a tremor. Furthermore, ET is a highly heterogeneous disorder with varying age of onset, heredity, and phenotype. Future studies of ET will need to markedly extend phenotypic–genotypic characterization and classification while combining various imaging modalities to achieve both high spatial and temporal resolutions. Achieving these objectives will provide a better understanding of clinical-genetic-imaging correlations, and ultimately shed light on the molecular basis underlying ET.
References
1.
2.
3.
4.
5.
6.
7.
Deuschl G, Bain P, Brin M. Consensus statement of the movement disorder society on tremor. Ad hoc scientific committee. Mov Disord. 1998;13(Suppl 3):2–23.PubMed
8.
9.
Zesiewicz TA, Elble RJ, Louis ED, et al. Evidence-based guideline update: treatment of essential tremor. Neurology. 2011;77(19):1752–5.PubMedCrossRef
< div class='tao-gold-member'>
Only gold members can continue reading. Log In or Register a > to continue
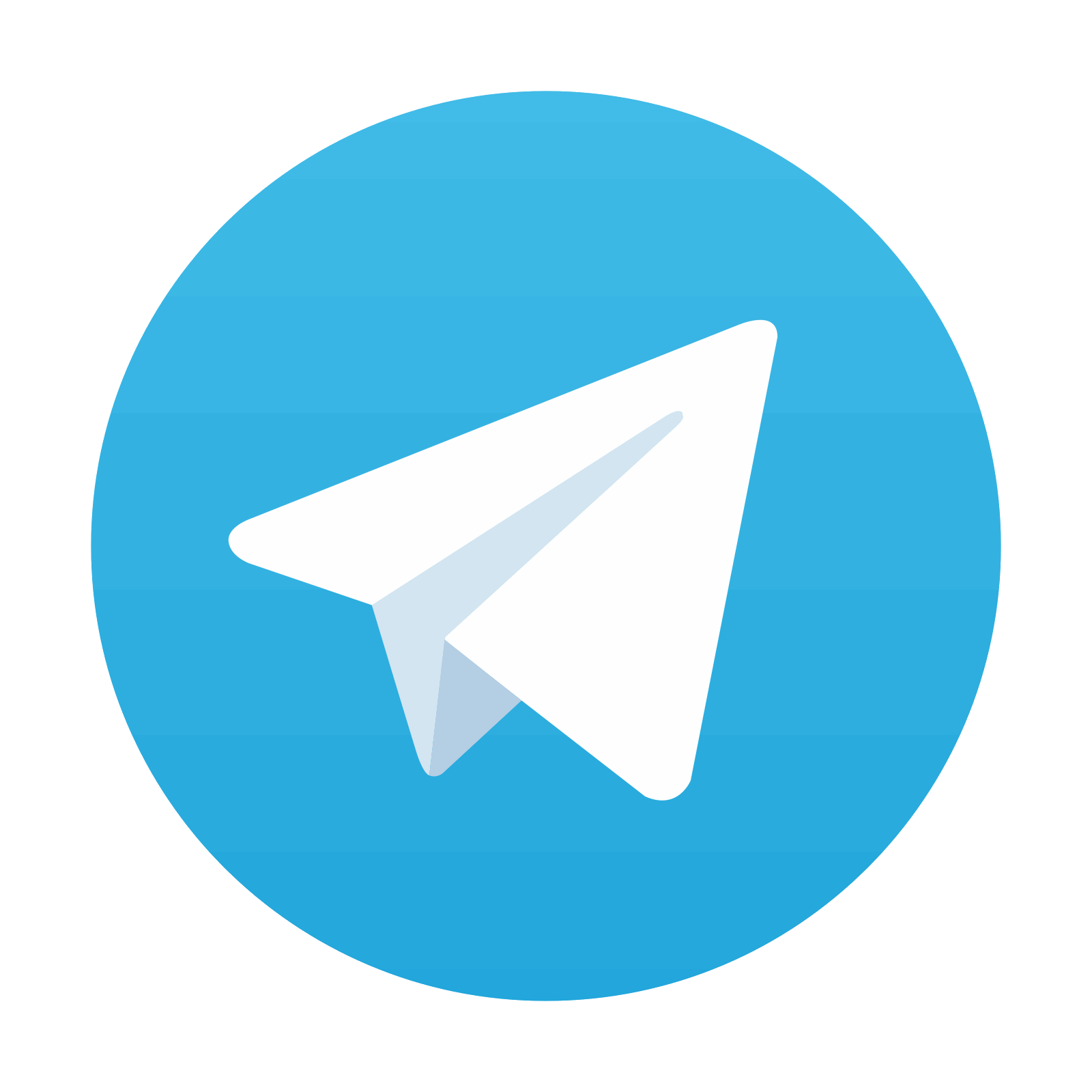
Stay updated, free articles. Join our Telegram channel

Full access? Get Clinical Tree
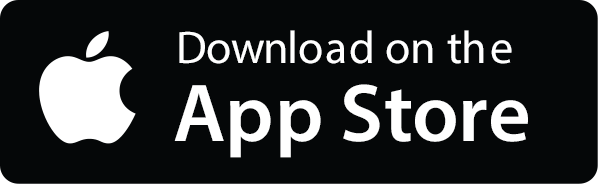
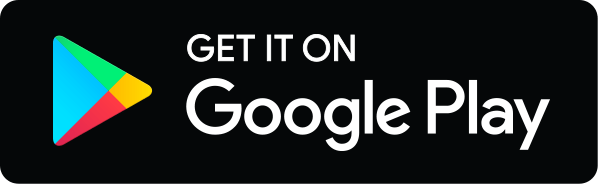