Fig. 15.1
Upper panel: example of an early human fear-conditioning task describing anticipatory skin conductance responses (SCR) to a conditioned stimulus (CS+), in the figure at locations A and B (From Rodnick (1937)). Lower panels: activity correlated with a parametric modulation of the amplitude of anticipatory SCR to CS+ during the course of fear conditioning (decreasing slope), showing clusters of activity in the bilateral amygdala and thalamus (N = 34, pFDR < 0.025) (Reproduced by kind permission of Elsevier from Spoormaker et al. (2011))
Using fMRI, limbic and paralimbic regions such as the amygdala, hippocampus, dorsal anterior cingulate cortex (dACC), and ventromedial prefrontal cortex (vmPFC), as well as the insula and thalamus, have been repeatedly demonstrated to subserve fear conditioning and extinction in healthy individuals (for a systematic review, see Sehlmeyer et al. 2009). Already early fMRI studies on human fear conditioning revealed activity in the dACC and bilateral insula associated with CS+ (Büchel et al. 1998; LaBar et al. 1998), whereas the first human fMRI fear extinction study showed an increase in activity in the vmPFC during fear extinction (Phelps et al. 2004). In analyses addressing integration of SCR and fMRI data, the onset SCR to CS+ as described above appears specifically correlated to amygdala and thalamus activity to CS+ during fear conditioning (e.g., Spoormaker et al. 2011), see Fig. 15.1, lower panels. A quickly growing body of research has started to address the overlap in neurocognitive mechanisms underlying fear conditioning and extinction in preclinical and clinical studies, leading authoritative reviews to conclude that this is a successful model for translating data from animal models to human subjects and psychiatric patients (Milad and Quirk 2012; Pitman et al. 2012).
15.2.1 Functional Imaging of Fear Conditioning and Extinction: Relevance for PTSD
Fear conditioning and extinction has been increasingly recognized as a promising human and animal model for studying the neural circuitry involved in anxiety disorders, most notably PTSD (Rauch et al. 2006; Pape and Pare 2010). Besides forming the core of theoretical models on the etiology of normal and pathological anxiety (Lissek et al. 2005), multiple independent research groups have now provided the critical evidence for impaired extinction in PTSD and other anxiety disorders (Lissek et al. 2005; Blechert et al. 2007; Wessa and Flor 2007; Milad et al. 2009).
Crucially, the amygdala and dACC show abnormal fMRI activity in PTSD patients during extinction and at recall of extinction (Milad et al. 2009), whereas hypoactivity was observed in both the vmPFC and hippocampus. This pattern of activation and deactivation has also been reported by a recent meta-analysis of 26 functional neuroimaging studies of PTSD employing various tasks (from symptom provocation to fear extinction) that found that the dACC and amygdala were the most strongly activated regions in PTSD, whereas the vmPFC and the inferior frontal gyri were the most strongly deactivated regions in PTSD (Hayes et al. 2012). Moreover, a decrease in vmPFC activity was associated with increased amygdala activity (Hayes et al. 2012), in line with the inhibitory connections from the vmPFC to amygdala (LeDoux 2000). Neurocircuitry models of PTSD postulate that this failure of the vmPFC and hippocampus to downregulate a hyperactive amygdala is the core feature characterizing PTSD, which is postulated to be responsible for increased fear responses, impaired fear extinction learning and retrieval, and impairments in general emotion regulation and attentional biases towards threat (Rauch et al. 2006). In a recent comprehensive review on biological studies of PTSD (Pitman et al. 2012), a main conclusion was that the amygdala was hyperactive in PTSD in response to trauma-related stimuli as well as generic threat stimuli, and during fear conditioning, compared to control subjects. Here it is important to note, however, that amygdala deactivation has been observed in PTSD as well (Etkin and Wager 2007). Such amygdala deactivation has been proposed to be characteristic of dissociative subtype of PTSD, with limbic overmodulation rather than undermodulation as a core feature (Lanius et al. 2010a), which results in increased frontal cortical activity and decreased amygdala activity to traumatic script challenge. Patients responding with this pattern are typically characterized by early trauma histories (Lanius et al. 2010b). This would be an interesting area for further research on the use of neuroimaging tools in PTSD patient stratification.
The dACC showed increased activity during fear conditioning and recall of extinction in PTSD compared to trauma-exposed healthy controls; the vmPFC, in contrast, was concluded to have decreased activity during both trauma-related and trauma-unrelated affective stimuli. Hippocampus involvement may be task dependent and is likely related to “deficits in recognizing safe contexts” (Pitman et al. 2012). Context dependencies of CS+ (e.g., fear conditioning in context A and fear extinction in context B, manipulated by presenting different background colors during both phases) involve the hippocampus (Milad et al. 2007) and are impaired in PTSD patients compared to trauma-exposed controls (Milad et al. 2009). The hippocampus is involved at a more basic level in pattern completion or separation to CS+, which would activate the vmPFC in turn (Lissek 2012). The emotional circuitry sensitive to PTSD extends to other regions involved in affective processing, such as the insula, but this has also been observed in other anxiety disorders and may not be specific to PTSD (Etkin and Wager 2007).
15.2.2 Comparison of PTSD with Other Anxiety Disorders
Which brain activity is specific to PTSD? This question was addressed by Etkin and Wager (2007) in a meta-analysis of imaging studies that compares PTSD with specific phobias, social anxiety disorder, and fear conditioning in healthy subjects. Most functional imaging (positron emission tomography, PET; and functional magnetic resonance imaging, fMRI) studies included in the meta-analysis used symptom provocation: i.e., 10 out of 15 imaging studies on PTSD used visual- or script-based trauma cues, five out of eight imaging studies on social phobia used negative facial expressions (the other addressing public speaking and speech anticipation), and six out of seven imaging studies on specific phobias used images, words, or videos of the phobic object/animal/situation.
Results revealed that all disorders (patients > control subjects) showed hyperactivity in the amygdala and insula and that this pattern of activity was analogous to fear conditioning in healthy subjects. This suggests overlapping fear circuitry involved in both symptom provocation in patients and fear conditioning in controls, relevant across disorders. However, only PTSD further showed hypoactivity in the ventro- and dorsomedial prefrontal cortex (dmPFC), anterior hippocampus, and parahippocampal gyrus, among others, that was specific to this disorder. According to the meta-analysis, results of social and specific phobias were strongly overlapping, whereas the pattern of activation and deactivation in PTSD showed more deviation and complexity. In PTSD, hypoactivity in medial prefrontal regions tended to co-occur, whereas a pattern of hypoactivity in the medial prefrontal and hyperactivity in the amygdala was also observed in this meta-analysis. Interestingly, hyperactivity in the amygdala tended to occur more frequently in phobias than in PTSD (Etkin and Wager 2007), with potential relevance for PTSD subtype stratification (Lanius et al. 2010a, b) as mentioned above.
Due to this specificity, hypoactivity in medial prefrontal and hippocampal areas in PTSD may be of special interest. A logical prediction is that hypoactivity in these regions is correlated with extinction and/or generalization impairments. Future research has yet to address this question; however, studies have already shown that mPFC activity correlates with subjectively reported PTSD symptom severity (Shin et al. 2005). Intriguingly and consistent with this, symptom severity improvements after cognitive-behavioral therapy for PTSD have been found to be related to an increase in mPFC and a decrease in amygdala activity (Felmingham et al. 2007). Although the hypoactivity of the vmPFC and hyperactivity of the amygdala in PTSD are unequivocal, there is some lack of clarity regarding whether or not the dmPFC (dACC) is hyper- or hypoactivated in PTSD: Etkin and Wager (2007) found clear hypoactivity in PTSD, while other studies have shown hyperactivity of the dACC during fear conditioning in PTSD (Milad et al. 2009) and positive correlations between dACC and amygdala activity (Pitman et al. 2012). It is possible though that hyperactivity is confined to the more posterior dACC (middle cingulate cortex) that extends into the supplementary motor area, which has been found to consistently activate during fear conditioning (Sehlmeyer et al. 2009). More rostral portions of the dACC and ACC extending into the ventral parts may then be more hypoactivated in PTSD and involved in extinction learning and recall (Phelps et al. 2004; Etkin and Wager 2007; Milad et al. 2009). For a brief summary, see Fig. 15.2.
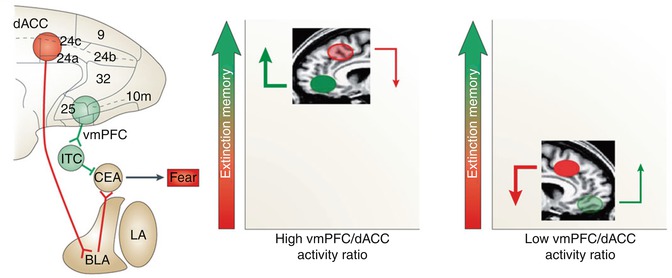
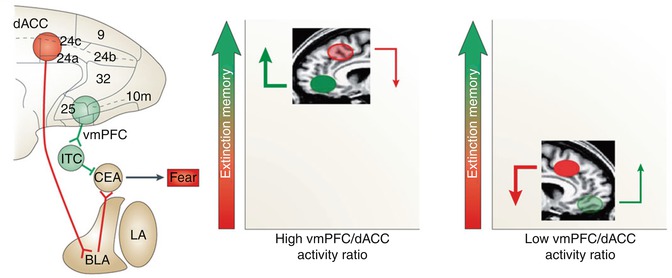
Fig. 15.2
Functional interactions among fear circuitry regions. A recent review on the biology of PTSD described the relevance of dACC excitatory connections to the basolateral amygdala (BLA), vmPFC inhibitory connections to the central nucleus of the amygdala (CEA) through intercalated cells (ITC), and a high vmPFC/dACC activity ratio for successful fear memory encoding and retrieval (left). As compared to stronger inhibitory influence of the vmPFC and lower excitatory input of the dACC over the amygdala under healthy conditions (middle), excess dACC activity during fear conditioning and extinction results in hyperactivity in the amygdala and reduced vmPFC activity (right), which further implies a failure to downregulate the amygdala, causing functional impairments in extinction memory encoding and/or retrieval (Reproduced by kind permission of Macmillan Publishers from Pitman et al. (2012))
15.2.3 Fear Conditioning: Functional and Imaging Limitations
Although fear conditioning and extinction constitutes one of the most robust paradigms in experimental psychophysiology, allowing disturbing symptom provocation tasks to be replaced by less stressful and better controlled experimental stimuli, the task has too received critique for exclusively focusing on physiological (and subjective) components of emotion while not addressing the behavioral component of (pathological) anxiety, i.e., avoidance and safety behavior (Beckers et al. 2013). New tasks combining fear conditioning with exploration and avoidance behavior in virtual reality environments are a promising start into this understudied dimension (Grillon et al. 2006). Moreover, PTSD is primarily characterized by distressing intrusive memories in the form of flashbacks and nightmares, and electrical shocks as US do not tap into (dys)function of such memory aspects. Tasks exploiting conditioning with highly intrusive movie scenes as US (Wegerer et al. 2013) may provide a promising and clinically useful extension of the typical fear-conditioning task. Furthermore, stimuli in fear-conditioning tasks are typically visual or auditory; more research attention could be devoted to alternative stimuli given the promise of olfactory trauma reminders in neuroimaging studies of PTSD (Vermetten et al. 2007).
It is possibly one of the great paradoxes in functional MR imaging of anxiety disorders that the brain regions that are most relevant, such as the amygdala, vmPFC, subgenual ACC, and orbitofrontal cortex, are among the regions that are most strongly affected by magnetic susceptibility-induced artifacts and consequently signal loss (Schwarzbauer et al. 2010). Such artifacts are caused by inhomogeneities of the magnetic field and arise especially at and in proximity of brain/air borders, due to the anatomy itself. fMRI is especially sensitive to local variations of field homogeneity, as the primary source of the image contrast is its sensitivity to small changes in the magnetic properties of hemoglobin, depending on the blood oxygenation (leading to the so-called blood oxygen level dependent – BOLD – effect, which is reflected by changes of the T2* relaxation rates of neighboring nuclear spins). As a result, fMRI image acquisition optimization is desirable to recover signal from these essential regions.
15.2.4 Panic Disorder
Panic disorder is arguably an anxiety disorder most closely related to the specific phobias and PTSD since in panic disorder, patients have learned to fear upcoming panic attacks (US) often triggered by interoceptive stimuli (CS+) thought to prequel a following attack. Agoraphobia, a fear of open or public spaces, is a frequent comorbid disorder in panic disorder. Psychophysiological research has shown impairments in extinction learning (Michael et al. 2007) and overgeneralization in panic disorder patients to various deviating versions of CS+ (Lissek et al. 2010). No imaging data have yet been published on these tasks; however, clear predictions of neural correlates of overgeneralization would point to the hippocampus given its role in pattern completion versus separation and the mPFC in relation to impaired extinction learning (Lissek 2012). Interestingly, an initial study showed increased midbrain activity in panic patients during fear conditioning (Lueken et al. 2013), which was paralleled by impaired differential learning in panic disorder patients (manifest in subjective ratings); however, no psychophysiological measures were recorded. Another fear conditioning study without psychophysiological measures also observed increased midbrain activity in panic disorder patients in response to safety cues, coactivating with clusters of activity in the ventral striatum, the anterior medial temporal lobe, and the subgenual ACC (Tuescher et al. 2011).
In general, it is of note that panic disorder has been studied to a lesser degree than PTSD, and the imaging studies that have been conducted to date, employing general affective tasks (e.g., emotional stroop, facial recognition, negative pictures), have shown mixed results (see Dresler et al. 2013, for a review). fMRI studies of affective tasks (N = 9) were inconclusive, but abnormal activity in the ACC (either increased or decreased) occurred across tasks (Dresler et al. 2013). Hyperactivity of the amygdala has not been consistently found during these tasks; however, a few case studies of spontaneous panic attacks during functional imaging sessions reported increased amygdala and insula activity (Dresler et al. 2013). Another review has implicated the amygdala, insula, mPFC and hippocampus in panic disorder (de Carvalho et al. 2010); yet as no meta-analyses have yet been conducted, a formal statistical evaluation of such potentially relevant effects is precluded.
For panic disorder, promising alternative models have been tested that may eventually be combined with fear conditioning to achieve a comprehensive view on this disorder. Hyper- and hypocapnia (elevated and reduced CO2 levels in the blood) have been experimentally induced in panic disorder (Martinez et al. 1998). This resulted in differences in heart rate and blood pressure between panic disorder patients and controls (Martinez et al. 1998), the correlates of which in fMRI need to be more closely examined. Pharmacological models of panic attacks have also been reported, such as with panicogenic neuropeptide cholecystokinin tetrapeptide (CCK-4), showing increased amygdala activation compared to placebo after CCK-4 administration in healthy subjects, as well as in the vmPFC, lateral prefrontal regions, brainstem, and cerebellum, among others (Eser et al. 2009). The reported anticipatory anxiety associated with the dACC in this study is also worth mentioning. CCK-4 led to an increase in subjective panic symptoms and heart rate, which may interfere with the experimental readout in fMRI studies – also here closer examination of potential interference of autonomic responses to panicogenic stimuli with the BOLD signal appears of interest, although the specific, localized increase in limbic and paralimbic areas after CKK-4 seems to go beyond potential unspecific baseline differences.
One methodological issue that is relevant for panic disorder but also for other anxiety disorders is that anxiety typically correlates with respiratory alterations that may produce significant changes in cerebral blood flow independent of task-related neural activation (Giardino et al. 2007). Measuring arterial carbon dioxide tension (or at the very least, breathing patterns) may be a useful way to control for variance in fMRI data of nonneuronal origin.
15.2.5 Specific Phobias, Social Anxiety Disorder, and Generalized Anxiety Disorder
A recent meta-analysis on specific phobias has confirmed the initial conclusions by Etkin and Wager (2007) and reported that phobic stimuli were associated with increased activity in the amygdala, insula, and pallidum and that these regions and the cerebellum and thalamus were also more activated in phobic patients relative to healthy controls in response to phobic stimuli (Ipser et al. 2013). Differences between patients and controls in the ACC were related to general affective processing. Noteworthy is that this meta-analysis also observed reduced activity in the dACC, thalamus, insula, and more lateral prefrontal regions after cognitive-behavioral treatment (N = 3) (Ipser et al. 2013). The same group performed a meta-analysis on social anxiety disorder as a distinct and epidemiologically relevant anxiety disorder, which also revealed increased activity in the amygdala, pallidum, and ACC in response to social stimuli (e.g., faces, linguistic stimuli), as well as in the hippocampal complex (perirhinal cortex). These conclusions are still tentative as the amount of included fMRI studies was rather small (N = 7); however, the reported regions fit well with the regions activated in fear-conditioning tasks (Sehlmeyer et al. 2009), and the absence of hypoactivity in the mPFC could have functional relevance for the specificity of the phobic fear (Lissek 2012) versus the overgeneralization in, for instance, PTSD.
Generalized anxiety disorder, an anxiety disorder characterized by excessive and diffuse anxiety and worrying rather than specific fear, has shown less consistent neural correlates than the fear-related anxiety disorders. An increased amygdala response to all – aversive and neutral – stimuli has been observed in generalized anxiety disorder (Nitschke et al. 2009), although another study reported decreased amygdala activity in response to fearful faces in patients compared to healthy volunteers (Blair et al. 2008), and further studies did not observe any group differences between patients and controls during affective stimulus processing (Whalen et al. 2008; Etkin et al. 2010). It may be that diffuse anxiety may be associated with different neural correlates than amygdala-mediated fear and a role for the involvement of the bed nucleus of the stria terminalis has been proposed (Davis 1998) and observed in generalized anxiety disorder patients in response to uncertainty (Yassa et al. 2012). Moreover, a few studies point to abnormal patterns of (de)activation in the dorsomedial and dorsolateral prefrontal cortices associated with emotional (dys)regulation (Blair et al. 2012; Ball et al. 2012), which differed from healthy controls but not from patients with generalized social phobia (Blair et al. 2012). Further research is necessary to replicate these promising observations.
15.3 Obsessive-Compulsive Disorder
The situation is different in obsessive-compulsive disorder (OCD), which has received much attention in neuroimaging and is rather discrepant from the other anxiety disorders, both in symptomatology and imaging findings. OCD is characterized by obsessive thoughts and images, with which persons cope by means of ritualistic and stereotyped overt or covert behavior. The disorder has been removed from the section of anxiety disorders in the fifth version of the diagnostic and statistical manual of mental disorders (DSM-5) and now has a separate chapter: Obsessive-Compulsive and Related Disorders. This also makes sense from an imaging perspective: instead of dysfunctional neural circuitry primarily in limbic and medial prefrontal areas, both anatomical (Radua and Mataix-Cols 2009; Peng et al. 2012) and functional (Menzies et al. 2008; Rotge et al. 2008) meta-analyses have implicated the striatum and particularly cortico-striato-thalamic loops in OCD, with additional differential activity in the orbito- and prefrontal cortices (Menzies et al. 2008; Rotge et al. 2008). Changes in striatal volumes have not always been found (Rotge et al. 2009), although heterogeneity in the meta-analyses may be due to methodological differences (Ferreira and Busatto 2010).
The meta-analysis by Menzies et al. (2008
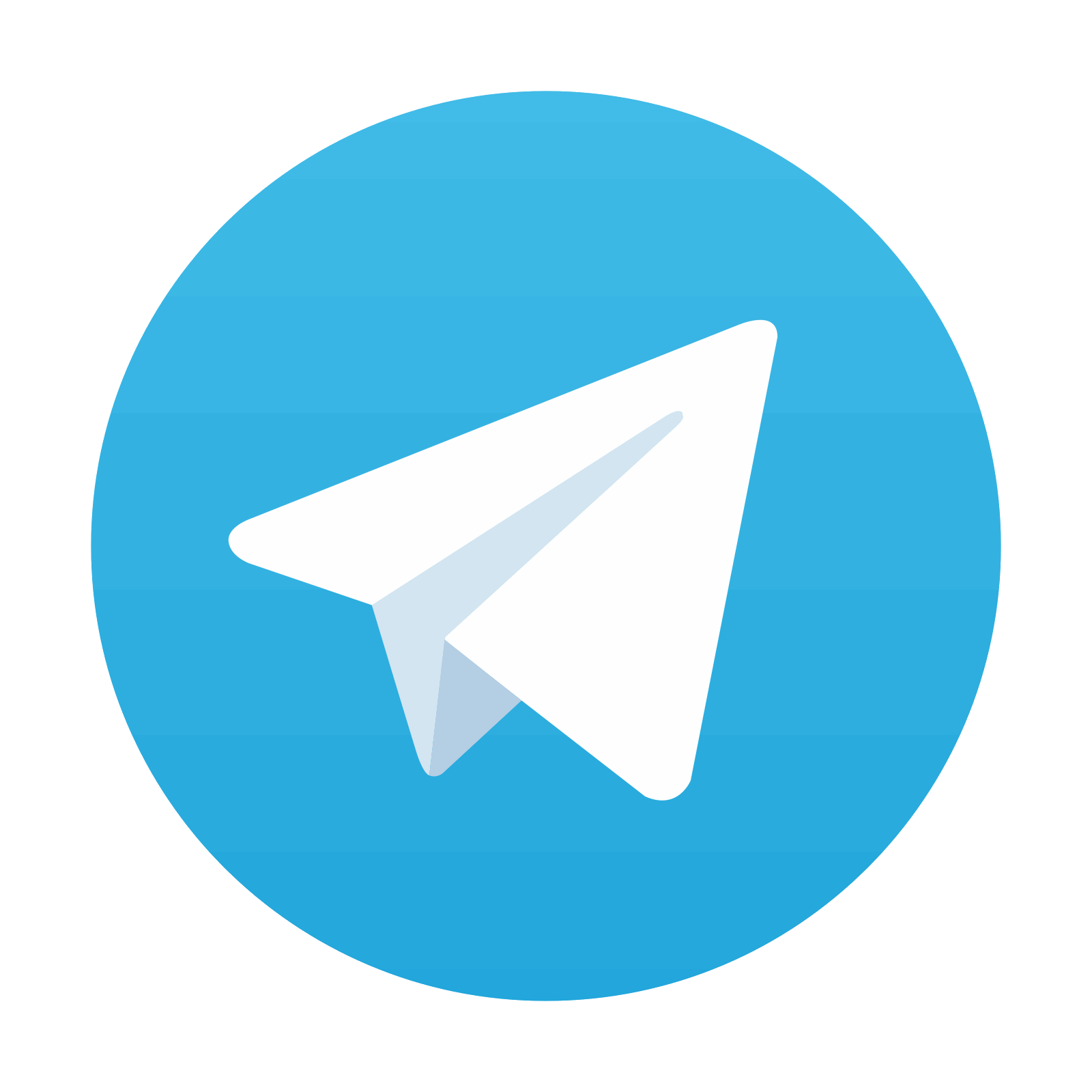
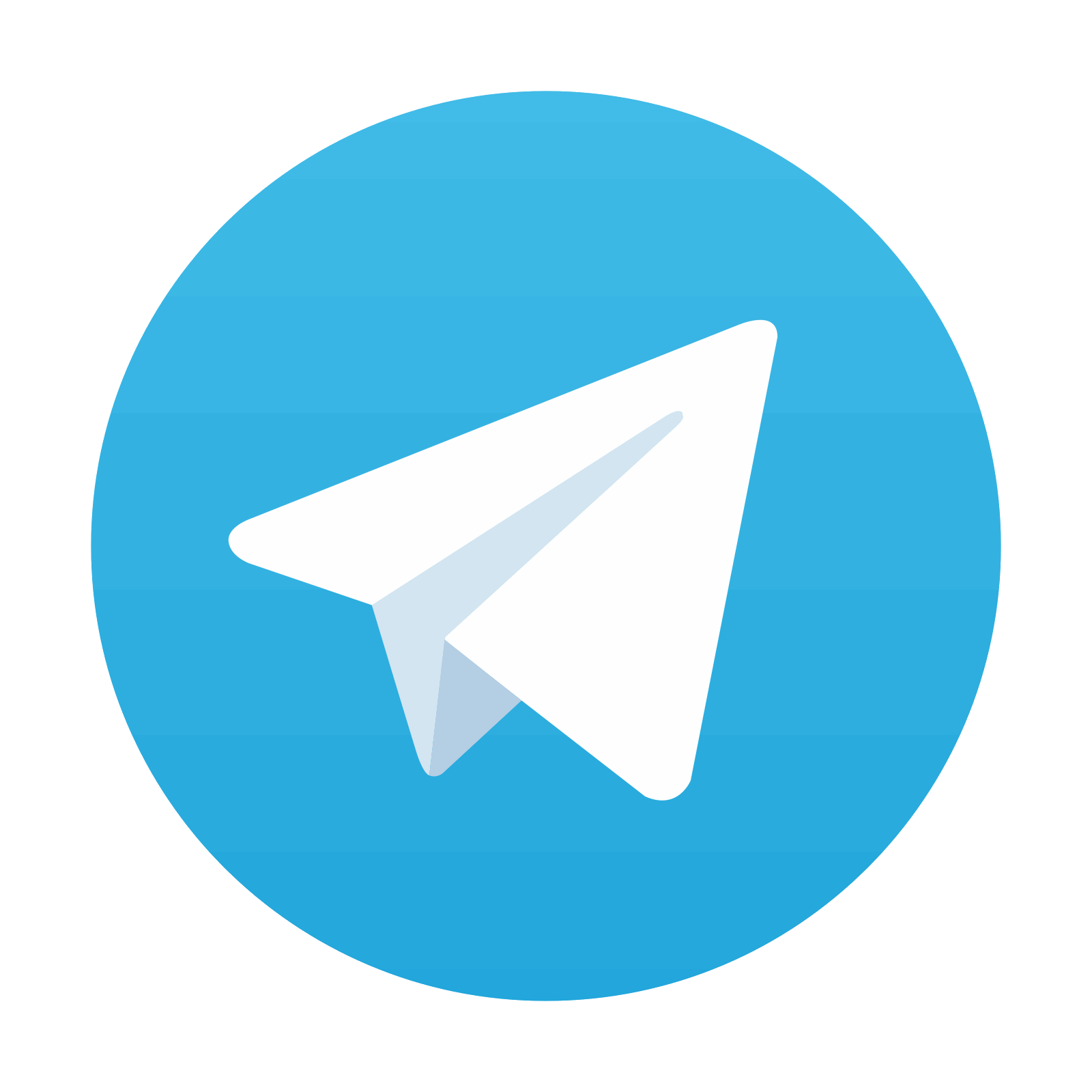
Stay updated, free articles. Join our Telegram channel

Full access? Get Clinical Tree
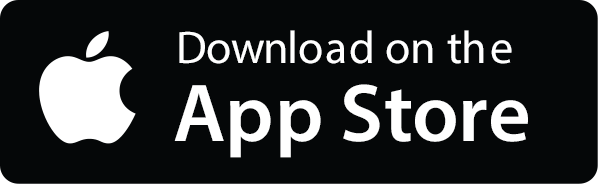
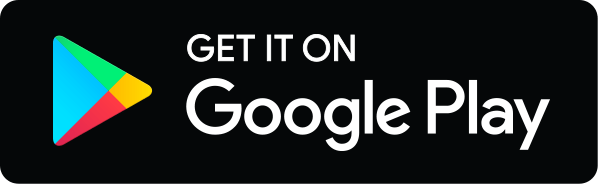