Fig. 11.1
Contrast reduction using dual energy CT coronary angiography. Single energy CTCA (SE-CTCA) performed with 75 ml of iodinated contrast (above): Axial image (a) at the ascending aorta showing a good intraluminal enhancement of 328 HU and image noise of 34 HU. Curved multiplanar reconstruction (MPR) of the left anterior descending (LAD) coronary artery depicting a normal vessel (b). Volume rendering of the heart and coronary arteries showing a normal coronary tree (c). Dual energy CTCA of the same patient performed with 35 ml of iodinated contrast (below): Axial image (d) at ascending aorta showing a good intraluminal enhancement of 304 HU and image noise of 19 HU. Curved MPR of the LAD depicts a normal vessel (e). Volume rendering of the heart and coronary arteries showing a normal study (f)
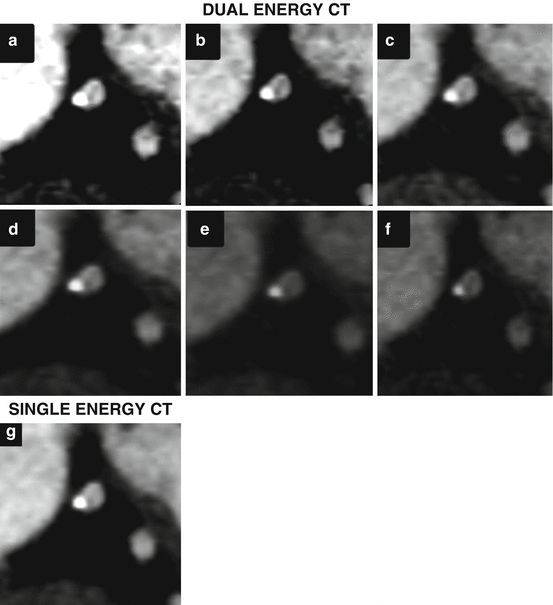
Fig. 11.2
Dual energy CT (DE-CTCA) versus single energy CT (SE-CTCA) in a 63 year-old male with suspected CAD. DE-CTCA at different energy levels and using a material decomposition approach. (a) 40 kev; (b) 50 kev; (c) 60 kev; (d) 70 kev; (e) 80 kev; (f) Iodine-water. DE-CTCA showing an orthogonal plane of the left anterior descending artery where a calcified plaque is identified at 9 o’clock. The plaque has greater volume at lower energy levels and is reduced at higher ones demonstrating a reduction in the blooming effect at higher levels. Iodine water shows the same plaque volume as monoenergetic imaging at 80 kev. (g) SECT at the same orthogonal plane shows the calcified plaque with similar size than 60 kev thus overestimating plaque size
With monochromatic information, two major artifacts that appear in SECT can be mitigated; the blooming and beam hardening artifacts:
Blooming artifact: At lower energy levels, the blooming artifact that is present in cases of severe calcification becomes worse (Figs. 11.2 and 11.3). Calcified plaque volumes are therefore overestimated, thus commonly leading to an underestimation of lumen area. For that reason it is very useful to read images acquired using higher energy levels. In general, the levels which best assess the degree of coronary stenosis in the presence of calcification are higher than 90 keV, although these levels are associated to significant reduction in luminal attenuation [18].
Fig. 11.3
Dual energy CT coronary angiography (DE-CTCA) of the same patient as Fig. 11.1 at different energy levels: (a) 40 kev; (b) 60 kev; (c) 80 kev; and using single energy imaging (SE-CTCA). DE-CTCA in an oblique axial plane shows a calcified plaque at the proximal left anterior descending artery (arrow). The plaque has greater size at 40 kev (a), mid size at 60 kev (b) and a reduced size at 80 kev (c). SE-CTCA (d) shows the calcified plaque with similar size as 60 kev
Beam hardening artifact (BHA): This artifact is associated with severe vessel calcification and high density anatomical structures [21]. It creates low density artifacts adjacent to calcified plaques. In some cases it is difficult to determine if the plaque has an associated non-calcified component or if there is a BHA present. Similar to the blooming artifact, BHA are reduced or canceled at higher energy levels (Figs. 11.2 and 11.3) [18, 22].
Overall, DECT with monochromatic evaluation allows reducing blooming as well as BHA. Both artifacts have a significant impact in stenosis quantification in SECT leading to a decrease in the positive predictive value in patients with diffusely calcified plaques [15, 23].
Material Decomposition
An interesting possibility for coronary artery evaluation is to use material decomposition. In this analysis materials can be selected or removed. For CAD two pairs of materials are proposed; iodine-calcium (Figs. 11.4 and 11.5) and calcium-iodine (Fig. 11.6).
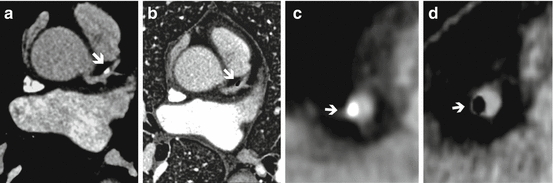
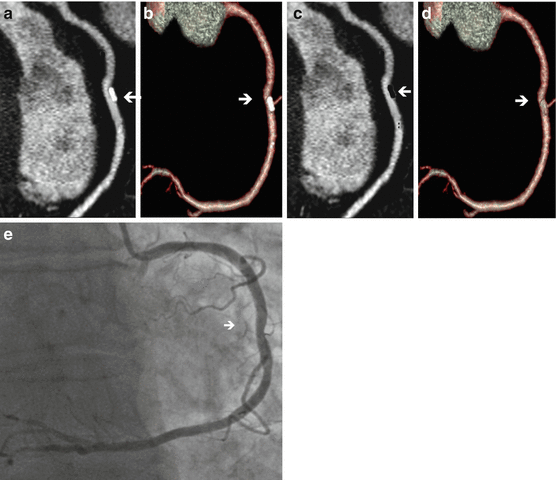
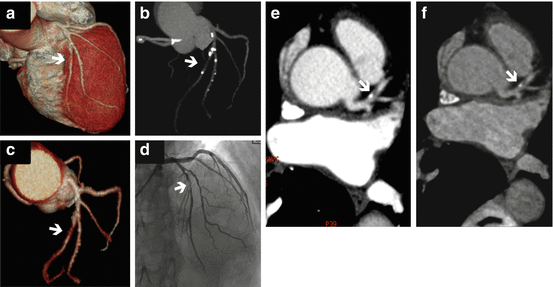
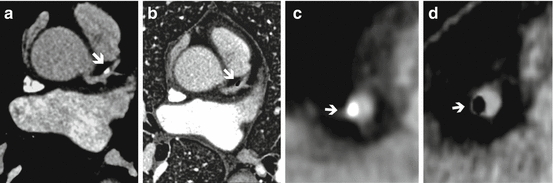
Fig. 11.4
Dual energy CT coronary angiography in monochromatic 60 kev and material decomposition iodine-calcium. (a) Axial image in 60 kev showing a calcified plaque at the proximal left anterior descending coronary artery (arrow). (b) Material decomposition iodine-calcium: the calcium is extracted and stenosis can be measured. (c) Orthogonal view of the calcified plaque. (d) Orthogonal view of iodine-calcium pair, where the calcified plaque is removed
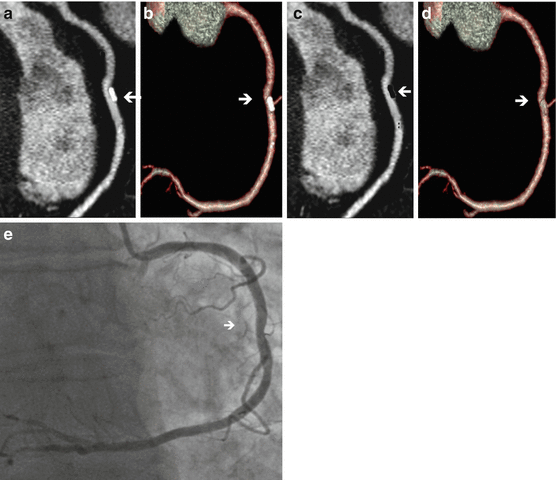
Fig. 11.5
Dual energy CT coronary angiography of the right coronary artery (RCA). (a) Curved multiplanar reconstruction (MPR) showing a calcified plaque in the mid RCA (arrow). (b) Volume rendering showing similar findings. (c) Curved MPR with material decompisition iodine-calcium where the calcified plaque is removed (arrow). (d) Volume rendering with plaque removal (arrow). (e) Invasive coronary angiography showing a minimal lesion at the mid RCA where the plaque is present
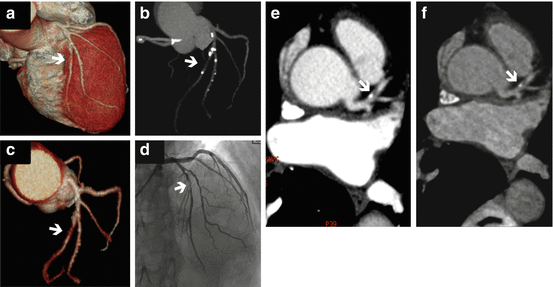
Fig. 11.6
Dual Energy CT coronary angiography to measure coronary stenosis and virtual non-contrast imaging. Volume rendering of the heart (a) showing a severe stenosis at the mid left anterior descending coronary artery (LAD) due to mixed plaque, mostly non-calcified (arrow). Maximum intensity projection (b) and volume rendering of the coronary tree (c) demonstrating the same lesion. Invasive coronary angiography confirming the findings (d). Axial view showing a mixed plaque in the proximal LAD (arrow). (f) Same axial view with material decomposition calcium-iodine (virtual non-contrast) showing only the calcium
In the iodine-calcium pair, calcium is removed from the vessel wall and iodine is maintained. This subtraction allows performing a more precise quantification of the stenosis where the calcified plaque is present. This tool has to be complemented with monochromatic evaluation of the vessel at different energy levels, preferably at mainly higher ones (≥80 keV) which reduce blooming and beam hardening artifacts giving a more precise final assessment. Coronary arteries are the most challenging site to perform this subtraction, whereas other vessels such as aorta and great branches are easier territories. In calcium-iodine pair, iodine is removed from the vessel lumen and calcified plaques are kept (virtual non-contrast). Accordingly, this approach has the potential to calculate the coronary artery calcium score during the same scan, thus providing a significant incremental prognostic value to the contrast-enhanced CTCA without requirements of an additional non-enhanced scan acquisition [24, 25].
Evaluation of De Novo Lesions
Numerous studies have explored the role of CTCA for the detection of obstructive CAD [23, 26, 27]. Overall, these studies have demonstrated that CTCA has a high diagnostic accuracy to detect obstructive CAD in different populations, although the best scenario at least for conventional SECT seems to be patients at intermediate risk. Indeed, a number of multicenter studies confirmed these findings, with sensitivities ranging between 85 % and 95 %, specificities between 83 % and 90 %, and similar accuracy compared to invasive angiography regarding the ability to predict short term revascularization. Despite slight differences in study design and populations, there is robust evidence that positions CTCA as the non invasive diagnostic tool with the highest negative predictive value [23, 26, 27].
A recent large multicenter registry (CONFIRM) that included 15,207 patients at intermediate risk of CAD who underwent CTCA demonstrated that patients without CAD and those with only mild disease had low rates of need of invasive angiography (2.5 % y 8.3 %, respectively), and very low revascularization rates (0.3 % y 2.5 %, respectively), after a 2.3 year follow-up. On the contrary, patients with obstructive lesions showed significantly higher rates of invasive angiograms and revascularization, with increments from one-vessel disease (44.3 % y 28.0 %, respectively), two-vessel disease (53.3 % y 43.6 %, respectively), and three-vessel disease (69.4 % y 66.8 %, respectively) [28]. These findings support the role of CTCA as an effective gatekeeper to invasive coronary angiography.
Notwithstanding, although CTCA has shown a relatively high diagnostic accuracy in most clinical scenarios, it does not provide a significant incremental value over functional tests in patients with high pretest probability [29].
This limitation of the technique appears to be at least in part related to the fact that such high risk patients usually have diffusely calcified vessels. Indeed, despite the incorporation of novel technologies in the field such as double source CT, scanners with larger coverage, detector technology, and faster gantry rotation speed, has greatly improved temporal and spatial resolution, diffuse calcification endures as the variable with the greatest impact in the diagnostic accuracy [15].
Several studies have shown a significant drop in the diagnostic accuracy in patients with a calcium score (Agatston) higher than 400, being this attributed mainly to the blooming artifact previously described that leads to overestimation of the degree of luminal encroachment [26, 30].
In a post-hoc analysis of the CORE-64 study, the evaluation of non-calcified segments yielded a positive likelihood ratio (LR +) of 34.4 (95 % CI 23.1, 51.2), compared to a LR+ of 9.9 (95 % CI 7.5, 13.1) among mildly calcified segments, a LR+ of 4.3 (95 % CI 3.3, 5.5) among segments with moderate calcification, and a LR+ of 2.8 (95 % CI 2.2, 3.5) among severely calcified segments, respectively; clearly exposing the negative impact of coronary calcification in the diagnostic accuracy of CTCA. Furthermore, the same study reported that while 16 % of segments with mild calcification were deemed as with poor image quality, 43 % of segments with severe calcification reached such qualification [31].
The role of DECT imaging for the evaluation of de novo lesions seems to be related to the potential incorporation of a wider population within the scope of the technique. Such statement is supported, at least theoretically, by the aforementioned ability of DECT to attenuate the adverse effect of beam hardening and blooming artifacts, thereby potentially aiding the difficult assessment of severely calcified vessels (Figs. 11.2, 11.3, and 11.5) [21, 22, 32, 33].
There are limited publications showing the potential of DECT for the assessment of CAD. However, initial results are very promising. A recent study demonstrated a significant reduction of beam hardening artifacts that result with standard SECT by using higher monoenergetic energy levels with cardiac DECT [22]. How this more confident evaluation of the myocardium will enable the evaluation of higher risk patients is not known but the potential to improve CT perfusion assessment is real. The ability of DECT to reduce beam hardening artifacts (BHA) is attributed to the polychromatic nature of x-rays and the energy dependency of x-ray attenuation [34]. Accordingly DECT imaging might also have improved diagnostic performance compared to conventional CT for the assessment of myocardial perfusion, preserving image quality and without increment in effective radiation dose levels. This might be of interest towards the further functional assessment of patients with intermediate to high probability of CAD with stress myocardial perfusion CT.
At the Society of Cardiovascular Computed Tomography (SCCT) 2012 meeting, a prospective study performed in 39 patients who underwent both DECT and SECT was presented that deserves a comment. Thirty coronary plaques were selected (ten calcified, ten mixed, and ten non-calcified plaques) for analysis. The readers measured lumen area adjacent to the plaque at the area of maximal stenosis, as well as plaque area, lumen diameter, and vessel diameter. Luminal stenosis decreased with increasing monochromatic energy levels. However, plaques were largest at 40 kev and smallest at 140 kev, with modest modifications at 100 kev and greater. The largest difference was observed from 40 to 80 kev, where it got significantly smaller, whereas above 80 kev it didn’t modify significantly. Compared to SECT, the 140-keV monochromatic images at DECT showed a 21 ± 11 % greater lumen area and an 11 ± 7 % greater luminal diameter. The calcified plaque area was reduced by 26 ± 11 % and plaque diameter decreased 15 ± 9 % (p < 0.05) compared to SECT. Stenosis percentage was 25 % lower on the DECT than it was on SECT. Authors concluded that DECT could have an important role in CAD, since it might lead to a reclassification of the severity of lesions [35].
Another study conducted by Scheske et al. assessed the ability of single-source rapid kilovolt peak-switching dual-energy to reduce BHA in CTCA compared to SECT, and attempted to define the optimal monochromatic-energy levels for coronary evaluation. Coronary and myocardial signal-to-noise ratio (SNR), contrast-to-noise ratio (CNR), and iodine concentration were measured at different segments and monochromatic energy levels ranging from 40 to 140 keV. BHA was present at SECT with basal inferior wall mean reduction of 19.7 HU ± 29.2 (standard deviation) and mid-septum increase of 46.3 HU ± 36.3. DECT promoted a reduction in this artifact at 90 keV or greater (1.7 HU ± 18.4 in basal inferior wall and 20.1 HU ± 37.5 in midseptum at 90 keV; p < 0.05). Authors concluded that DECT with rapid kVp switching results in reduction of BHA and improvements in SNR and CNR in the myocardium and coronary arteries [22].
Plaque Characterization with DECT
The potential role of DECT for the assessment of plaque characterization deserves consideration. Thin-cap fibroatheroma lesions, the most common substrate of plaque rupture and acute thrombotic coronary occlusion have been the target of vulnerable plaque imaging during the past decade [4, 7, 12, 13]. The high prevalence and the uncertain natural history of high risk coronary plaques have led to disappointing results of invasive imaging tools regarding the identification of lesions likely to cause clinically meaningful events [11, 36, 37]. For these reasons, as well as due to the fact that invasive imaging aims only at secondary prevention, several investigators have begun to explore the potential role of non-invasive imaging and particularly of CTCA for the assessment of vulnerable plaque [38–40].
Briefly, CTCA has the capability to identify several characteristics associated to high risk plaques such as positive remodeling (which has been systematically and positively associated to the size of the underlying necrotic core and therefore provides indirect data regarding the underlying plaque composition) [41–44], spotty calcifications [40], and characteristics related to thin-cap fibroatheroma such as the napkin-ring sign and low-attenuation plaques [38, 39, 45].
The potential role of DECT for the assessment of plaque characterization seems to be related to the fact that using x-rays at different energies impacts the attenuation values of key plaque components such as fibrous tissue and necrotic core. The hypothetical basis of this concept stands on the fact that tissue attenuation levels are related to both the physical properties of the plaque components (atomic number and density) and by the delivered x-ray energy. DECT imaging has been shown to discriminate the composition of kidney stones and tumor characterization [46, 47].
A number of ex vivo and in vivo studies have explored the diagnostic accuracy of DECT versus SECT imaging for plaque characterization, yielding conflicting results [48–50].
A recent study using CTCA at two different energy levels (100 and 140 kV) has shown to improve the sensitivity and specificity for identifying necrotic core ex vivo. Nevertheless, the diagnostic accuracy in vivo for the detection of necrotic core was suboptimal and DECT images were of lower quality than SECT images, although DECT showed a modest improvement compared to SECT in terms of diagnostic accuracy [51]. Further studies are warranted to explore the application of motion correction algorithms (MCA) that are described below in order to improve the temporal resolution of DECT, that has been identified as one of the main limitations of DECT for plaque characterization in vivo [51, 52].
Interestingly, Tanami et al. showed that the diagnostic performance of CT analysis for ex vivo plaque characterization was superior at lower energy settings (80 kV), with an area under the curve at ROC analysis for the discrimination between lipid-rich and fibrotic plaques of 0.81 for 80 kV, 0.77 for 100 kV, 0.68 for 120 kV, and of 0.65 for 140 kV. Furthermore, the dual energy index, or ratio of the CT attenuation value at the 80 kV setting divided by the 140 kV setting (Hounsfield ratio [HR], 80:140) seems to emerge as potential tool for plaque classification, with an area under the curve of 0.95 [49].
Further studies exploring the potential role of non-invasive spectral imaging of coronary atherosclerosis by DECT are warranted in order to address whether this approach might help unravel the characterization of non-calcified plaques, particularly the discrimination between fibrous tissue and necrotic core [53].
Role of CTCA in Patients with Acute Chest Pain
In the US alone, more than seven million patients are admitted every year with acute chest pain suspected of acute coronary syndromes (ACS), and are evaluated for approximately 24 h including serial ECGs, enzyme level assessment, and eventually the performance of functional tests. This scenario represents a large economical burden for the US health system. Furthermore, almost 5 % of patients with ACS are not recognized by standard diagnostic algorithms, potentially leading to serious legal implications [54].
During the past decade, CTCA has emerged as a useful tool for the triage of patients with acute chest pain with low to intermediate probability of CAD. This has been supported by three randomized multicenter studies (CT-STAT, ACRIN-PA, and ROMICAT II) that positioned CTCA as a safe strategy that allows a significant reduction in diagnostic time, leading to a larger percentage of patients with discharge from the emergency department (ED). Moreover, this was achieved without an increase in costs [55–57].
In the CT-STAT trial, a randomized study that included 749 patients with low-risk acute chest pain, despite a similar diagnostic performance was observed between the two strategies, patients assigned to CTCA had a significant reduction in diagnostic time (54 %; 2.9 h vs. 6.2 h; p < 0.001) and in total cost (38 %; USD 2.137 vs. USD 3.458, p < 0.001) [55]. In the ACRIN-PA trial, that randomized 1,370 patients with low to intermediate risk to CTCA or standard of care, CTCA was associated to significantly larger rates of discharge from the ED (50 % vs. 23 %, p < 0.001), and to a significantly lower number of unnecessary (false positive) invasive angiograms (p < 0.05). Furthermore, it is noteworthy that of the 640 patients with a negative CTCA, none suffered an adverse event after 30 days of follow-up [56]. Finally, in the ROMICAT II trial, that randomized 1,000 patients with acute chest pain and no ischemic ECG changes to CTCA or standard of care, patients assigned to CTCA showed a significant reduction in diagnostic time (5.8 h vs. 21.0 h; p < 0.001), and a significant increase in the percent of patients with discharge from the ED (47 % vs. 12 %, p < 0.001). It should be stressed that in this study no differences were observed regarding costs, despite as pre-specified in the protocol diagnostic tools within the control group were used at discretion of the treating physician [57]. A recent meta-analysis of studies that explored the role of CTCA in the triage of patients with acute chest pain unit concluded that CTCA is a safe strategy and improves patient management in the ED, cutting down costs and hospital admission rates [58]. Furthermore, acute chest pain patients with negative or mild CT findings can be safely and promptly discharged from the ED without further testing, and with excellent 30 day event-free survival [59]. Overall, the robust favorable aforementioned evidence has lead to the integration of CTCA is different guidelines of clinical practice for the care of patients with acute chest pain [60–62].
The role of DECT in the triage of patients with acute chest pain is not only related to its capacity to identify obstructive CAD as accurately as conventional CT, but probably more interestingly, to the potentially more dedicated ability of DECT to evaluate myocardial perfusion assessment without beam hardening artifact.
Recently, Weininger et al. prospectively compared the diagnostic accuracy of adenosine stress-rest myocardial perfusion CT versus adenosine-stress first-pass DECT myocardial perfusion for the assessment of acute chest pain in patients who were clinically referred to stress/rest SPECT and cardiac MRI. Patients were randomly assigned to underwent adenosine-stress dynamic real-time myocardial perfusion CT using a novel “shuttle” mode on a second generation dual-source CT (group A), or adenosine-stress first-pass dual-energy myocardial perfusion CT using the same CT scanner in dual-energy mode (group B). CT findings were compared with MRI and SPECT. In Group A 149/170 myocardial segments (88 %) could be evaluated whereas in group B 100 % of myocardial segments were available for analysis. Real-time perfusion CT (versus SPECT) had 86 % sensitivity, 98 % specificity, 94 % positive predictive value, and 96 % negative predictive value in comparison with perfusion MRI for the detection of myocardial perfusion defects. DECT myocardial perfusion (versus SPECT) had 93 % sensitivity, 99 % specificity, 92 % positive predictive value, and 96 % negative predictive value for detecting hypoperfused myocardial segments. Authors concluded that myocardial perfusion CT imaging is useful for the triage of patients with acute chest pain compared to MRI and SPECT, and that DECT showed greater number of assessable segments compared to the dynamic mode [63].
Another paper performed by Wang et al. evaluated the incremental value of dual-energy CT over CTCA for the detection of significant coronary stenosis compared to quantitative coronary angiography (QCA) and SPECT. Thirty-four patients were studied with DECT angiography (DE CTA) and DECT perfusion (DE CTP). DE-CTA showed a sensitivity of 82 %, specificity of 91 % and accuracy of 86 % for detecting ≥50 % coronary stenosis on the vessel-based analysis compared to QCA. DE-CTP had sensitivity of 68 %, specificity of 93 %, and sensitivity of 81 %, and specificity of 92 % for identifying any type of perfusion deficits on the segment- and territory-based analysis, respectively compared to SPECT. The combination of DE-CTA and DE-CTP resulted in a sensitivity of 90 %, specificity of 86 % and accuracy of 88 % for detecting ≥50 % coronary stenosis, respectively. Authors concluded that the combination DE-CTP and DE-CTA may improve diagnostic performance compared to CTA alone for the diagnosis of significant coronary stenosis [64].
Evaluation of Coronary Stents
The assessment of coronary stent patency by CTCA is somewhat limited compared to the assessment of de novo lesions, being this mainly attributed to high attenuation artifacts such as BHA, partial volume effect, motion artifacts, and blooming effect related to stent material, and to the lower spatial resolution compared to invasive angiography. Indeed, with earlier scanner generations (≤64 row) the rate of non-evaluable stents ranged between 3 % and 24 % [65]. However, several studies demonstrated a reliable diagnostic performance of CTCA for the detection of in-stent-restenosis (ISR), particularly of stents ≥3 mm in diameter [66–68]. Stent assessment has significantly improved with the advent of third (64-row) and forth (>64 row) generation scanners, and depends also on the strut thickness, strut cell type, and stent material [69, 70]. It is noteworthy that stent lumen visibility is greatly influenced by stent material, with a 90 % lumen visibility within magnesium stents, and a 50–59 % visibility within the most common steel, cobalt-chromium, or tantalum [70].
Blooming is probably the most important issue in coronary stent imaging. It is mainly related to metal artifacts and the partial volume averaging effect. In the presence of blooming the stent struts appear to be thicker, causing an underestimation of the stent lumen of up to 30 % [71].
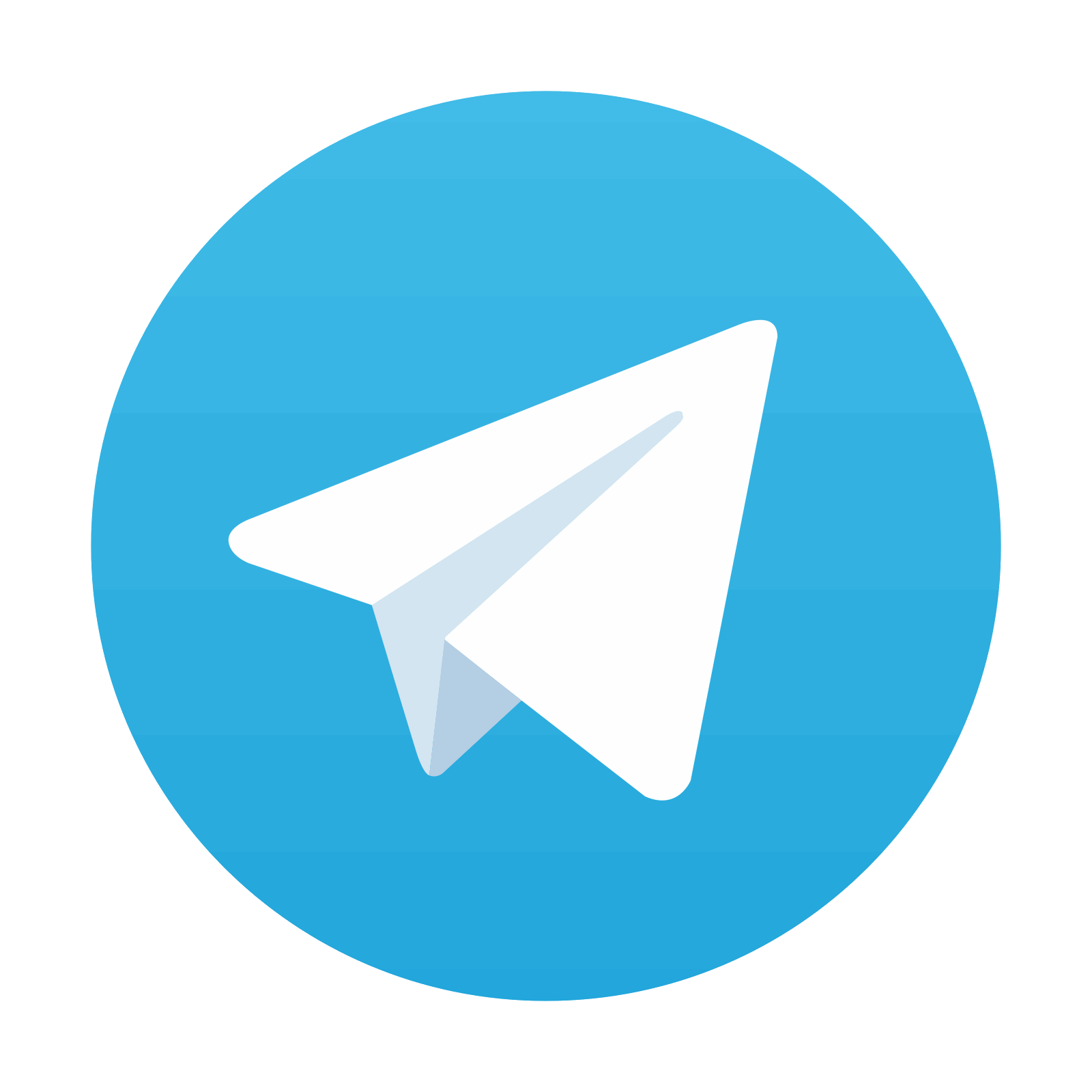
Stay updated, free articles. Join our Telegram channel

Full access? Get Clinical Tree
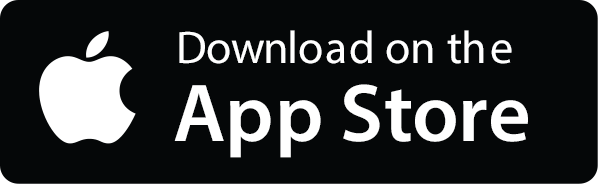
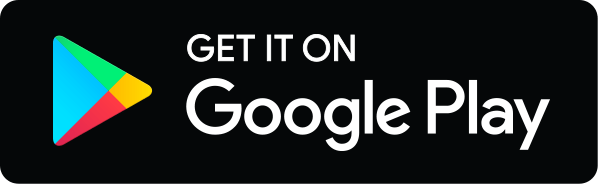