Fig. 11.1
Schematic view of the events occurring in the genesis of BOLD signal. Explanations in the text
Neuronal firing gives also rise to synaptic activity, and, during synaptic activity, neurotransmitters are released together with other substances as ADP, H+, K+, and nitric oxide (NO). All these substances, but especially NO, are powerful vasodilators [12, 13]. The mechanism at the basis of the neurovascular coupling seems to be the action of NO (possibly mediated by astrocytes) on the precapillary sphincters, where it causes smooth muscle sphincters to relax: the upstream arterial pressure gives rise to important sphincters vasodilation, with consequent huge inflow of oxygenate blood. The spatial extension of vasodilation involves a volume of 1–3 mm3 (spatial resolution), an area corresponding to the local anatomy of the terminal microvascular bed, which is similar, but non equal, to the neuronal firing area (the first is far wider). The latency (temporal resolution) of the vasodilation is of some seconds, the time necessary for NO to be released by synaptic activity, to diffuse in the interstitial space, to reach sphincters, and to transduce the signal in smooth muscle relaxation The vasodilation consequent a neuronal firing is also called “neurovascular coupling.”
Deoxyhemoglobin production and vasodilation are two phenomena not proportional: vasodilation is largely prevalent compared to the increase in oxygen utilization [14]. Consequently, the increase in blood flow is huge with respect to deoxyhemoglobin production. The local increased supply of arterial oxygenated blood dilutes the deoxyhemoglobin concentration in the capillary and venular side of the area where neural activity was increased. The dilution is so important that deoxyhemoglobin concentration reaches values well below those at rest. When we consider gradient echo images of the brain at MR high fields (T2*), such a reduction in paramagnetic deoxyhemoglobin gives rise to a clear-cut local signal increase.
At this point, we may give an answer to a previous question: why the last Ogawa and coll. deduction was not completely true? Because, in their anesthetized rats, there was no possible local vasodilation induced by any neuronal activity. As a matter of fact, when you analyze a BOLD signal in an unanesthetized subject, you have to take into account not only deoxyhemoglobin increase, consequent to neural activity, but also, and especially, arterial blood flow increase, triggered by synaptic activity.
Summing up, BOLD signal is an indirect phenomenon, not generated by neuronal spikes per se, but derived from the physicochemical and physiological consequences of neuronal firing and synaptic activity. It mirrors neuronal activity, but has far less spatial (mm instead of μm) and temporal (seconds instead of ms) resolution.
11.3 Hemodynamic Response
The hemodynamic response (HDR) describes the time course of the BOLD signal, generated by an activated cortical area and acquired by an MR EPI GE T2* sequence ( Fig. 11.2). It was described for the first time in 1995 [15] and is usually plotted as a % signal change during time. It consists in a curve starting with a small initial negativity (dip), followed by a steep rise, which reaches a peak in 2–3 s. If the stimulus is short, the signal returns to the baseline, after the peak. If the stimulus is longer, the increase is higher and continues with a plateau, preceded by an extra peak (overshoot). After the end of the stimulus, with a latency of 2–3 s, a drop occurs, which continues beyond the baseline and becomes negative (undershoot poststimulus).
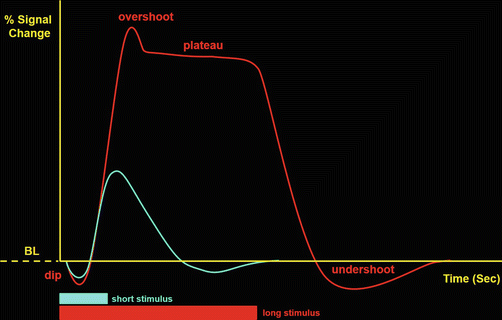
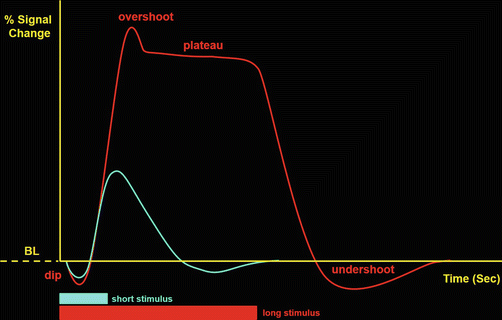
Fig. 11.2
Time course of hemodynamic response (HDR): responses appear different depending on stimulus duration. When stimulus is short (short blue bar), the curve is smaller in height and duration (blue curve); when stimulus is longer (long red bar), the curve, besides being taller and longer, shows a dip, a plateau, an overshoot, and an undershoot (red curve)
Three variables are at the basis of the described time course: (1) deoxyhemoglobin concentration (and oxygen consumption), (2) local cerebral blood flow (CBF), and (3) cerebral blood volume (CBV).
The initial dip is very small: its intensity is in the order of magnitude of 0.5–1.0 % of the whole BOLD signal (it often is not detectable in 1.5 Tesla systems). It is very spatially localized and corresponds to neuronal firing. It occurs in the gray matter only and is due to the local increase in the deoxyhemoglobin concentration. Since few seconds are needed for the local vasodilation to begin, in the early phase, the increased oxygen consumption, due to the raised neuronal firing, is not matched with the increase in CBF (hence in oxyhemoglobin) and the paramagnetic deoxyhemoglobin prevails, giving rise to a negative signal. As soon as vasodilation begins, the huge oxygenated blood flow predominates over the locally produced deoxyhemoglobin, and the curve inverts its slope, increases very quickly, and goes beyond the zero up to reach the peak just 5–8 s after the beginning of the event ( Fig. 11.3). The so-called BOLD effect is usually referred to this positive response: it is more spatially diffuse, with respect to the area of neuronal firing, because it involves capillaries and venules and has a lower spatial resolution. The local increase in CBF, besides increasing oxyhemoglobin concentration, produces an increase also in CBV, whose dynamic is slower than the other phenomena described. When the stimulation ends, neuronal firing ceases and also local vasodilation ceases, but with a latency of 2–3 s. The curve drops off toward the baseline, goes beyond it, and becomes negative (undershoot). Overshoot and undershoot are both due to the disproportion between CBV and CBF, whose dynamics are faster than that of CBV [16]. When stimulation begins, CBF increases faster than CBV, with a relative increase of oxyhemoglobin (overshoot); when stimulation ends, CBF regains baseline values faster than that of CBV, this time with a relative increase of deoxyhemoglobin (undershoot). The undershoot amplitude is often a function of the stimulus duration; usually it takes 1–2 min to regain the baseline.
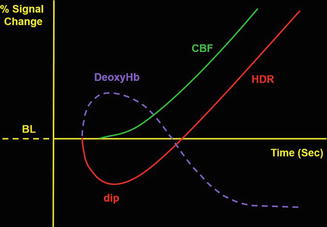
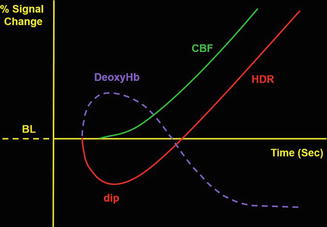
Fig. 11.3
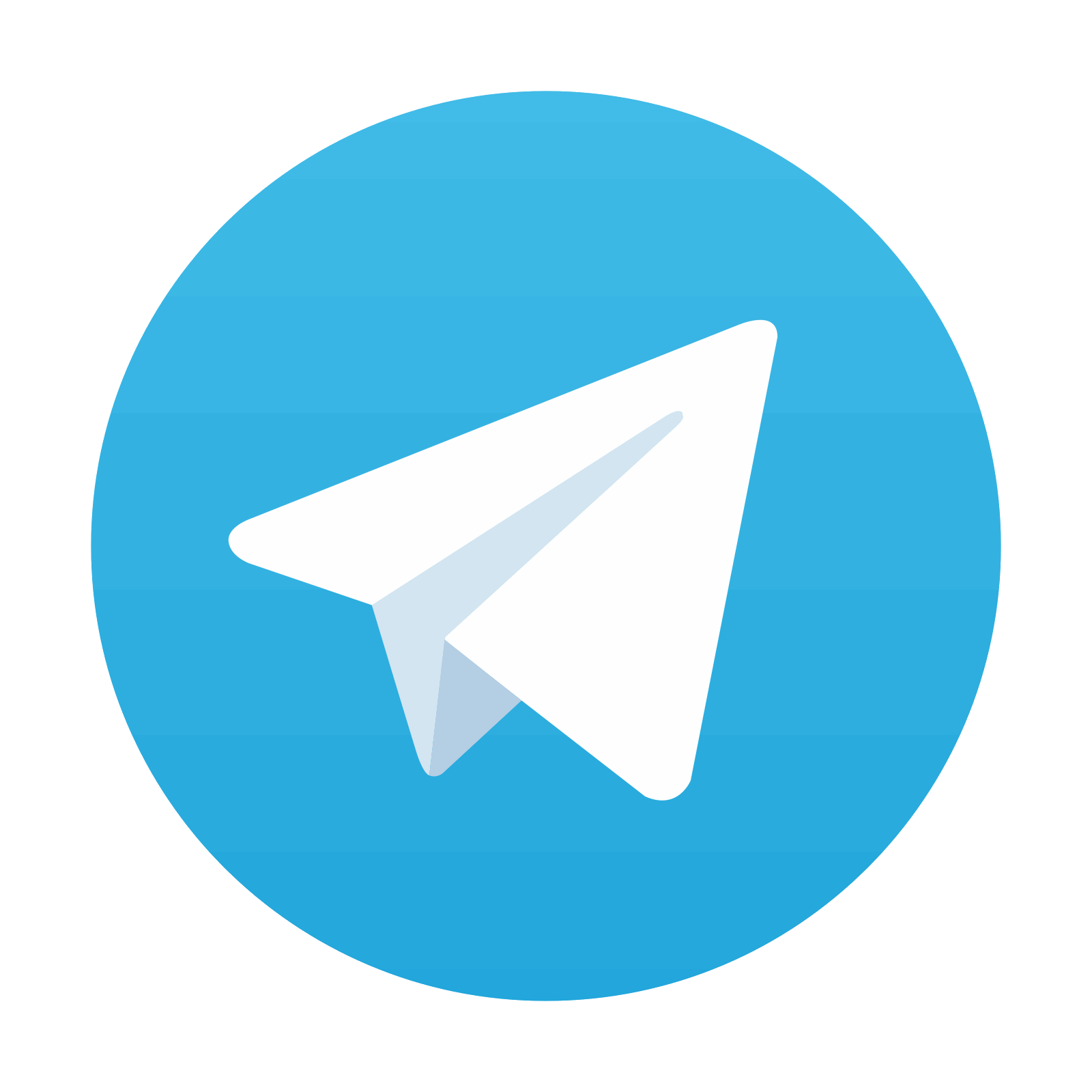
Events characterizing the dip. The initial decrease in hemodynamic response (HDR, red) is due to a mismatch between deoxyhemoglobin concentration (violet) and cerebral blood flow (CBF, green). At this initial stage, local vasodilation is still not occurred. The increased oxygen consumption, due to increased neuronal firing, gives rise to a local increase in deoxyhemoglobin concentration, with consequent signal decrease. A soon as vasodilation occurs, the big local increase in CBF (and hence in oxyhemoglobin) dilutes deoxyhemoglobin and the signal rapidly increases
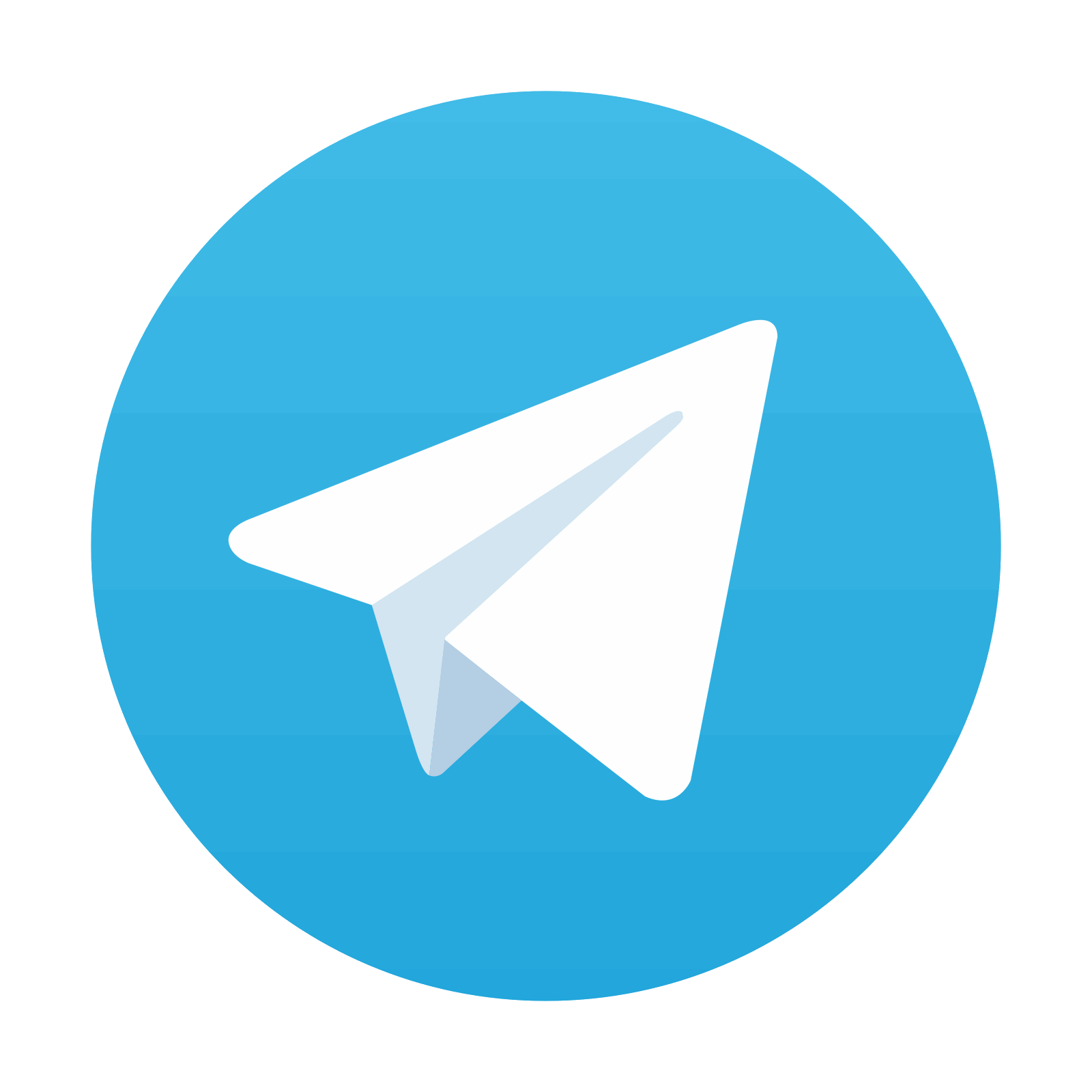
Stay updated, free articles. Join our Telegram channel

Full access? Get Clinical Tree
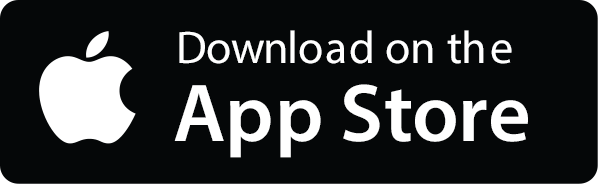
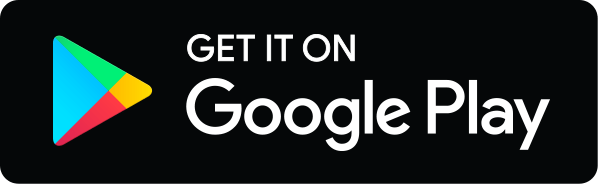
