Fig. 11.1
Gray matter volume changes in blepharospasm patients. Significant differences in gray volume, detected using voxel-based morphometry are seen in the sensorimotor area (data from [37]). Regions are shown on axial and coronal slices and on a 3D reconstructed brain
Similar to the changes in gray matter, metabolic changes in the resting state were seen in several areas known to be related to blink production and control. Glucose metabolism , measured with 18F-FDG PET, was increased in cortical regions, including inferior frontal gyri, right posterior cingulate gyrus, left middle occipital gyrus, right fusiform gyrus, and left anterior cingulate gyrus. Subcortical areas were also affected, with increased metabolism in the right caudate and decreased in the left inferior cerebellar hemisphere and thalamus [40]. Glucose hypermetabolism in the thalamus and pons was observed in patients treated with botulinum toxin, suggesting these changes are intrinsic to the disorder and not dependent on sensory feedback from the eyelid spasms [41]. In another study, however, metabolic changes in the thalamus correlated with disease severity [36]. Thalamic hypermetabolism (in ventral anterior (VA) and ventral lateral (VL)) was more pronounced in blepharospasm patients with photophobia compared with those without photophobia. Nonphotophobic blepharospasm patients also had decreased metabolism in their midbrain [42].
Some metabolic studies have explored changes in different brain states. rCBF, measured with H2 15O PET, was decreased in the primary sensorimotor area in response to lower face stimulation [43]. The sleep–wake cycle also affects metabolism in patients. During wakefulness, there was increased metabolism in the cerebellum and pons. During sleep, when blepharospasm movements are suppressed, there was decreased metabolism in the superior-medial frontal cortex, an area associated with eyelid control [44]. These studies showed that metabolism is sensitive to the precise state of the patient and likely the frequency of eye blinking; this may explain some of the variability of the metabolic studies done “at rest.”
There are suggestions of abnormal sensory integration and basal ganglia function with studies not focused on eyelid control. fMRI studies of blepharospasm patients evaluated brain activity elicited by nondystonic motor tasks. During handgrip, the thalamus, caudate nucleus, putamen, and globus pallidus were more active in patients than in controls [45]. During whistling, blepharospasm patients have increased activity in the postcentral cortex and caudal supplementary motor cortex bilaterally, left dorsolateral prefrontal cortex, and left paravermal cerebellum [46]. Using fMRI, activation with patients’ blink spasms was compared with healthy volunteers’ volitional blinks. Blink spasms increased activation in the putamen [47]; when patients added volitional blinks to their spasms, cortical areas related to blink production (anterior visual cortex, anterior cingulate, primary motor cortex, thalamus, and superior cerebellum) were more active than in the controls [48]. Forehead stimulation produced increased activation in the putamen that was reversed by botulinum toxin treatment [49].
There is great variability in the results from the different imaging modalities and even within modalities. The variability maybe, in part, due to the inhomogeneity of the population sampled and the different sensitivity of each modality utilized. In addition, different imaging parameters or processing methods used within the same modality can lead to different results. However, put together, these studies find structural, functional, and metabolic deficits in areas seen to produce secondary blepharospasm, such as thalamus [50], basal ganglia [51], cortical areas involved in blink control such as sensorimotor cortex and anterior cingulate among others, and in the cerebellum, an area thought to have a role in dystonia [52].
Cervical Dystonia
One goal of neuroimaging studies is to find the sites of pathology. Neuropathology studies, which would be the most direct method, in all the primary dystonias are few and findings are scarce. A report from four postmortem brains showed subtle changes in the midbrain and cerebellum [53], with absence of anomalies in other brain areas presumed affected in dystonias such as basal ganglia, thalamus, or cerebral cortex. Another postmortem study showed increased concentrations of copper and manganese in the putamen of dystonia patients [54]. Transcranial sonography of the lentiform nucleus of CD patients showed a slight hyperechogenicity contralateral to the head deviation in 9 of 10 subjects [55].
A VBM study reported that CD patients have increased gray matter volume in the thalamus, caudate head bilaterally, superior temporal lobe, and left cerebellum and decreased gray matter in bilateral putamen [34]. Trends toward significant changes were also seen in the globus pallidus, bilateral orbitofrontal cortex, SMA, medial frontal gyrus, and cingulate cortex [56]. Another study reproduced the left caudate and putamen findings and found additional changes in the bilateral premotor and primary sensorimotor cortices [57]. While no correlations were found with disease duration, severity, or age in a cross-sectional study, the left sensorimotor cortex was further reduced when patients were tested/retested 5 years after [57]. The different results between cross-sectional and longitudinal studies highlight the great degree of variability existent across subjects. Additional longitudinal studies of dystonia are needed, since they allow for more controlled evaluation of these changes.
White matter in patients also shows some alterations. Based on small ROI drawn in the white matter between putamen and thalamus, Blood et al. [58] reported that patients had more asymmetry than controls in the FA values. These changes were reversed after botulinum toxin treatment, suggesting the abnormalities were related to the symptoms and that the connection between basal ganglia and thalamus displays plasticity. Another study found no changes in FA, but reported increased MD in the white matter adjacent to pallidum, caudate, and putamen bilaterally in patients [59]. In an ROI analysis, another study found that FA in CD patients was significantly reduced in the body of the corpus callosum and bilateral putamen. MD was reduced in the right caudate, left putamen, bilateral prefrontal cortex, and left supplementary motor cortex [39]. ROI analyses, while less specific on the location, increase the power and compensate for variability between subjects, both in anatomy and in the manifestation of the dystonia, thus, providing more robust results. In line with the midbrain-cerebellum changes seen in postmortem brains, tractography showed altered connections between the pallidal output fibers and the brainstem [60]. There was a right/left hemisphere asymmetry in the tracts, in accordance with the asymmetric clinical manifestation of the disorder.
Using single-voxel proton magnetic resonance spectroscopy (1H-MRS) , basal ganglia NAA/Cr and NAA/Cho were reduced in patients [61]. NAA/Cr is a marker of neuronal integrity, and NAA/Cho a possible indicator of membrane dysfunction, suggesting, in this situation, loss of dopaminergic terminals or abnormal function of the striatal neurons. Because drugs that block dopamine receptors can produce dystonia, particularly of the head and neck, it is believed that the dopaminergic pathways can be involved in the etiology of the disorder. Indeed, an 11C-N-methyl-spiperone PET study showed that while there were no significant differences between healthy controls and patients, patients had a trend toward higher tracer uptake in the hemisphere contralateral to the symptoms [62]. Using 123I iodobenzamide SPECT, there were no differences in radiotracer uptake in basal ganglia between healthy controls and patients, but an interstriatal analysis showed that the basal ganglia contralateral to the direction of the torticollis had a higher binding to D2 receptors than the ipsilateral one [63]. A SPECT study compared the presynaptic striatal dopamine receptors, measured as 123I beta-CIT binding, with the postsynaptic dopamine receptors, measured with 123I-epidepride [64]. While the presynaptic receptor binding was similar for healthy controls and patients, the postsynaptic binding was lower in the CD group. The decreased postsynaptic D2 receptors could explain the lack of inhibition of the thalamo-cortical (indirect) pathway, and also explain the relative increased number of presynaptic receptors seen in other studies [63].
Patients’ glucose metabolism, measured with 18F-FDG PET, was increased in the basal ganglia-thalamo-cortical loop, including the basal ganglia, thalamus, premotor and motor cortex, and cerebellum [65], and bilaterally in sensorimotor areas when compared with controls [66]. A recent exploratory study showed that most of the sensorimotor differences were reverted when symptoms were alleviated following epidural premotor cortical stimulation [66]. These results are in line with the notion of CD being a disorder not only of the basal ganglia, but rather representing a more complex association with the sensorimotor network, including sensorimotor cortex, thalamus, and cerebellum.
fMRI studies have shown that nondystonic tasks impair activity in CD patients. As seen in blepharospasm patients, basal ganglia activity was altered during handgrip in CD patients. CD patients’ handgrip resulted in increased activity in basal ganglia, caudate nucleus, putamen, and thalamus [45]. On the other hand, patients’ movement imagery produced less activity than healthy volunteers in bilateral parietal, left premotor, and anterior cingulate cortices; movement execution showed impaired activation in the ipsilateral putamen, insula, and cingulate cortex [67]. As a response to passive movement of nondystonic areas, CD patients showed increased activation in primary and secondary sensory cortices contralateral to the stimulation, cingulate cortex, and bilateral cerebellum [68]. Several studies explored the correlation between botulinum toxin and fMRI activity. SMA activity in response to passive movements was positively correlated with botulinum toxin dosage and negatively correlated with the Toronto Western Spasmodic Torticollis Rating Scale (TWSTRS) score, suggesting a general disinhibition of the somatosensory system [68]. Similar results are seen during finger tapping, where SMA was abnormally hypoactive in CD patients, and improved toward normal after botulinum toxin treatment [69]. In response to median nerve stimulation, secondary somatosensory and insular cortices were less active in CD patients; however, these changes were reverted 4 weeks after botulinum toxin treatment [70]. These functional findings reinforce the notion of CD as a disorder of the sensorimotor system extending beyond the representation of the affected area. They also show that changes in symptoms, even induced peripherally by botulinum toxin, affect not only primary sensory areas, but also the secondary sensory association areas, SMA, and insula. The abnormalities in these areas are presumably not indicators of the underlying etiological pathology since they revert with botulinum toxin treatment.
Spasmodic Dysphonia
As in the other primary dystonias, there are no consistent radiological findings to explain SD , supporting the idea it is a heterogeneous voice disorder [71]. Similar to other primary dystonias, in addition to basal ganglia involvement, cortical contribution has been suspected. SD patients show changes in cortical thickness, gray matter volume and function in areas related to speech control, including the laryngeal sensorimotor cortex, inferior frontal gyrus, superior and middle temporal cortices, supramarginal gyri, and cerebellum [72]. In these patients, DTI findings indicate patients have decreased FA in the genu of the right internal capsule, a site confirmed in one histopathological examination, and increased MD bilaterally in the lentiform nucleus, ventral thalamus, and cerebellum. These changes correlated positively with severity of the clinical symptoms [73].
While no resting metabolism data are available in SD patients, there are several functional studies with PET and fMRI that found cortical, thalamic, basal ganglia, and cerebellar deficits. An early H2 15O PET case study suggested a reduction in SMA during phonation [74]. However, during speech-related activity, patients had hyperactivity in a network including the right dorsal precentral gyrus, cerebellar hemisphere and vermis, primary auditory cortex, anterior cingulate, and anterior insula [75]. The unimodal and heteromodal association areas, including the posterior supramarginal area and posterior middle temporal gyri and the dorsal postcentral gyrus, were hypoactive in the same patients [75]. These changes reverted after botulinum toxin treatment [75]. The improvement provided by the treatment correlated with increased sensorimotor activity and decreased activity in areas unrelated to motor control. The decreases in posterior auditory association cortex and the right ventral precentral gyrus and increases in SMA proper, heteromodal sensory areas (anterior middle temporal gyrus), and periaqueductal gray matter were not affected by the treatment, thus, considered traits of the disorder. During narrative whispering, increased activity was limited to the SMA proper. Treatment produced changes in the pre-SMA, right dorsal precentral gyrus, caudate, anterior putamen, ventral thalamus, pulvinar, cerebellar hemisphere, and vermis. Therefore, basal ganglia function is not particularly different between healthy volunteers and SD patients, but it is one of the areas where botulinum toxin treatment shows a marked effect.
SD patients showed increased extent of activation in the primary sensorimotor cortex, insula, and superior temporal gyrus during symptomatic and asymptomatic tasks and decreased activation extent in the basal ganglia, thalamus, and cerebellum only during asymptomatic tasks. The extent of activation in the primary sensorimotor cortex and ventral thalamus during a symptomatic task, and in the inferior temporal cortex and cerebellum during symptomatic and asymptomatic voice production, was greater in patients with the abductor form of dysphonia than for those with the adductor form [76].
All aspects of the speech control system [77], including unimodal and heteromodal cortices, basal ganglia, and SMA, have altered function and structure in SD. Pathways extending from the motor cortex to the midbrain are also affected.
Focal Hand Dystonia
Among all the dystonias, focal hand is the most studied one using imaging techniques. Most studies find deficits in the sensory and motor circuits. The gray matter volumes as assessed with VBM of the basal ganglia [78] and the sensorimotor cortices [79] of focal hand dystonia patients differ from those of healthy volunteers. Some of the structural changes are shared with other dystonia types. For example, the volume of the globus pallidus internus and the orbitofrontal cortex are less in dystonic patients than healthy volunteers, but these changes are not specific to focal hand dystonia [56]. Volume changes might be related to plasticity. Evidence for this is that the volume of the middle section of the putamen gray matter in pianists, with and without dystonia, covaries with the variability of their keystroke timing during scale playing. Patients have more variability, and the volume of the middle section of the right putamen is larger in patients than in healthy piano players [80].
Plasticity of the morphological changes is also observed in the white matter. FA values of the corticospinal tract were shown to be larger for musicians than nonmusicians [81], suggesting that practice can effect changes independent of disease. An ROI comparison of FA values of the small white matter region between the basal ganglia and thalamus showed left/right asymmetry in two patients with focal hand dystonia before treatment that reversed after botulinum toxin treatment [58]. Writer’s cramp patients had increased FA values bilaterally in the posterior limb of the internal capsule and adjacent structures, involving tracts connecting primary sensorimotor areas and subcortical structures [82].
Sensorimotor cortex and basal ganglia of patients were reported to have reduced levels of GABA measured with MRS [83]. This would be in accordance with the concept of reduced inhibition seen in electrophysiological studies [84]. However, the voxel size for GABA MRS is rather large (on the order of tens of cubic centimeters), making it difficult to detect signals when different tissues are part of the region of observation (Fig. 11.3). An attempt to confirm these findings in a second MRS study with improved technique observed only a trend [85]. MRS showed no differences in N-acetylaspartate/creatine or lactate/creatine in the lentiform nucleus [85, 86] or the sensorimotor area [85].
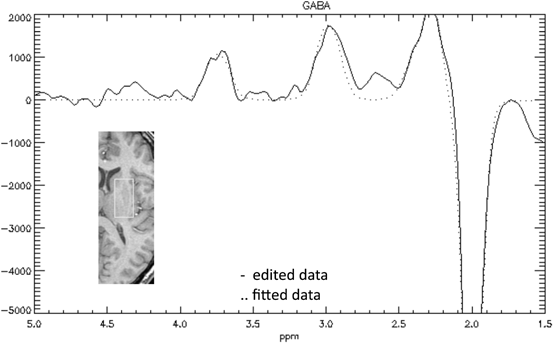
Fig. 11.3
Magnetic resonance spectroscopy. Example of the spectra for GABA (g (gamma)-aminobutyric acid) edited signal from a single subject (data from [85]). Inset brain section shows voxel location. Trace shows edited MRS with the GABA peak at 3.0 ppm, and the inverted NAA peak at 2.0 ppm
Several studies looked at CBF and metabolism during performance of tasks that generate or do not generate dystonic postures. During a writing task, writer’s cramp patients had impaired activation in the contralateral primary motor cortex and enhanced frontal activation. Improvements in writing, seen after botulinum toxin treatment, were accompanied by increases in parietal cortex and caudal SMA activations; however, the impairment persisted in the primary motor cortex [87]. In another study, the primary sensory cortex was overactive in writer’s cramp patients during a writing task. This change in rCBF, measured with H2 15O PET, correlated with subjective patients’ scores (Fig. 11.4). The activity in the primary sensory and motor cortices correlated with electromyography (EMG) activity during writing. The sensory changes suggested a possible dysfunction of sensory feedback [88]. When vibrotactile stimulation was applied to either arm, the rCBF response, measured with H2 15O PET, was decreased bilaterally in sensorimotor cortices and SMA, even though patients had unilateral symptoms. These changes did not depend on muscle cocontraction, since healthy volunteers had even larger responses when stimulation was applied during muscle contraction [89, 90]. Putamen rCBV measured with C15O, rCBF, and H2 15O show no functional alteration in patients; however, D2 binding measured with 18F-spiperone is decreased in patients [91]. Nevertheless, 18F-N-methyl-benperidol (NMB), a more selective D2 receptor that excludes serotonin receptors (5-HT2A), failed to reproduce D2 receptor changes [92]. These results indicate a complex mechanism for the disorder where there are subclinical brain deficits and unclear neurotransmitter alterations.
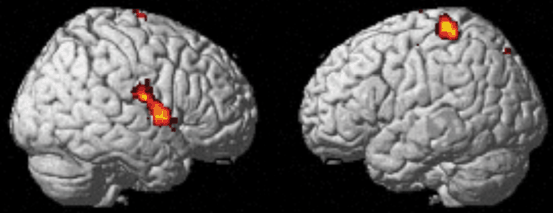
Fig. 11.4
[15O] H2O PET in focal hand dystonia patients (data from [88]). Regional cerebral blood flow in primary and secondary sensory areas correlates with patients’ subjective dystonia score
Several fMRI studies compared task performance and brain activations between healthy volunteers and focal hand dystonia patients. They found differences in brain activity even when performance was matched [93–95]. A comparison between guitar players with and without dystonia found the patients’ group to have increased activity in the contralateral sensorimotor cortex and decreased activity bilaterally in the premotor areas when performing hand movements, irrespective of whether the task triggered dystonia or not [94]. Extension or relaxation of the wrist resulted in decreased activation of the sensorimotor cortex and the SMA in writers’ cramp patients compared with controls, suggesting deficits both in inhibitory and excitatory mechanisms [95]. When patients tried to control an isolated finger movement, they had less activation in the primary sensory area, associative parietal areas, and right sensorimotor area of the cerebellum than when they were moving two fingers together (Fig. 11.5). These decreases were significantly more pronounced in patients than in healthy volunteers. Nevertheless, patients had increased functional connectivity between the left posterior putamen, left sensorimotor cortex, and left cerebellum. The left anterior putamen connectivity also differed in the bilateral SMA, bilateral motor cingulate area, left primary motor area, premotor cortex, and left cerebellum, suggesting a compensatory mechanism [93].
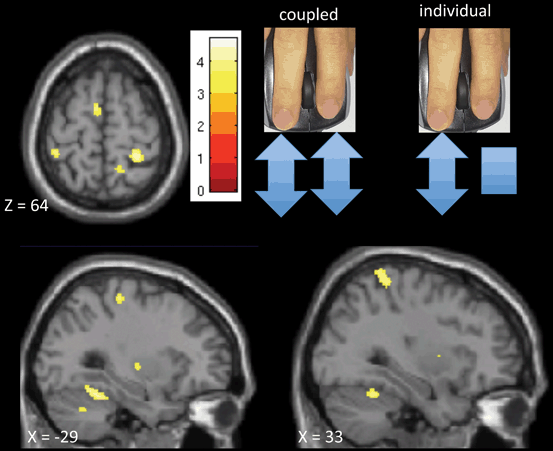
Fig. 11.5
fMRI study of focal hand dystonia (FHD) patients (data from [93]). Compared with healthy volunteer activations, FHD patients show widespread deficits when controlling individual finger movements compared with performing the same movement with both fingers. Healthy volunteers have more activation in the supplementary motor area (SMA) , cerebellum, bilateral putamen and bilateral sensory cortex when performing different tasks with each finger (individual task) than when both fingers do the same. Patients show less difference in these areas, suggesting lack of surround inhibition in the FHD
Somatosensory finger representation is less demarcated in patients than in controls. It differs between dystonic musicians and healthy nonmusician controls, with patients having smaller distances between individual finger representation [96]. Sensory representation of the fingers had lower spatial separation for patients; the order of the representation was also reversed in the secondary sensory cortex and posterior parietal cortex in patients compared with controls [97]. Another study showed that digits involved in writing (D1, D2, and D3) had smaller interdigit separation in area 3b and overlapping activity, while the distance for asymptomatic digits was preserved. The cluster location, closer to the symptomatic digits, suggested that dystonic symptoms could arise from reduced cortical spacing. In another study, sensory input elicited a lower response in patients’ area 3a [98]. Somatotopy is also affected in patients’ basal ganglia. Another fMRI study showed that, in patients, the representation of hand, lip, and toe movements was disorganized in the left putamen, contralateral to the affected side, and to a lesser extent, on the ipsilateral side [99]. Moreover, while the brain response to sensory stimulation of individual fingers predicts the activation from combined stimulation with a 12 % error for the healthy controls, prediction error is 30 % for patients, suggesting a nonlinear interaction [100].
Repetitive transcranial magnetic stimulation (rTMS) of patients’ primary sensory cortices can produce a subjective benefit in writing quality. In one study, these improvements were paralleled by bilateral increases in the blood oxygen level-dependent (BOLD) signal on fMRI in the primary sensory cortex, parietal cortex, and SMA. There were neither behavioral nor imaging changes after sham stimulation [101]. Basal ganglia also have abnormal responses to motor tasks. One study showed that after performing a motor task, BOLD signal in the motor area returned to baseline; however, the basal ganglia activation level remained elevated in patients [102]. In another study, basal ganglia and thalamus were hyperactive during tactile stimulation of the index finger of writer’s cramp patients, suggesting a deficit in the center-surround inhibition within their circuits. Basal ganglia and thalamus interaction is also affected in musicians with focal hand dystonia; they were active in healthy volunteers, but not in patients during finger tapping [103]. Basal ganglia and thalamic deficits may lead to compensation with augmented activations in cerebellum and cortical areas. This was seen in a study showing increased activity in the posterior vermis, right paramedian cerebellar hemisphere, and dorsal pons, which correlated with disease severity [104]. Patients also had hyperactivity in areas of the visual cortex, anterior insula, and intraparietal sulcus.
Several studies compared finger tapping in healthy volunteers and focal hand dystonia patients. One of them found that while cerebellum and contralateral sensorimotor and premotor areas were active in both groups, ipsilateral activation in the premotor area was larger in patients during tapping with the affected hand, and left cerebellar activation was reduced in patients when tapping with either hand [103]. Another study reported that the execution of simple or complex finger-tapping activates primary motor cortex, primary sensory cortex, SMA, left insula, and bilateral cerebellum. In this study, musicians and writer’s cramp patients activated the same network as controls, but to a lower level for both the symptomatic and nonsymptomatic hands [105]. To avoid confounds due to symptomatic task execution in focal hand dystonia patients, a couple of studies explored motor imagery of different hand movements in patients and healthy controls. One study compared imagery of grasping a pencil and writing with grasping the same pencil to sharpen it. Writing imagery produced larger activation than sharpening imagery in the left dorsal premotor area, an area involved in task planning. But, when the grasping was evaluated, healthy volunteers used more of the ventral premotor cortex, while focal hand dystonia patients relied on the dorsal premotor cortex [106]. A second study compared the imagery of writing from a kinesthetic perspective with the observation of a hand performing the movement subjects were asked to imagine. In patients, writing imagery produced an activation deficit of the left sensorimotor cortex, mesial SMA, and left premotor cortex, bilateral putamen, and bilateral thalamus when compared with the writing observation condition [107]. As in the other dystonias, focal hand dystonia affects structure and function of the components of the motor control circuit and the sensory networks. These alterations are observed as structural changes in white and gray matter. Functional changes are seen not only for tasks triggering dystonia, but also for a variety of tasks not related to the dystonia.
Conclusions and Future Directions
The basal ganglia, originally thought to be the sole origin of dystonia, can now be seen as one player in a more widespread dysfunction. Involvement of the cerebral cortex, in particular, sensorimotor, SMA, and parietal association areas, are consistent in all the primary dystonias. The cerebellum is also affected in all the dystonias discussed here. Unfortunately, there is a large variability of results found in imaging studies across groups and modalities. There are a number of reasons for this, including variation in patient characteristics, MRI hardware, and sequences employed; but, some results are also likely false positives due to small sample sizes. Till date, imaging has not produced a biomarker of dystonia, but has been helpful in expanding our understanding of the brain areas affected. Electrophysiology and imaging studies support the concepts of surround inhibition, compensation, and network imbalance as mechanisms for the disorder. Basal ganglia-thalamo-cortical, cerebellar-thalamo-cortical circuits, and the communication between sensory and motor areas seem to play key roles. What is not yet clear is which of the observed changes are the root causes of the dystonia and which ones are consequences. The ability to study manifesting and nonmanifesting carriers of the DYT1 gene or musicians with and without dystonia is helping to separate the plasticity that compensates for a deficit from the maladaptive plasticity that could result in dystonia. As noted at the outset, the main clinical role for neuroimaging in dystonia, at present, is to identify a secondary cause.
< div class='tao-gold-member'>
Only gold members can continue reading. Log In or Register a > to continue
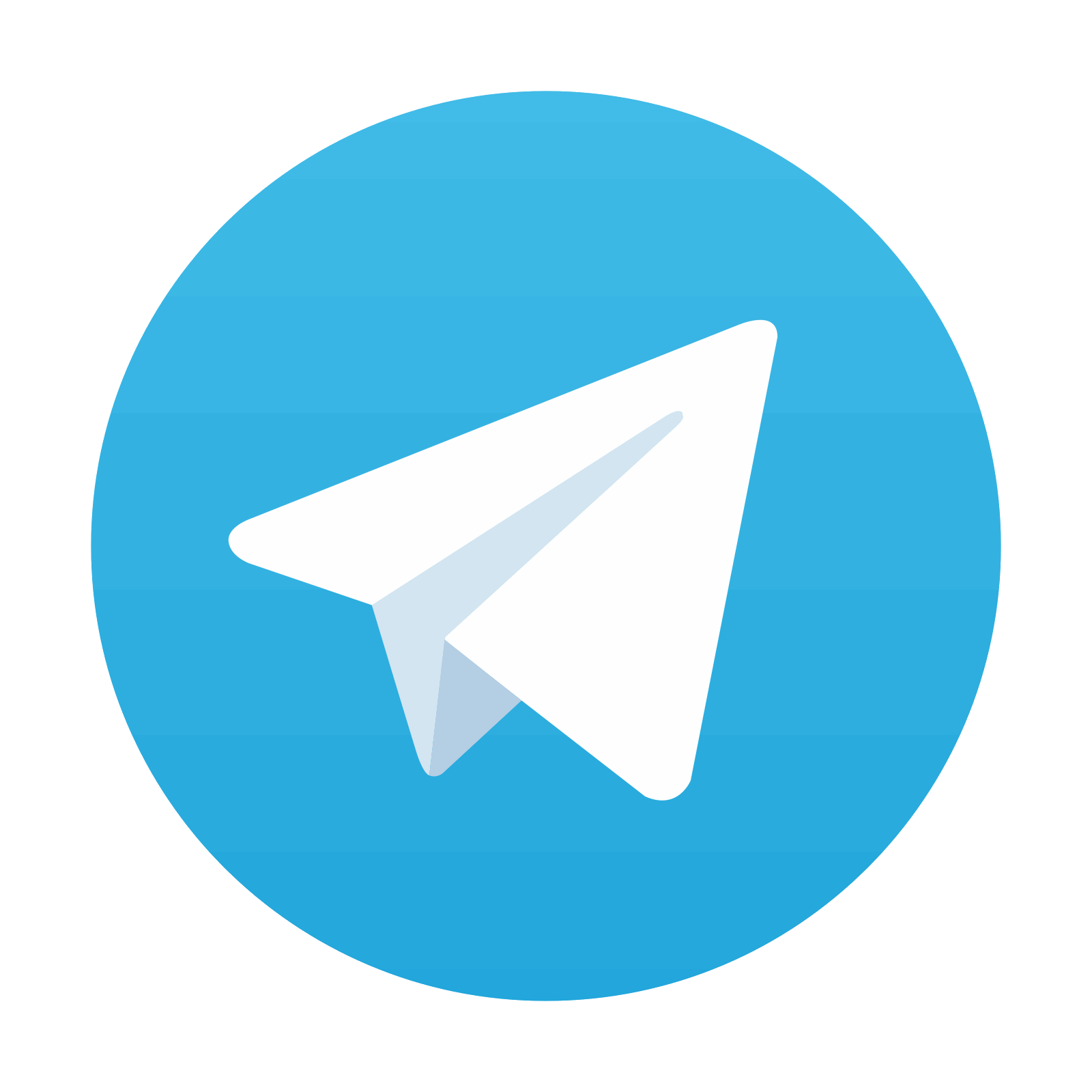
Stay updated, free articles. Join our Telegram channel

Full access? Get Clinical Tree
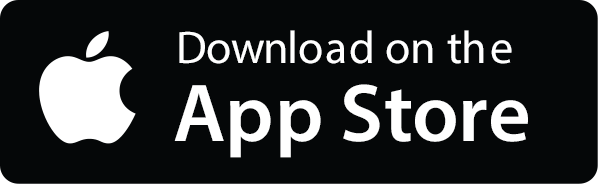
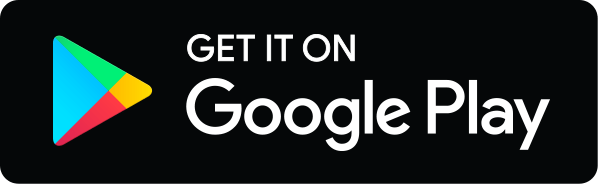