Fig. 1
Clinical CT scans and 3D reconstructions of human hearts displaying high spatial resolution and anatomical features of cardiovascular disease . (a) Cross-sectional CT image of the heart. White arrow indicates regions of calcified plaques in the coronary artery. (b) CT scan showing region of non-calcified plaques in the right coronary artery. (c) 3D reconstruction of the heart from CT images, with the left arterial descending (LAD) coronary artery in proximal view
4 Contrast Agents for CT
CT contrast agents play a key role in cardiovascular CT scans; the use of contrast agents in CT can improve the delineation between different soft tissues and is critical for highlighting blood vessels. The primary mechanism for X-ray attenuation in CT is the photoelectric effect. At CT energy levels (25–140 keV), incoming X-rays have enough energy to interact with and eject inter-shell electrons (k or l shell) of the atom. Ejection of a k-shell electron produces a significant increase in X-ray attenuation and allows for increased separation between tissue and contrast media. For this reason, CT contrast agents are typically high atomic number elements such as iodine, barium, gold, bismuth, and lanthanides that have l and/or k edges that are at much higher energies than tissue and are closer to diagnostic X-ray energies. However, CT suffers from low sensitivity to contrast agents, typically requiring millimolar local concentrations to produce contrast that can be distinguished from the background [57].
Barium sulfate is an agent used for imaging the gastrointestinal tract. The agent is typically swallowed and allows visualization of the digestive system [58]. While barium sulfate is limited to imaging of the GI tract, iodine based agents are widely used intravenously for a variety of applications. Iodinated contrast agents (ICA) can be used to visualize the vasculature as well as the organs such as the brain, heart, liver, and kidneys [59–61]. Clinical ICA are small molecules that are based on a tri-iodinated benzene ring. These types of structures reduce osmolality and toxicity compared with injections of iodide ion based agents. These iodinated rings are typically functionalized with amides and alcohol groups to provide water solubility, and can be monomers or dimers. These agents can be further categorized into ionic and non-ionic formulations which yield different properties in terms of toxicity and clearance from the blood stream. Regardless of the molecular structure of these agents, clinically approved ICA suffer from rapid renal clearance from the circulation after intravenous injection, on the order of minutes [62]. Given this rapid clearance, large amounts of contrast agent are often used for prolonged or multiple CT scans, with doses of 150 ml being used routinely [63]. Injecting large volumes of ICA increases the chance of severe renal impairment, known as contrast induced nephropathy , in specific patient populations [64]. Contrast-induced nephropathy is more likely in patients with preexisting renal dysfunction [65], who are now 26 % of the US population over 65 [66]. This proportion is expected to increase in the coming years, since renal dysfunction is common in diabetics and the prevalence of diabetes is predicted to rapidly rise [67, 68]. Iodinated contrast agents also can commonly cause adverse reactions in patients ranging from mild symptoms including nausea and vomiting to severe reactions such as anaphylaxis [69].
These issues with agents have created interest in developing new contrast agents for CT. Nanotechnology has been the primary source of new contrast agents for CT , since nanoparticle platforms allow for increased payload delivery, longer circulation times, targeted agents, and straightforward integration of multiple properties (e.g., drugs or additional contrast media) [70–74]. New agents are also being sought that have less adverse effects than current clinical agents. Researchers are able to control the size, shape, and surface properties of nanoparticles , which can drastically alter the biological interactions of these agents. For instance, while small molecules are rapidly cleared through renal filtration, a nanoparticle can be precisely synthesized to a size >10 nm, reducing renal clearance and therefore increasing circulation time. Additionally, control of the surface properties of the particle can allow for increased circulation time [75–78], or specific targeting to tissue [79–81]. With control over these properties, nanotechnology offers an effective solution to designing contrast agents with desirable properties for specific applications.
5 Nanoparticles for CT Imaging of Cardiovascular Disease
5.1 Iodinated Contrast Agents
As mentioned, iodinated small molecule contrast agents are the most widely used class of agent in CT scanning. While different formulations (ionic vs non-ionic, dimer vs monomer) have slightly differing biocompatibility properties, they continue to suffer from rapid clearance and risk of adverse reactions [69]. Researchers have turned to nanotechnology to improve the properties of these iodinated agents. Incorporation of iodinated agents into nanoparticles such as nano-emulsions , micelles , liposomes , dendrimers have resulted in improvements in circulation time and biocompatibility. The structures of some of the nanoparticle agents used as CT contrast agents are displayed in Fig. 2. Long blood circulation time of contrast agents may help to improve the assessment of cardiovascular health. A long circulating agent also can decrease the total dose necessary for an imaging procedure, potentially reducing the burden of the agent on the patient. The use of a blood pool contrast agent for CT may provide information about coronary artery disease and progression, allowing visualization of the extent of stenosis in an artery and well as calcified and non-calcified plaques [25]. Use of a contrast agent also allows for assessment of cardiac functions such as ejection fraction and stroke volume [43]. In addition, contrast agents are needed to highlight blood vessels during stent placement procedures.
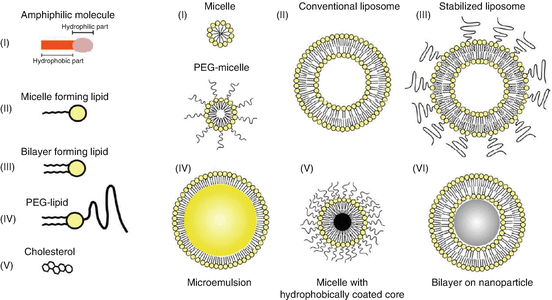
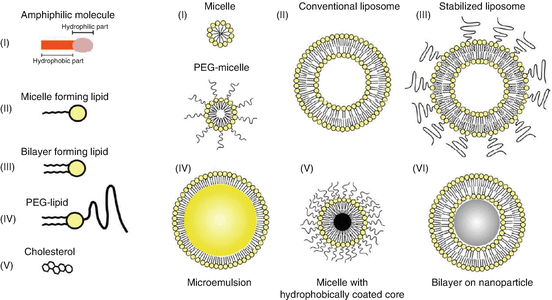
Fig. 2
Schematic representation of nanoparticle platforms. PEG functionalized nanoparticles are less likely to be taken up by cells of the mononuclear phagocytic system, leading to longer circulation half-lives. Reproduced with permissions from Ref. [82]
To incorporate iodinated agents into nanoparticles , nano-emulsions have been formed with a hydrophobic core of iodinated oil. Nano-emulsions can be formed via simple synthetic methods , which produce stable iodinated particles and have been an attractive choice for iodinated contrast agents. An emulsion is formed with a suitable amphiphilic surfactant encapsulating an iodinated oil core. An iodinated oil called Lipiodol (also known as Ethiodol ) is FDA approved and consists of iodine combined with ethyl esters of poppy seed oil [83]. Because Lipiodol is hydrophobic, it has limited clinical uses (it is used as an agent for lymphangiography and trans-arterial chemoembolization) [84, 85]. To expand the applications of Lipiodol as a blood pool agent, Pluronic F127 and a polyethylene glycol (PEG) diamine were used to encapsulate Lipiodol into a nano-emulsion [86]. These particles, 150 nm in diameter, were shown to be thermodynamically stable and produce vascular CT contrast in the ventricles and major arteries for up to 4 h.
Nano-emulsions have also been reported where a mixture of phospholipids and cholesterol was used to surround the hydrophobic core, which consisted of iodinated triglycerides. A nano-emulsion formed with endogenous oils and lipids was demonstrated as an effective contrast agent for hepatic imaging and is available as a commercial product known as Fenestra LC® [87, 88]. The iodinated triglycerides making up the core of this nano-emulsion are rapidly metabolized by the liver [89]. To provide longer circulation as a blood pool contrast agent , the agent has been reformulated with PEG included at the surface of the particle to reduce uptake by hepatocytes, which is known as Fenestra VC® [90, 91]. As a blood pool agent, Fenestra VC® has been used to study cardiac function in mice, quantifying ejection fraction, stroke volume, and cardiac output [92]. In this study, Fenestra VC® provided vascular contrast for 3 h with a nearly 500 HU value difference between the blood and myocardium. Moreover, with the use of Fenestra VC® , Detombe et al. studied cardiac function and ventricular remodeling in a mouse model of myocardial infarction. The group was able to assess systolic and diastolic volumes and ejection fraction noninvasively over the course of 4 weeks after myocardial infarction [93]. Both studies utilized cardiac gating with micro-CT to quantify cardiac function accurately.
While Fenestra formulations have been used successfully for cardiac applications, relatively poor inclusion of iodine by weight means that very large volume administrations of the agent are required (up to 40 % of total blood volume). To overcome this issue, researchers have used denser iodinated oils to improve iodine content in the formulations. Using, a dense tri-iodinated oil, de Vries et al. formed nano-emulsions using the amphiphilic polymer, poly(butadiene)-b-poly(ethylene oxide) (PBD-PEO) [94]. The agent was able to provide strong vascular contrast in the chambers of the heart of up to 220 HU directly after administration. Attenuation of the liver and spleen remained at high levels over the three hour observation period suggesting the mononuclear phagocytic system as the main clearance pathway for the agent. Novel approaches to increasing the density of iodinated oils continue to be explored [95, 96]. For instance, Attia et al. reported agents that contain up to 65 % wt. of iodine via use of a hexa-iodinated monoglyceride and a PEGylated nonionic surfactant [91]. This formulation showed clear delineation of the major arteries and heart chambers post-injection with a long circulation half-life of 6.1 h.
Micelles are similar to nano-emulsions , in that they are self-assemblies of amphiphilic molecules where the hydrophilic headgroups point outwards and the hydrophobic tails point inwards (Fig. 2i). The main difference between emulsions and micelles is that there is not an oil core for micelles. Therefore, as CT contrast agents , iodinated molecules are typically linked to the hydrophobic tails of the amphiphiles, concentrating the iodinated moieties into the center of the micelle. This allows for encapsulation of high iodinated payloads [97]. There have been several reports of iodinated micelles used as blood pool contrast agents [98]. Torchilin et al. reported the synthesis of micelles using iodine containing poly-l-lysine (MPEG-iodolysine). The micelles were approximately 80 nm in diameter with 33.8 % iodine content. The agent was injected intravenously and allowed for CT imaging of the aorta, heart, liver, and spleen for up to 3 h in rats [99].
While nano-emulsions and micelles can carry hydrophobic contrast media, liposomes are versatile platforms that can carry both aqueous and hydrophobic payloads. Liposomes are synthesized through the use of amphiphilic lipids or polymers that self-assemble into bilayers . This architecture allows for encapsulation of hydrophilic agents in the core as well as hydrophobic agents in the bilayer. One of the first liposomal iodinated contrast agents was synthesized using l-α-phosphatidylcholine and was loaded with a number of different aqueous iodinated agents [100]. The agent was injected intravenously as a blood pool contrast agent. Uptake of the liposome was seen in the liver and spleen in a dose dependent manner with maximum attenuation of 240 HU seen at 1 h. Because these early liposomes provided strong contrast of the liver and spleen, it was primarily used to visualize tumors in these organs [101, 102].
With improvements to liposome design, most notably the modification of amphiphilic lipids/polymers with PEG , the diagnostic applications of liposomal contrast agents expanded [103]. The addition of PEG to liposomal formulations allows for significantly increased blood circulation times improving their utility as a blood pool agents. One of the earliest reports of PEGylated liposomes, PEG-phosphatidylethanolamine (PEG-PE) liposomes, found that the addition of PEG increased the blood time circulation to up to 5 h as compared to 30 min for non-PEGylated formulations. Iodinated PEGylated liposomes have been successful in providing strong vascular contrast of up to 900 HU in the aorta, as reported in a study by Mukundan et al. [104–106].
With the use of liposomes, a significant issue is the leakage of internal payloads through the lipid bilayer. Hydrophilic payloads have been found to diffuse through bilayers due to chemical gradients. To overcome this issue, some groups have added cholesterol to liposome formulations, which reduces the permeability of the liposome and thereby decreases payload leakage [107, 108]. Additionally, direct iodination of the lipids used to form the liposome can be used to prevent internal leakage, since covalently binding iodine onto lipid constrains the contrast generating material in the bilayer [109].
Moreover by incorporating iodinated molecules into the lipid bilayer, the aqueous core can carry a separate payload including drugs for theranostics applications, another imaging agent for multimodal imaging, or more of the same agent for increased payload delivery. A study by Kweon et al. reported liposomes that were simultaneously loaded with iodinated contrast into both the core and lipid bilayer (Fig. 3a) [110]. For lipid preparation, 1,2-dimyristoyl-sn-glycero-3-phosphocholine (DMPC), cholesterol, and Lipiodol (iodinated oil) were mixed together before freeze-drying . As mentioned above, cholesterol increases the rigidity of the lipid membrane decreasing leakiness of the liposomes . These lipids prepared together were used to encapsulate iopamidol (a water soluble iodinated agent) into the core of the liposomes. This dual loading procedure produced particles about 280 nm in diameter, and a solution whose iodine concentration was 49.2 mg/ml (Fig. 3b). Inclusion of both Lipiodol and iopamidol was found to improve iodine content in the nanoparticle solution over either payload alone. Intravenous injection of the liposome produced strong contrast in the aorta after injection and was observed for up to 2.5 h (Fig. 3c). Because of the lack of PEG , high uptake was seen in the liver and spleen, resulting in attenuation values of up to 684 HU for the spleen (Fig. 3d, e). This study demonstrates the versatility of liposomes for contrast agent delivery.
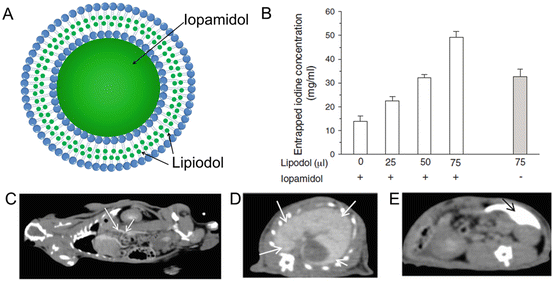
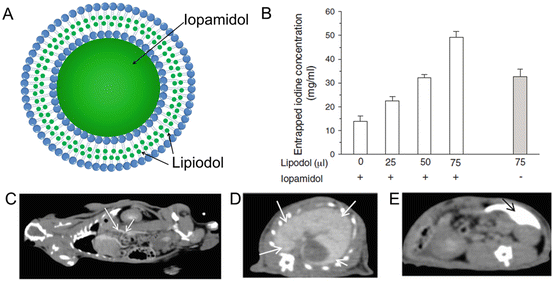
Fig. 3
(a) Schematic depiction of a liposomal formulation where Lipiodol is incorporated into the lipid bilayer and iopamidol is loaded into the core. (b) Iodine content of liposomes loaded with increasing concentrations of Lipiodol in the bilayer and with and without iopamidol in the core. The highest iodine loading was seen with inclusion of both Lipiodol and iopamidol as compared to Lipiodol alone. (c) Enhancement of aorta post-injection of Lipiodol and iopamidol containing liposomes indicated by white arrows. (d, e) Accumulation of iodinated liposomes in the (d) liver and (e) spleen. Reproduced with permission from Ref. [110]
Dendrimers are another platform that has been used to develop contrast agents for computed tomography . Dendrimers are polymeric molecules formed through sequential branched growth steps termed “generations .” Each generation exponentially increases the number of branches, isolating the core from the external environment. Additionally, the last generation of the branched sequence can be functionalized to confer specific properties to the dendrimer. The branched structure provides excellent molecular stability and can provide many sites for functionalization at the ends of the branches [111]. Dendrimers synthesized using poly(amido amine) (PAMAM) allow for functionalization through amine groups at the available termini of the molecule. By conjugating triiodobenzenepropanoic acid (DMAA-IPA) onto a PAMAM dendrimer, Yordanov et al. were able to incorporate up to 33 % iodine content by weight [112]. Large dendrimers are often quickly cleared through the mononuclear phagocytic system; however, Fu et al. reported dendrimer-based CT contrast agents with improved circulation times. These dendrimers were formed via the use of a large PEG core (6000–12,000 MW) and poly-l-lysine for branch generation. The amine termini of these dendrimers were functionalized with triiodophthalamide molecules to provide CT contrast [113].
5.2 Targeted Iodinated Agents
Nanotechnology offers several platforms upon which to develop contrast agents for CT as outlined above. Blood pool agents are useful to help visualize several pathologies in coronary artery disease and myocardial infarction, however specific targeting of contrast agents can allow single mechanisms or processes in cardiovascular disease to be probed. The progression of coronary artery disease occurs in many stages, providing numerous targets to study. Targeting can be accomplished by attaching ligands such as antibodies or peptides to the surface of the particle. Moreover, the use of native biological material such as lipoproteins (e.g., LDL, HDL) can provide targeting for cardiovascular disease .
Pan et al. utilized poly(styrene-b-acrylic acid) to produce nano-emulsions with high payloads of Lipiodol (up to 37 % wt of iodine) under 100 nm in diameter [114]. Furthermore, the authors conjugated an anti-fibrin monoclonal antibody onto the particle using a modified avidin–biotin linker. Fibrin is generated during the coagulation cascade and is a key element in thrombus formation. It is a very high density target and therefore suitable for molecular imaging via CT (high density targets are needed due to the low sensitivity of CT). A fibrin targeted agent may reveal information on plaque rupture and subsequent thrombus formation. Using a fibrin clot phantom, the agent found a CNR value of 98 ± 12 compared to the surrounding water. From rat experiments , the blood half-life of the agent was found to be 56 min, although in vivo thrombus targeting experiments were not reported.
Detecting the macrophage content within a plaque is hypothesized to be a marker of plaque stability, since increased macrophage population has been found to be characteristic of vulnerable plaques [115]. Macrophages are another high density target, due to their high populations in plaques and ability to take up very large amounts of agent. Several types of iodinated contrast agents have been developed to noninvasively image macrophages in plaques. Hyafil et al. utilized a formulation for macrophage imaging termed N1177 , which consists of ethyl-3,5-bis(acetylamino)-2,4,6-triiodobenzoate contained in an amphiphilic tri-block copolymer [116]. The emulsion was prepared through milling and further stabilized with PEG . The particles had an average diameter of 259 nm. The authors hypothesized that N1177 is taken up by macrophages in the circulation and subsequently infiltrate the plaque allowing for detection. Two hours after intravenous injection using the balloon-injury rabbit model of atherosclerosis , specific enhancement of the arterial plaque could be identified in CT with a maximum enhancement of 14 HU. Histology confirmed the localization of N1177 particles in macrophages within plaques. The same group published a follow-up study focusing on N1177 where they quantified inflammation through macrophage targeting [117].
The use of N1177 as a specific macrophage marker was used to investigate atherosclerotic plaque rupture in a study by Van Herck et al. [118]. The authors hypothesized that after plaque rupture, increased influx of macrophages to the rupture site would allow for detection of the rupture. The authors initially investigated cytotoxicity of macrophages using N1177 in vitro which they found no effect on viability or secretion of cytokines by J774 macrophages (Fig. 4a, b). In this case, the atherosclerosis model used was rabbits kept on hypercholesterolemic diets long term (12–15 months) to establish plaques. As a control, an I.V. injection of N1177 showed slight enhancement of the aortic lumen but returned to pre-scan levels within 2 h, demonstrating that N1177 did not significantly enhance non-ruptured plaques. This result was contrary to the findings of Hyafil et al. [116], but the models of atherosclerosis used in the two studies differed considerably. On the other hand, when the authors induced plaque rupture mechanically and injected N1177, the attenuation of the ruptured plaque was increased significantly (34 HU, P < 0.001) as compared to pre-ruptured plaque (Fig. 4c). Histology on the plaques showed increased staining of RAM-11 (a macrophage marker) in ruptured plaques. Additionally, N1177 was found in macrophage rich areas of the ruptured plaques but not in non-ruptured plaques using time-of-flight-static secondary ion mass spectrometry. These results demonstrate that N1177 uptake in macrophages could be used to rapidly detect and assess plaque rupture. Other groups have also focused on macrophage specific targeting. For example, Ding et al. developed iodinated oil nano-emulsions containing quantum dots for dual modal imaging of plaque macrophages with CT and fluorescence [119].
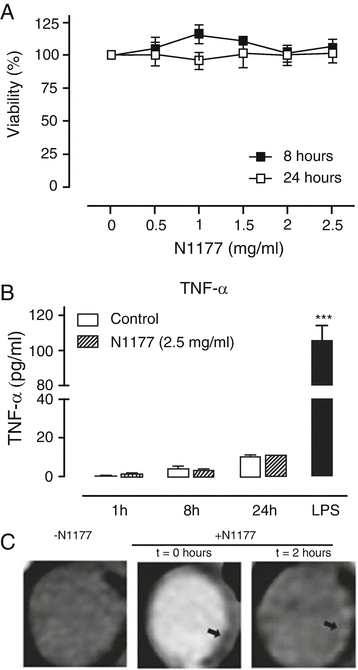
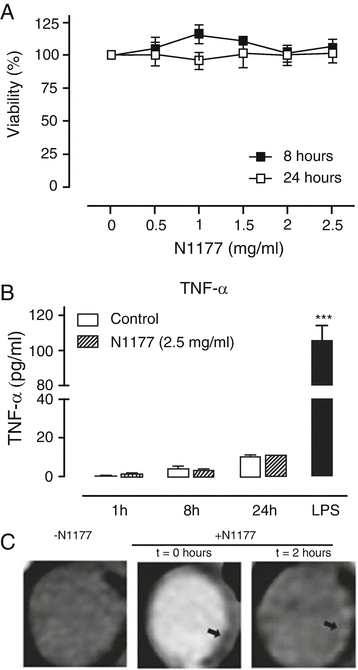
Fig. 4
(a) Viability of J774 macrophages after treatment with N177. (b) TNF-α release after treatment with N1177 . (c) CT images of rabbit aortas after mechanical plaque rupture without N1177 , immediately after injection of N1177 and 2 h post-injection. Significant increases in attenuation were seen after rupture of plaque. Reproduced with permission from Ref. [118]
Low-density lipoprotein is an attractive platform for targeted imaging due to its evasion of the immune system, biocompatibility, biodegradability, defined size and innate targeting. LDL infiltration in leaky endothelium is a key stage in early atherosclerosis development. A number of studies have attempted to use LDL as nanoparticle platform to carry imaging agents for detection of plaque for multimodalities [120–122]. For iodinated contrast agents , a study by Hill et al. demonstrated the feasibility of using LDL containing iodinated triglycerides to target liver cells (HepG2) that overexpress the LDL-receptor [123]. This in vitro study resulted in visible enhancement of the cells , but would require further testing in vivo.
5.3 Bromine Based Agents
In addition to iodinated agents, bromide containing agents have been explored as CT contrast agents for several decades [124]. The most widely used formulations are based on perfluorooctylbromide (PFOB) , which is a brominated fluorocarbon. While the CT contrast generated by bromine is relatively poor, necessitating very high doses, the excellent biocompatibility and inert nature of PFOB motivated developing CT contrast agents on this compound. These fluorocarbons are emulsified with phospholipids to produce stable nanoparticles that are capable of attenuating X-rays. Early studies demonstrated PFOB as an effective CT contrast agent for enhancement of the liver, spleen and vasculature [125, 126]. Other studies have looked at the potential of PFOB emulsions as a multimodal imaging contrast agent for ultrasound or MRI in addition to CT [127, 128]. A study by Li et al. included superparamagnetic iron nanoparticles (Fe3O4) in a PFOB emulsification to produce tri-modal contrast agent for CT, MRI, and ultrasound [129]. The magnetic-PFOB nanoparticles were approximately 200 nm in diameter and interestingly demonstrated higher echogenicity than PFOB particles alone. Additionally, contrast enhancement of the liver was seen after intravenous injection for both CT and MR imaging.
5.4 Inorganic Blood Pool Agents
While iodinated small molecules are the contrast agents used for X-ray computed tomography clinically, there has been significant preclinical interest in the development of inorganic nanoparticles as imaging agents. Inorganic nanoparticles can provide improved X-ray attenuation at CT energy ranges due to higher k-edge energy levels as compared to iodinated agents. Furthermore, the payloads of inorganic nanoparticles can be much higher than for iodine based agents, since the density of inorganic materials is often very high. For example, Brown et al. reported a solid bismuth core CT contrast agent (core density 9.8 g/ml) whose 76 nm cores carried a payload of 6 million Bi atoms each [130]. Additionally, the use of inorganic nanocrystals may alleviate biocompatibility complications associated with iodinated agents as outlined above.
Of the various types of inorganic nanocrystals that have been proposed as CT contrast agents, gold nanoparticles (AuNP) have been the focus of most work. Gold nanoparticle syntheses allow for control of size, shape, and functionality of the particles. This precise control allows for specific tailoring of these particles for a given application. AuNP can be included in various nanotechnology platforms, i.e., micelles , liposomes , dendrimers , and as ligand stabilized crystalline nanoparticles [131, 132]. Gold nanoparticles are also well known for their biocompatible properties and have been used in a variety of biomedical applications such as drug delivery, imaging, biosensors, and photothermal ablation [133–137].
As a CT contrast agent, the physical parameters of the particle as well as the chemical properties can drastically influence the particle’s biological properties in terms of clearance and biocompatibility. One of the first AuNP developed as an X-ray contrast agent was a 1.9 nm spherical formulation, and was shown to provide strong enhancement of the major vessels. However, due to the small size of the agent, the particles were rapidly cleared through the renal system as evidenced by high attenuation in the bladder in images acquired 10 min after injection [138]. Increasing the size and/or modifying the surface functionality of AuNP has been shown to improve circulation time, as found in studies where gold nanoparticles whose core size was around 10 nm. After coating with PEG , the nanoparticles had an overall size of 38 nm. These nanoparticles were not renally cleared and provided vascular contrast over a period of 12–24 h [139].
A study by Kim et al. examined the effectiveness of AuNP as blood pool contrast agents after surface modification with PEG [140]. AuNP 30 nm in diameter were synthesized through the reduction of gold chloride by sodium citrate using a modified Turkevich method [141, 142]. Ligand exchange was performed with 5000 methoxy-PEG-thiol for stabilization and to provide a long circulation half-life (Fig. 5a). Cytotoxicity of the AuNP-PEG particles were evaluated in HepG2 (epithelial liver cell line). The particles were well tolerated up to 1000 μg/ml of Au (Fig. 5b). After intravenous injection of AuNP-PEG, the agent yielded strong contrast in the heart and major arterial vessels (>100 HU). Figure 5c shows a 3D reconstruction of the heart and vasculature, derived from images acquired at 10 min post injection. A roughly 100 HU increase in attenuation in the heart and blood vessels was sustained for 4 h post-injection, attesting to their long-circulation half-life provided by the PEG coating . The attenuation of the liver and spleen was found to increase over the 24 h period, indicating the major clearance pathways for the PEG coated AuNP. Due to the uptake of the particles by Kupffer cells and hepatocytes, the authors demonstrated visualization of a hepatoma in vivo in a rat model. Figure 5d shows cross section scans 12 h post injection, with strong attenuation in the aorta (white arrow head) and delineation of the hepatoma (white arrow). Other studies have focused on different AuNP structures (e.g., nanorods [80, 143]), coatings (such as gum-arabic [144, 145]) or incorporated gold into other platforms such as dendrimers [146–149].
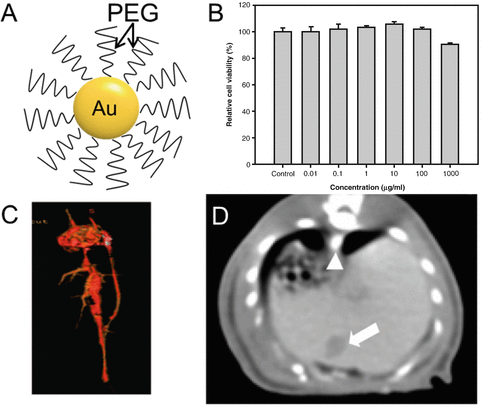
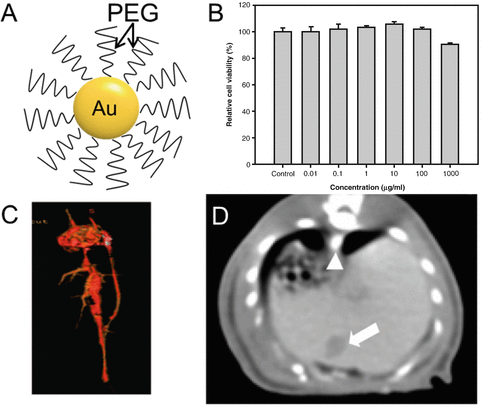
Fig. 5
(a) Schematic of PEG coated gold nanoparticles for blood pool imaging . (b) Cytotoxicity results for HepG2 cells treated with increasing concentrations of PEG coated AuNP. (c) 3D reconstruction of the heart and major vessels after injection of AuNP-PEG from CT images. (d) Cross section scans of rat hepatoma model, 12 h post injection of AuNP-PEG. Attenuation of aorta indicated by arrow head and hepatoma by white arrow. Reproduced with permission from Ref. [140]
While AuNP have desirable properties for X-ray imaging, the cost of gold may be an issue for widespread adoption and scale up. Other high atomic number elements have been explored as CT contrast agents. For example, studies have shown bismuth nanoparticles as a possible CT blood pool contrast agent [150]. Naha et al. reported the development of dextran coated bismuth-iron nanoparticles for in vivo blood contrast imaging with both CT and MRI [151].
Tantalum is another element that has been recently explored as a candidate for CT contrast agents due to the low toxicity of its oxide and strong X-ray absorption [152, 153]. A group from General Electric have published on several tantalum oxide formulations where the overall nanoparticle size is below 5 nm, thus allowing for swift renal excretion. Due to the relative natural abundance of tantalum compared to gold, tantalum is an order of magnitude cheaper, which may facilitate widespread use. In another study, tantalum oxide nanocrystals were prepared using reverse emulsions and then surface functionalized with PEG and a fluorophore tag [154]. This synthesis can be done on a bulk scale and allows the core size to be controlled in the 6–15 nm diameter range. As a blood pool agent, strong contrast enhancement of the heart and major blood vessels could be seen for up to 3 h post-injection, with eventual accumulation in the spleen and liver.
Gadolinium chelates serve as MRI contrast agents for several biomedical applications including cancer and vascular imaging [155, 156]. Despite the toxicity concerns over non-chelated gadolinium and tissue retention, studies have evaluated gadolinium as a potential CT contrast agent [157, 158]. Due to its unique properties for MRI, researchers have developed gadolinium nanoparticles for dual modal imaging with CT and MRI. The addition of gold to gadolinium chelates has shown to provide significant contrast for both modalities [73, 159, 160].
A number of other high atomic number elements have shown promise as effective CT contrast agents. Gold and silver core–shell nanoparticles have been shown to produce stronger CT contrast than iodinated agents [161, 162]. A report by Chou et al. demonstrated the use of iron/platinum nanoparticles for dual CT and MRI molecular imaging after intravenous injection [163]. Several studies have reported the use of tungsten nanoparticles as CT contrast agents that provide better enhancement compared to traditional iodinated agents [164–166]. Moreover, ytterbium based nanoparticles have received attenuation as potential CT agents due to favorable X-ray absorption spectra and relative natural abundance compared to other potential elements mentioned [167, 168]. Ytterbium based nanoparticles also present multimodal capabilities through upconversion fluorescence imaging. Upconverting fluorescent probes allow for excitation at NIR-wavelengths for deep tissue penetration with reduced background tissue absorption. Liu et al. synthesized ytterbium based particles coated in PEG for use as a CT contrast agent [169]. Xing et al. synthesized tri-modal nanoparticles for upconversion fluorescence, MR and CT imaging [170]. The group synthesized gadolinium-based upconverting nanoparticles (doped with yttrium, ytterbium, erbium, and thulium) that were also surface coated with gold nanoparticles . In vivo imaging of these trimodal particles was demonstrated with subcutaneous injection of the particles at tumor bearing locations in mice.
5.5 Targeted Inorganic CT Contrast Agents
The synthesis of inorganic nanoparticles presents an opportunity for relatively straightforward functionalization of the particle surface for targeting capabilities. Common methods for targeting involve conjugation of peptides complimentary to a specific target [171, 172]. The use of antibodies conjugated to inorganic nanoparticles to create targeted CT contrast agents has been demonstrated in several studies [173, 174]. While several methods have been developed for targeted tumor imaging with CT, targeted CT cardiovascular imaging with inorganic nanoparticles is less well explored. Chhour et al. demonstrated the use AuNP to specifically label monocytes and track their recruitment to developing atherosclerotic plaques in mice with CT [175]. The group found significantly increased attenuation in the plaques 5 days after injection of the labeled cells. Due to CT’s low sensitivity to contrast agents, a large amount of contrast generating material needs to be delivered to a site for imaging, as compared to MRI or PET.
The advent of multicolor or spectral CT has allowed different elements to be distinguished in CT images due to the characteristic X-ray attenuation profiles of the elements [176]. Spectral CT utilizes novel, photon-counting detectors to discern the energies of incident X-rays . By separating the transmitted X-rays into multiple energy bins, X-ray attenuation in the field of view can be correlated to specific elements (since the boundaries of the bins are usually set to the k-edges of the elements expected to be found in the subject). Discerning multiple material types in CT scans presents the opportunity for better delineation between contrast agent and high attenuating tissue. For instance, early investigations have involved discriminating barium/calcifications and ytterbium/bone using spectral CT [177, 178]. A study by Cormode et al. examined the composition of atherosclerotic plaques using spectral CT [179]. The purpose of the study was to examine the use of spectral CT to characterize macrophage burden, calcification and artery stenosis in a single CT scan. Gold nanoparticles incorporated into a coating similar to high density lipoprotein (Au-HDL ), 7.2 nm in overall diameter, were used to target macrophages in atherosclerotic plaques (Fig. 6a) [180]. As an initial demonstration of spectral CT, a phantom consisting of different concentrations of Au-HDL, iodine, and calcium phosphate were scanned (Fig. 6b). By using spectral CT with energy bins tuned to the k-edges of the elements involved, gold, iodine, and Ca3(PO4)2, concentration gradients could be distinguished. The photoelectric effect was used to visualize Ca3(PO4)2. For in vivo studies, Au-HDL was intravenously injected to allow macrophage targeting and after 24 h an additional vascular phase iodinated contrast agent was injected. A conventional CT scan image is seen in Fig. 6c. Comparatively, the scan with multicolor CT revealed the aorta, which had been strongly enhanced by the iodinated agent (red color), was distinguishable from Au-HDL localized in a nearby plaque (gold color) (Fig. 6c). Electron microscopy and confocal microscopy confirmed that the Au-HDL agent was targeted to macrophages within the plaque. Pan et al. studied the use a bismuth based nanoparticle conjugated to a fibrin specific antibody, which the authors termed NanoK [181]. The NanoK particles were found to have a hydrodynamic diameter between 150 and 230 nm. Anti-fibrin NanoK were in situ incubated with balloon induced thrombuses using temporary snares for 30 min. Arterial circulation was reestablished for 30 min to wash unbound particles and then the animal was euthanized. Using spectral CT, the NanoK particles could be distinguished from calcified regions at the area of induced clot injury.
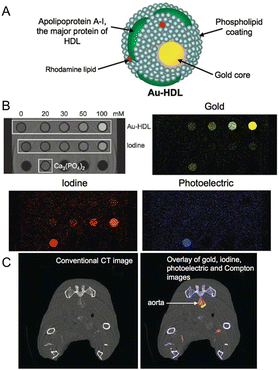
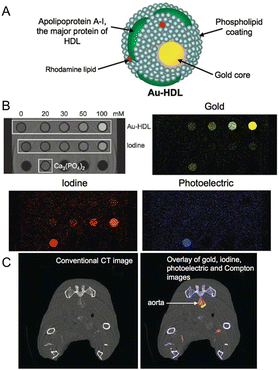
Fig. 6
(a) Schematic depiction of the structure of Au-HDL. (b) Spectral CT phantom imaging of concentration gradients of Au-HDL and an iodinated contrast agent. Ca3(PO4)2 was located in the third row of the phantom. Via materials basis decomposition of the spectral imaging data, attenuation from Au-HDL, iodine, and Ca3(PO4)2 was distinguishable. (c) Injection of Au-HDL and 24 h later injection of a vascular phase iodinated agent. Conventional CT image (left) compared to an overlay of gold, iodine, photoelectric, and Compton images from a spectral CT scan (right). Reproduced with permission from Ref. [179]
6 Conclusions
Current clinical contrast agents for X-ray computed tomography provide important diagnostic information for evaluation of cardiovascular disease but suffer from a number of drawbacks. New contrast agents are being designed utilizing nanotechnology , offering solutions to improving blood circulation time, biocompatibility, and allowing for specific targeting. Several strategies have been developed to synthesize iodinated contrast agents for blood pool imaging including nano-emulsions , liposomes , micelles , and dendrimers . Similarly, a variety of inorganic elements have been synthesized for use as CT contrast agents. Gold nanoparticles have been most frequently reported as inorganic nanoparticles for CT contrast agents, however other elements including bismuth, tantalum, and ytterbium continue to be explored. Each technology has its own advantages and weaknesses in terms of particle stability, clearance time, and X-ray attenuation properties that make each platform distinct. With a variety of platforms and formulations, nanoparticle technology will continue to improve the diagnostic capabilities of cardiac CT. Nanoparticle based agents seem to have clear advantages for blood pool imaging and we expect that the next decade will see efforts to translate nanoparticle blood pool agents to the clinic. The field of targeted CT agents for cardiovascular agents is relatively nascent, but we expect that considerable progress will be made in this area, both in terms of expanding the number of targets that can be imaged and with regards to improvement in nanoparticle technology. Advances in CT imaging technology promise to aid in these efforts. The advent of spectral (multicolor) CT allows detection of contrast media without pre-injection scans and allows identification of multiple materials in a single scan. Developments in computing and algorithms such as iterative reconstruction will increase the sensitivity of CT via suppression of image noise. Doubtless, unforeseen innovations and new technologies will arise. Overall, we expect to see the field of nanoparticle contrast agents for cardiovascular CT imaging to continue to flourish over the next decade and beyond.
Acknowledgments
This work was supported by R01 HL131557 (D.P.C.), R00 EB012165 (D.P.C.), W. W. Smith Charitable Trust (D.P.C.), and the T32 HL007954 (P.C.). We also thank the University of Pennsylvania for startup funding.
References
1.
Mozaffarian D, Benjamin EJ, Go AS, Arnett DK, Blaha MJ, Cushman M, et al. Heart disease and stroke statistics- 2015 update: a report from the American heart association. Circulation. 2015;131(4):e29–322.
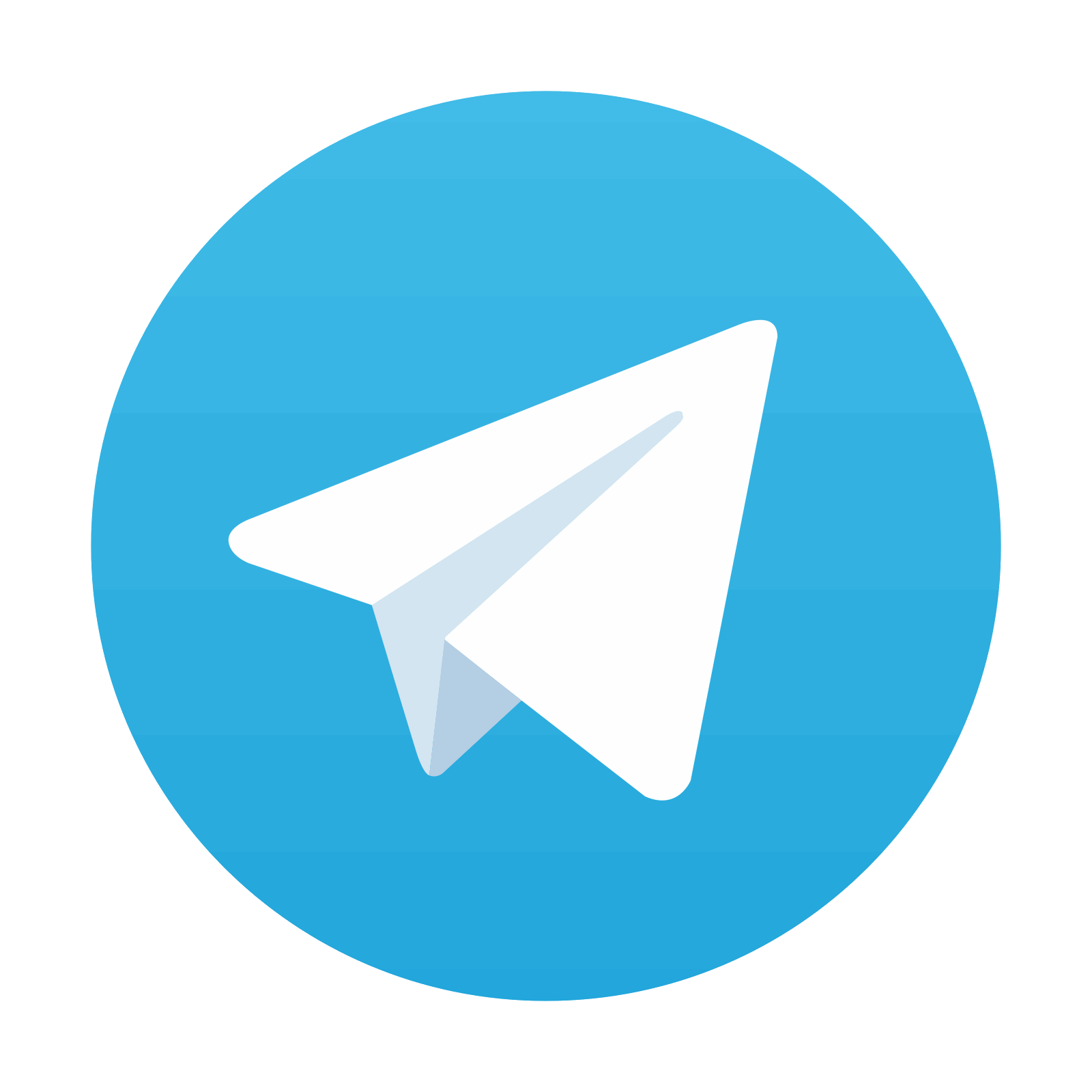
Stay updated, free articles. Join our Telegram channel

Full access? Get Clinical Tree
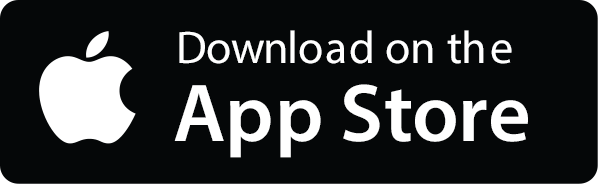
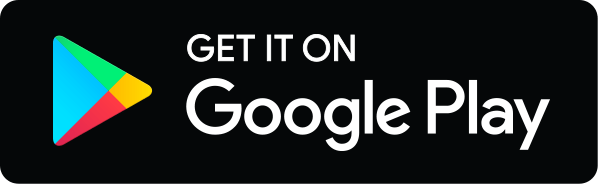